Understanding Photostability: Key Principles and Applications
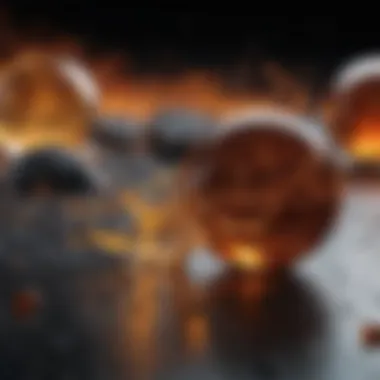
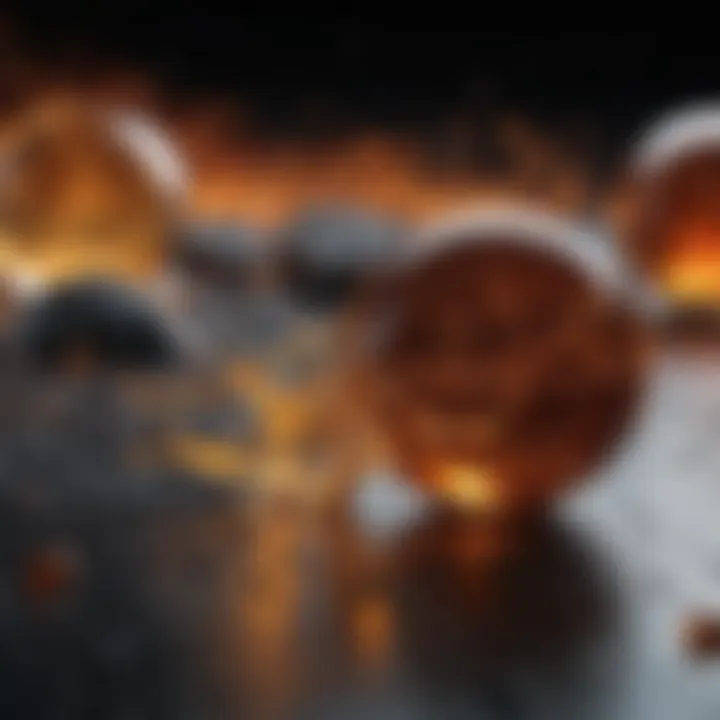
Intro
Photostability is an essential topic in material science, pharmaceuticals, and environmental research. The way materials react to light exposure can significantly impact their performance and longevity. Understanding photostability involves grasping its underlying principles, the multitude of factors that influence it, and the applications that arise from it. The complexity of light interactions with materials necessitates a careful examination of mechanisms, measurement approaches, and potential challenges.
This article aims to provide a comprehensive exploration of photostability. We will detail the fundamental concepts and significant findings, laying a strong foundation for the reader. Furthermore, the implications of photostability in various disciplines will be discussed, aiming to equip students, researchers, educators, and professionals with crucial insights.
Our approach involves a breakdown of key findings and methodologies that underpin this research area, while addressing the ongoing challenges and future directions in photostability studies. By the end, we hope to equip our readers with thorough knowledge and appreciation of the importance of photostability.
Prologue to Photostability
Photostability plays a vital role in the performance of materials exposed to light. This section elucidates its significance, particularly in understanding how various substances react when subjected to radiation. The knowledge of photostability not only informs material selection in scientific applications but also shapes development in industries like pharmaceuticals and environmental sciences.
Definition of Photostability
Photostability refers to the ability of a material to maintain its chemical and physical properties when exposed to light over time. This characteristic is crucial for many applications. For instance, in pharmaceuticals, the stability of drug compounds under light exposure can determine effective treatment durations. In environmental contexts, understanding the photostability of pollutants can significantly impact remediation efforts.
Historical Context and Evolution
The study of photostability has developed alongside advancements in chemistry and materials science. Early investigations primarily focused on how sunlight affects natural substances. Over decades, the scope has expanded, integrating modern techniques and technologies. Key milestones include the introduction of sophisticated spectroscopic methods and the establishment of standards in both material testing and product formulation. As environmental concerns increased, especially regarding man-made pollutants, research has evolved to address these contemporary challenges.
Fundamental Principles
Understanding the fundamental principles of photostability is crucial for its applications across various fields such as material science, pharmaceuticals, and environmental studies. It sets the framework for understanding how materials behave when exposed to light. The principal mechanisms that govern photostability allow researchers to predict performance, enhance formulations, and develop innovative materials designed to withstand light exposure. The implications of mastering these principles can lead to significant advancements in technology and improve product longevity.
Mechanisms of Photostability
Photochemical reactions
Photochemical reactions involve the transformation of molecules due to light absorption. When a substance absorbs light, it can undergo chemical changes that might impact its stability. The key characteristic of these reactions is their reliance on photon interaction. This makes photochemical reactions an essential focus in photostability discussions.
The unique feature of photochemical reactions is their ability to produce reactive species, such as free radicals. While this can lead to enhanced processes like polymerization, it also poses challenges. For instance, these reactive species can cause degradation of valuable materials. Hence, understanding their dynamics is essential in creating stable formulations.
Non-photochemical processes
Non-photochemical processes refer to chemical changes that occur in the absence of light. These processes might seem less relevant in photostability discussions, however, they play a critical role. The stability of materials can depend as much on non-light conditions as on light exposure itself. A key characteristic of these processes is their ability to alter physical properties without light.
A significant advantage of non-photochemical processes is that they can contribute to stability over time, particularly in dark environments. However, the challenge lies in identifying how these processes interact with photochemical changes. The effects can be complex, requiring in-depth understanding for material formulation.
Energy transfer mechanisms
Energy transfer mechanisms involve the migration of energy between different molecules. This is crucial for understanding how light energy affects stability. By transferring energy efficiently, some systems can mitigate potential damage from light exposure. This aspect becomes particularly beneficial in creating stable products that endure light.
The key characteristic is the ability to control energy flow within a material. This can enhance the overall photostability, leading to better performance and longevity of materials. However, the challenge lies in optimizing these mechanisms for practical use. Not all materials can benefit equally, and understanding each system's characteristics is essential for effective application.
Types of Photostability
Intrinsic photostability
Intrinsic photostability refers to a material's inherent ability to resist light-induced degradation without any external modifiers. The unique feature is its reliance on the material's intrinsic properties, such as molecular structure and chemical composition. Its main benefit lies in creating materials that do not require special formulations or additives to achieve stability. This can lead to more straightforward manufacturing processes.
However, the disadvantage is its limited applicability. Not all materials possess strong intrinsic photostability. For certain applications, additional stabilization techniques may be necessary.
Extrinsic photostability
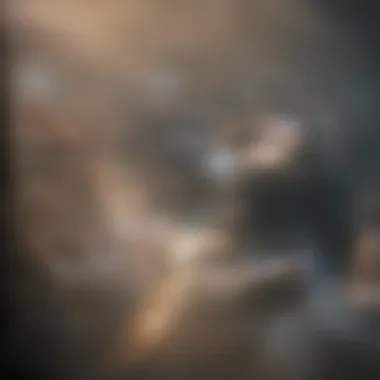
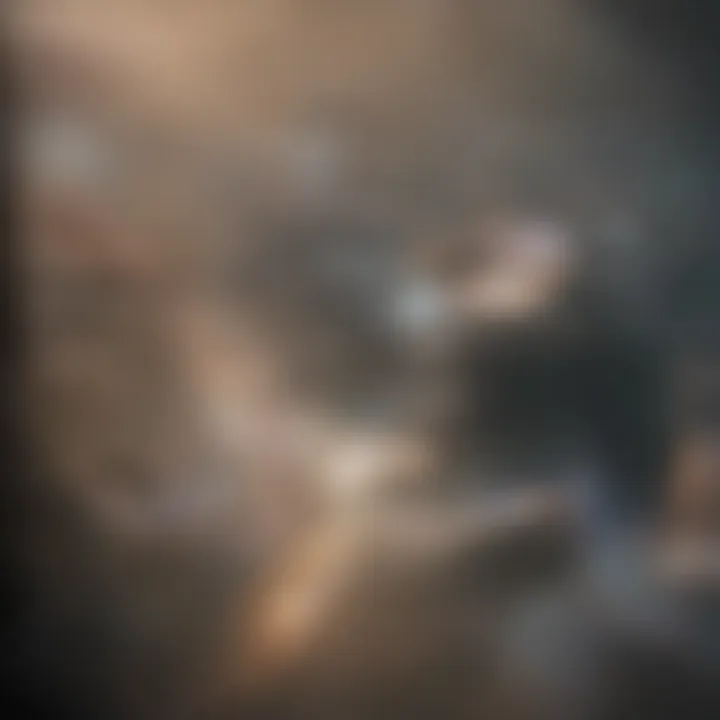
Extrinsic photostability involves the use of additives and formulations to enhance a material's resistance to light exposure. This can include the addition of stabilizers or coatings to absorb harmful energies. A key characteristic of extrinsic photostability is its adaptability, allowing for a wide range of materials to be tailored for specific applications.
The unique feature of this approach is the ability to optimize material performance for various environments. However, the challenge lies in ensuring that these additives do not introduce new degradation pathways or affect other essential properties negatively. Understanding both extrinsic and intrinsic factors is vital for creating robust materials.
Factors Influencing Photostability
Photostability plays a crucial role in various fields, impacting both the functionality and longevity of materials exposed to light. Understanding the factors that influence photostability helps researchers and professionals develop more effective materials and applications. The elements that affect photostability can be categorized into chemical composition, environmental conditions, and physical properties. Each of these factors contributes uniquely to how materials respond to light exposure.
Chemical Composition
The chemical makeup of a material heavily influences its photostability. Compounds with different molecular structures can exhibit varying resistance to light-induced degradation. For instance, the presence of certain functional groups can enhance stability by absorbing harmful wavelengths of light or by stabilizing the excited states that might lead to degradation. The choice of materials based on their chemical composition is fundamental. Materials such as UV stabilizers can be incorporated in polymers to improve their photostability, making them suitable for outdoor applications where they will experience prolonged light exposure.
Environmental Conditions
Temperature effects
Temperature significantly affects photostability. Higher temperatures can accelerate the rates of chemical reactions, including degradation processes when materials are exposed to light. Generally, elevated temperatures can lead to increased photodegradation, especially in organic compounds. This characteristic makes temperature a critical factor in studies of photostability. Researchers often conduct experiments at various temperatures to observe the behavior of materials under different thermal conditions. The unique feature of temperature effects is that it is controllable in experimental setups, allowing precise assessment of photostability. However, this can also pose challenges, as conditions must mimic real-world scenarios for accurate results.
Humidity influences
Humidity also plays an essential role in shaping photostability outcomes. Increased moisture can either stabilize or destabilize certain compounds when exposed to light. For example, some materials might absorb water, which could lead to hydrolysis and subsequent degradation. Others, however, might benefit from the presence of humidity, as it can create an environment that inhibits harmful photochemical reactions. The dynamic nature of humidity makes it a relevant topic for this article. One advantage is that it reflects real environmental conditions, aiding in developing practical solutions for material applications. The challenge lies in trying to predict its exact effects due to variability in environmental conditions.
Presence of oxygen
Oxygen is another critical factor influencing photostability. Many photodegradation processes are oxidative in nature, and the presence of oxygen can exacerbate these reactions. For example, when materials are exposed to light in the presence of oxygen, radical species can form, leading to faster degradation rates. This aspect makes the study of oxygen's role in photostability an essential focus. Understanding how materials behave in oxygen-rich environments can help in designing better stabilizers and protective coatings that minimize degradation. However, this introduces complexities, as not all materials interact with oxygen in the same way.
Physical Properties
Surface morphology
The surface morphology of materials affects how they interact with light. Materials with rough surfaces can scatter light differently compared to smoother ones, which can impact photostability. For instance, increased surface roughness might enhance light scattering, potentially reducing degradation in some cases. This characteristic of surface morphology makes it an intriguing subject in studying photostability. Choosing materials with optimal surface structures can lead to enhanced performance in photostability applications. However, balancing surface changes with other material properties can be challenging.
Particle size
Particle size is also a significant consideration in photostability. Smaller particles often have a larger surface area to volume ratio, impacting their interaction with light. This can lead to faster degradation due to increased exposure to photons. Additionally, the dispersal of nanoparticles can affect the overall stability of composite materials. Considering particle size is beneficial for optimizing photostability, especially in nanocomposites used in various industries. The trade-off includes evaluating how size influences not only photostability but also the material's other properties.
Understanding these factors enables better predictions and management of material behavior under light exposure. A comprehensive approach that considers chemical composition, environmental conditions, and physical properties is vital for advancing research in this field.
Measurement Techniques
The measurement of photostability is crucial for understanding how materials behave under light exposure. It allows researchers to quantify the stability of various substances, providing a solid foundation for applications in diverse fields such as material science, pharmaceuticals, and environmental studies. Accurate measurement techniques are essential to assess the durability of materials and optimize their formulations effectively. Key aspects include precise methods that can identify the degradation mechanisms and offer valuable data on performance reliability.
Spectroscopy Methods
UV-Vis Spectroscopy
UV-Vis spectroscopy is a widely used technique that measures the absorption of ultraviolet and visible light by a material. This method distinguishes substances based on their electronic transitions, providing insights into their photochemical behavior. One of the key characteristics of UV-Vis spectroscopy is its ability to analyze the absorbance spectra of various compounds quickly.
This technique is beneficial because it typically requires minimal sample preparation and offers high sensitivity. Unique features of UV-Vis spectroscopy include the capability to monitor real-time changes in a sampleโs absorption characteristics as it undergoes photodegradation. The advantages of this method include its speed and reliability in providing quantitative results, but it can be limited by the presence of complex matrices that affect readings.
Fluorescence Spectroscopy
Fluorescence spectroscopy involves the emission of light by substances after they absorb photons. This technique is particularly useful in assessing the photostability of materials as it provides detailed information about electronic states and molecular environments. A notable characteristic of fluorescence spectroscopy is its high sensitivity, often allowing for the detection of low concentrations of compounds.
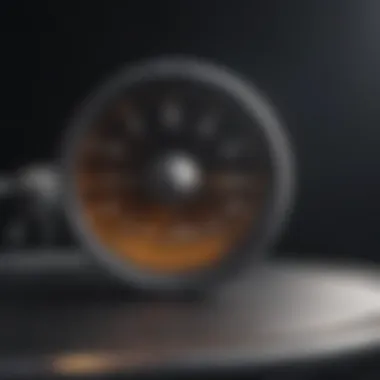
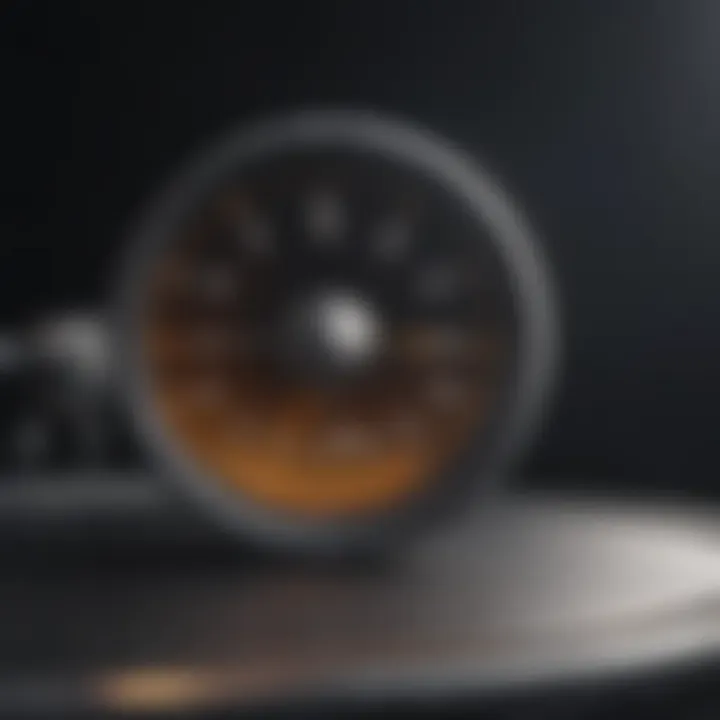
Fluorescence spectroscopy is popular in photostability assessments for its capability to enhance detection limits significantly. The unique feature of this method is its ability to reveal dynamic processes in a sample. It enables researchers to study excited states and energy transfer phenomena effectively. While the advantages of fluorescence spectroscopy are substantial, it may face challenges from solvent effects or variations in sample concentration which can impact the spectroscopic data quality.
Chromatographic Techniques
HPLC Analysis
HPLC analysis, or High-Performance Liquid Chromatography, is a crucial technique used for separating mixtures. It allows for the analysis of photostability in materials by resolving different components within a mixture based on their interaction with a stationary phase. The efficiency of HPLC comes from its high resolution and capacity to analyze complex samples.
This technique is beneficial for quantifying degradation products and understanding stability under light exposure. A unique feature of HPLC analysis is its versatility, as it can be adapted for various types of samples. However, it requires careful method development to optimize conditions, which can sometimes be a drawback.
Thin-Layer Chromatography
Thin-layer chromatography (TLC) is another vital analytical method for evaluating photostability. It offers a simple and quick means to assess the stability of materials by separating components on a flat surface. The key characteristic of TLC is its accessibility and ease of use in laboratories, making it a popular choice for preliminary analysis.
TLC can provide immediate visual results, which is an advantage in rapid assessments. Its unique feature is its capability to approximate the purity of a sample or detect specific degradation products. However, TLC may not offer the same quantitative precision as other methods, making it more suitable for qualitative assessments.
Accelerated Aging Tests
Accelerated aging tests are critical for assessing the photostability of materials under accelerated conditions. These tests simulate long-term exposure to light and environmental stressors in a shorter time frame. By utilizing specific temperature and humidity settings, researchers can efficiently predict how materials will behave over prolonged exposure.
Such tests provide rapid insights into potential degradation pathways and help researchers optimize formulations before product releases. Considerations must be taken into account when designing these tests to ensure that they accurately reflect real-world conditions. Overall, incorporating accelerated aging tests into the photostability assessment framework adds significant value to the evaluation process.
Applications of Photostability
The significance of photostability resonates across various domains, providing essential insights pertinent to both practical applications and theoretical frameworks. Understanding photostability enables professionals in material science, pharmaceuticals, and environmental studies to make informed decisions regarding the use and management of light-sensitive materials.
In material science, photostability plays a crucial role in the fabrication and longevity of products. Photostable materials are less prone to degradation when exposed to light, thus enhancing their performance and utility. This is particularly important in industries where durability and reliability are paramount, such as the construction and electronics sectors. Furthermore, the pharmaceutical industry relies on the photostability of drugs to ensure efficacy throughout their shelf life, potentially saving costs associated with formulation and re-testing. In environmental studies, understanding how pollutants break down under light exposure informs risk assessment and remediation efforts. By grasping the implications of photostability, researchers and practitioners can improve the design of innovative solutions that address the myriad challenges faced in their respective fields.
In Material Science
Polymeric materials
Polymeric materials have gained attention due to their versatility and adaptability. Their photostability determines their suitability in various applications, especially where exposure to light is unavoidable. A key characteristic of these materials is their ability to absorb light and convert it without significant degradation. This quality makes them a popular choice in products like coatings and packaging. However, their photostability can greatly vary depending on the polymer's composition and structure.
Their unique feature possibly lies in their molecular design, allowing for modifications that enhance photostability. However, one must consider the trade-off between flexibility in design and the actual performance under specific light conditions.
Nanocomposite materials
Nanocomposite materials incorporate nanoparticles to enhance the properties of traditional polymers. The integration of these nanoscale components leads to improved mechanical strength and photostability. The enhanced photostability of nanocomposites makes them increasingly valuable in applications such as outdoor furnishings and automotive parts. A notable advantage of these materials is their ability to prolong the lifespan of products, which is critical in commercial applications.
However, the complexity and cost associated with fabricating nanocomposites can be potential drawbacks. Understanding the balance between benefits and limitations is essential for their effective use in material science.
In Pharmaceuticals
Drug formulation
In drug formulation, photostability is vital for ensuring the efficacy and safety of pharmaceutical products. Drugs often contain active compounds that can degrade upon exposure to light. Thus, understanding their photostability allows formulators to create effective packaging solutions, preserving drug integrity. The key characteristic of this process is the careful selection of excipients and containers that protect the active ingredients from photo-induced degradation.
Photostability is beneficial because it extends the usability of medications, particularly those that are light-sensitive. However, the challenge lies in distinguishing between compounds based on their inherent stability in light, which requires extensive testing.
Shelf life determination
Shelf life determination involves evaluating how long a product can maintain its intended use without significant change. Photostability testing is crucial in this process, especially for light-sensitive products. A notable characteristic is that testing can reveal how environmental factors interact with light to affect stability. By determining shelf life effectively, manufacturers can confidently assert product quality and safety. However, inaccuracies in testing procedures can lead to overestimating the shelf life, resulting in potential consumer safety issues. Continuous improvement in testing technologies is necessary to mitigate these risks.
In Environmental Studies
Photodegradation of pollutants
The photodegradation of pollutants is an important environmental concern. It refers to the process by which light energy breaks down harmful substances, rendering them less toxic. A key characteristic is that not all pollutants degrade at the same rate or by the same mechanisms when exposed to light. Understanding these variations aids in developing effective remediation strategies. Photodegradation is beneficial as it can lead to natural pollution reduction processes. However, the incomplete breakdown of some pollutants can lead to harmful byproducts, posing further risks to ecosystems.
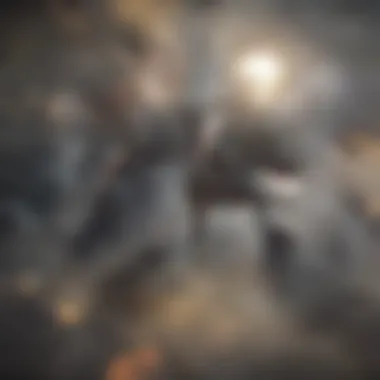
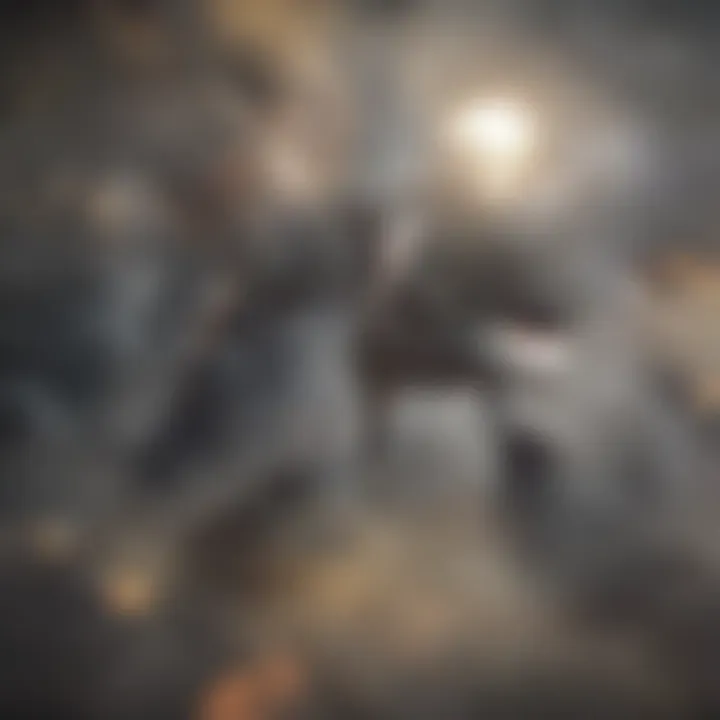
Effects on ecosystems
The effects of pollutants on ecosystems, particularly their photodegradation, must be examined to understand overall environmental health. Disruptions caused by photostable contaminants can have cascading effects on food chains and biodiversity. The key characteristic of this aspect is that light can either enhance or mitigate the harmful effects of pollutants. Knowing these effects is essential for formulating policies about environmental protection. While the photostable nature of certain pollutants may imply resilience, it also highlights the long-term risks to ecosystems.
Understanding photostability goes beyond theoretical significance; it encompasses practical applications that influence the design and efficacy of materials.
Challenges and Future Directions
Challenges in photostability research are vital for everyone involved in developing light-sensitive materials. Understanding these challenges enhances the approach taken to mitigate them and paves the way for innovative applications. This section details the significant obstacles current studies face and discusses burgeoning areas that show promise for future investigation. Addressing these challenges is essential for advancing the field and improving the performance of materials that encounter light exposure.
Limitations of Current Research
The study of photostability is still evolving, but it is riddled with limitations that hinder comprehensive understanding.
- Limited Scope: Much of the research focuses on a narrow range of materials, often neglecting others that may exhibit unique photostability behaviors.
- Variability in Conditions: Many studies do not replicate real-world conditions, leading to results that may not be applicable in practical scenarios.
- Standardization Issues: There is a lack of standardized methods for measuring photostability, which complicates comparisons between studies.
- Understudied Mechanisms: Some mechanisms underlying photostability are not fully understood, impeding the development of reliable predictors for the stability of materials.
These limitations suggest a need for broader and more rigorous research methodologies.
Emerging Research Areas
With the challenges established, new avenues for exploration are emerging. These areas hold significant potential for expanding knowledge and application of photostability.
Smart materials
Smart materials represent a dynamic area of research with a capacity to significantly enhance photostability. These materials can adapt to changes in their environment, including light exposure. A key characteristic of smart materials is their ability to respond to stimuli, such as temperature and light, which can optimize their performance. The unique feature of these materials is that they can incorporate mechanisms that actively enhance photostability, making them beneficial for long-term applications in various fields.
The advantages of smart materials include their inherent adaptability and responsiveness. However, one disadvantage is the complexity involved in their synthesis, which may lead to higher production costs.
Stabilization techniques
Stabilization techniques form another critical emerging area in photostability research. These methods focus on enhancing the durability of materials when exposed to light. A key characteristic of stabilization techniques is their ability to improve the resistance of materials to photodegradation through various means like additives or surface treatments. This makes them a popular choice among researchers aiming to prolong material life in light-exposed environments.
A unique feature of stabilization techniques is that they can be tailored to specific materials and their intended applications. The advantages of these techniques include increased material longevity and reliability. On the downside, there could be compatibility issues with certain formulations, which require thorough understanding and testing.
Investing in research on these challenges and emerging areas is essential to unravel the complexities of photostability and its applications.
Closure
The conclusion serves as a vital component of this article, synthesizing the diverse elements discussed and providing a holistic view of photostability. It emphasizes the key principles, factors, and applications of photostability that have been explored throughout the text. Understanding these aspects is essential for several reasons.
First, the summary of findings unravels the intricate mechanisms that define photostability. This understanding is pertinent not only for researchers but also for practitioners in various fields such as materials science and pharmaceuticals, where light exposure plays a significant role in material degradation.
Secondly, the conclusions drawn here highlight the importance of photostability in ensuring the longevity of sensitive materials. For industries relying on photostability, adopting appropriate measurement techniques and mitigating adverse factors are critical steps toward effective product formulation.
Moreover, discussions around the implications of the findings underline the necessity for ongoing research into emerging areas. Research that addresses current limitations and explores innovative stabilization techniques will be instrumental in advancing our knowledge and application of photostability in real-world settings.
"Understanding photostability is not just an academic endeavor; it is crucial for innovation across multiple disciplines."
Summary of Key Findings
The exploration of photostability has revealed several key findings. These include:
- Mechanisms of photostability: A detailed overview of photochemical and non-photochemical processes, explaining how materials react to light exposure.
- Factors that influence stability: Examples include chemical composition, environmental conditions like temperature and humidity, and physical properties such as surface morphology.
- Measurement techniques: Various approaches such as spectroscopy and chromatography have been evaluated for their efficacy in assessing photostability.
- Applications across fields: From material science to pharmaceuticals, the implications of photostability manifest in everyday life, showing its relevance to environmental studies as well.
Implications for Future Research
Looking forward, the implications for future research are substantial. Areas that merit attention include:
- Smart materials: The development of materials that either adapt or change properties when exposed to light could significantly enhance product performance and longevity.
- Stabilization techniques: Investigating novel methods for enhancing intrinsic and extrinsic photostability is critical. This includes not only material formulation but also environmental controls during product usage and storage.
- Longitudinal studies: Conducting long-term studies to better understand how materials behave under cumulative light exposure can provide deeper insights into their stability.
In summary, research in photostability holds the potential for notable advancements, reinforcing its significance in various applications. It is essential for future studies to bridge existing knowledge gaps and explore innovative pathways to ensure the effective utilization of materials designed for exposure to light.