Exploring Ferrites: Definitions and Applications
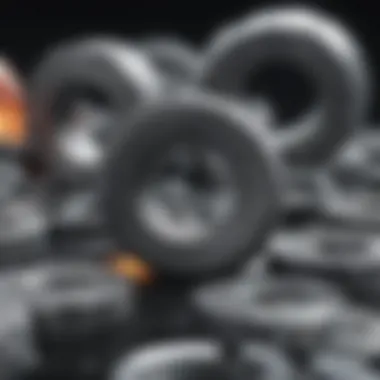
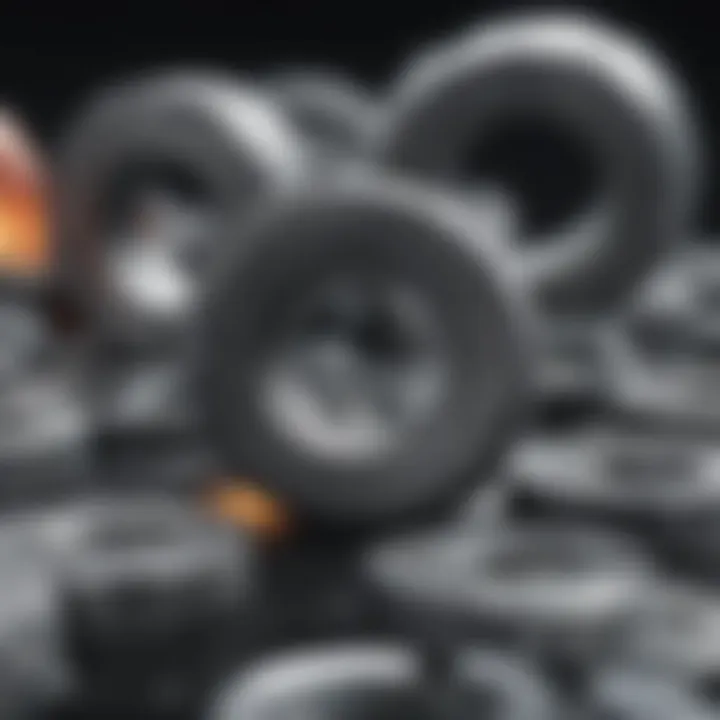
Intro
The world of ferrites is intricate, yet immensely significant in various domains of science and technology. Ferrites, primarily defined as ceramic compounds composed of iron oxide combined with metal oxides, play pivotal roles in modern applications ranging from electronics to material sciences. Understanding these materials is not merely an academic pursuit; it reflects broader implications in innovation and practical solutions in our day-to-day life. To dissect this multifaceted subject is both enlightening and essential for anyone striving to grasp the dynamics of contemporary technology.
In this article, we aim to explore the fundamental characteristics and classifications of ferrites, demystifying their properties, and elucidating how they function in different environments. Various industries depend on these materials for their unique magnetic properties, which allow them to store significant amounts of data and contribute to energy efficiency. By delving deeper into how ferrites fit into the puzzle of technological advancements, we offer a resource that combines theoretical foundations with real-world applications—valuable for students, researchers, and industry professionals alike.
"The potency of ferrites lies not just in their material makeup but in their ability to drive progress across several fields."
This rich narrative will guide you through the key findings of ferrites, their applications, and the methodology behind scientific research in the field, adapting a tone that balances both formal and informal styles. From the definitions to the possible future applications, we intend to build a thorough understanding of ferrites that resonates with a discerning audience.
Fundamentals of Ferrites
Understanding the basics of ferrites is crucial in unraveling their significance in modern science and technology. Ferrites, a blend of iron oxide with other metallic oxides, pave the way for various applications, particularly in electronics. Getting clear on what ferrites are and their core characteristics sets the stage for a deeper exploration into their composition and uses.
Composition of Ferrites
Basic Elements
The primary building blocks of ferrites typically include iron along with one or more other metal oxides. Iron adds desirable magnetic properties, while metals like zinc or nickel contribute to other essential attributes. Ferrites made from these combinations achieve a balance of characteristics required for electronic components. The presence of iron is significant here; it’s like the backbone of ferrites, providing stability and performance.
One special feature of these basic elements is their ability to form compact structures that enhance magnetic performance. This compactness is a big reason ferrites are favored in high-frequency applications. However, variability in the composition can lead to different overall behaviors, and that means each ferrite type can have its unique benefits or challenges.
Ionic Structure
When we speak of ionic structure, we touch on how ions are arranged within the ferrites. Typically, these materials have a face-centered cubic lattice structure. The arrangement of ions in this way directly influences magnetic properties, which makes it a hot topic in our exploration.
A notable characteristic here is the dual cation arrangement; ions occupy both tetrahedral and octahedral sites in the crystal lattice. This unique feature boosts the magnetic interactions within the ferrite. However, this can also introduce complexity during the synthesis stage, requiring careful control of conditions to yield desired materials.
Chemical Formulas
The chemical formulas of ferrites provide insights into their elemental composition. One common example is nickel ferrite, represented as NiFe₂O₄. The formula gives a snapshot of the elements involved, highlighting the ratio of metals to oxygen.
In terms of significance, these formulas facilitate understanding of physical properties associated with different ferrite types. For instance, the differing metal ratios can drastically alter magnetic permeability. It's a tool to design ferrites for specific functions, though one must note that using these formulas demands a grasp of how different compositions can impact performance.
Physical Properties of Ferrites
Magnetic Characteristics
Magnetic characteristics of ferrites are among the most compelling attributes, making them indispensable in many applications. Ferrites often exhibit high magnetic permeability and low coercivity, which means they can be magnetized quickly and demagnetized easily. This property is a key driver for their use in inductors and transformers.
A standout trait is their ability to retain magnetization, giving rise to several applications in electronics. However, it can lead to losses in high frequencies, so the trade-off must be examined. Selecting the right ferrite for the job can hinge on understanding these magnetic characteristics.
Electrical Conductivity
The electrical conductivity of ferrites is quite limited, which makes them insulative to a degree, but this is not a disadvantage. The lower conductivity helps prevent eddy currents that can cause energy losses. In applications where high-frequency signals are common, the insulation is crucial as it maintains performance efficiency.
Ferrites often exhibit what is known as mixed conductivity, with both ionic and electronic forms. This unique feature can be advantageous for specific applications, particularly where a balance between insulating and conducting materials is required. However, this necessity requires careful material selection to avoid unwanted conductivity issues in the final product.
Thermal Behavior
Lastly, the thermal behavior of ferrites is important for their reliability and longevity. They usually withstand high temperatures well, which allows their use in a range of environments without compromising performance. A key characteristic is that ferrites can retain their magnetic properties even when heated, which is vital in applications like transformers that often generate heat.
Interestingly, their thermal expansion can vary widely based on composition, so it must be considered when selecting materials for specific heat-sensitive tasks. Ignoring these traits could lead to failures in high-heat applications, emphasizing the need for diligent research in this area.
Historical Context of Ferrites
Discovery Timeline
The path ferrites took from obscurity to ubiquity has been quite remarkable. The term 'ferrite' first began appearing in the scientific lexicon in the early 20th century, but significant advancements only kicked off post-World War II. This timeline places ferrites at the intersection of electronics evolution.
One unique feature worth noting in this timeline is how the needs of wartime technology pressed researchers into new materials, sparking innovations that created the ferrites we utilize today. These advances paved avenues in microwave technology that resonate in today’s tech landscape.
Evolution of Usage
When we analyze how ferrites have been adapted over the years, a clear evolution emerges. Initially used in basic electrical components, ferrites swiftly made their way into consumer electronics by the 1960s. This transition illustrates dynamic adaptations to meet user needs.
A major characteristic of ferrites is their versatility; they serve diverse roles, from filters to cores, illustrating their growing importance in advancing technological infrastructures. However, increasing demands have led to exploration of new ferrite variants and composites, pushing the boundaries of their applications.
Key Researchers and Contributions
Several key figures have significantly impacted the field of ferrites, shaping their development and application. Notable contributions from researchers like Wilhelm Eduard Weber in the 1880s helped lay the groundwork for understanding magnetic materials, which directly ties to how modern ferrites are perceived.
It's intriguing to note how collective contributions have built an ever-expanding knowledge pool, which in turn informs new research directions in ferrite applications today. However, as diverse as these contributions are, a common thread is the ongoing pursuit of improving ferrite capabilities to meet the fast-paced demands of technology.
In summary, grasping the fundamentals of ferrites not only provides insight into their exceptional properties but also reveals how they became integral to various technological advancements. Through rigorous examination of their composition, physical properties, and historical context, we gain a holistic understanding of ferrites, establishing a foundation for exploring their diverse applications.
Classification of Ferrites
The classification of ferrites is crucial in understanding their diverse properties and applications across various domains. With a variety of structural types and compositions, distinguishing different types of ferrites helps researchers and engineers select the appropriate material for specific uses. This not only enhances the performance of devices but can also lead to cost-effective solutions in technology and manufacturing.
Different classes of ferrites possess unique characteristics, be it in magnetic strength, electrical resistivity, or thermal stability. As such, a clear classification aids in discovering innovations and optimizing current technologies, thereby cementing ferrites' significance in the modern tech ecosystem.
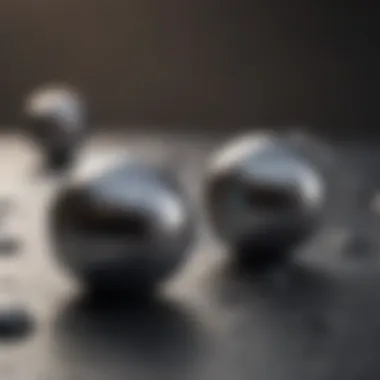
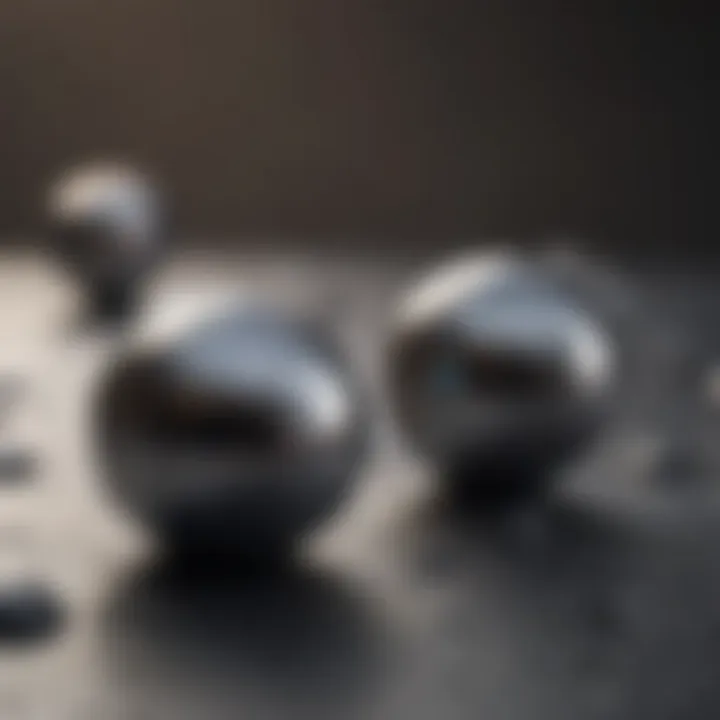
Spinel Ferrites
Structure and Properties
Spinel ferrites are notably defined by their cubic crystal structure, allowing for a variety of metals to occupy different lattice sites. This unique architectural arrangement contributes to their magnetic properties, making them sought after in the industry.
A key characteristic of spinel ferrites is their high permeability and low coercivity, which makes them particularly useful in applications requiring efficient magnetic coupling. The stability of this structure enables them to maintain their magnetic properties under varying conditions, which is a beneficial aspect when considering reliability in electronic applications. Yet, while they perform well within specific parameters, their saturation magnetization is not the highest, leading to a potential limitation depending on the usage context.
Common Spinel Ferrites
Among the common spinel ferrites, magnetite (Fe3O4) and zinc ferrite (ZnFe2O4) are often highlighted due to their wide range of applications, particularly in electronics and magnetic storage. These materials are favored because they exhibit robust magnetic behavior combined with excellent electrical insulation, making them ideal candidates for a variety of technologies.
The unique feature of common spinel ferrites is their ability to alter magnetic properties based on composition. For instance, adjusting metal ion concentrations can change the magnetic response, allowing customizability for specific tasks. This versatility is a significant advantage; however, it can also pose challenges in manufacturing uniform products at scale.
Applications
The applications of spinel ferrites span various fields, but they are most notable in electronic devices. Their usage in inductors, transformers, and magnetic cores illustrates their pivotal role in energy efficiency and signal processing. These materials are recognized for enhancing device performance and longevity, which is particularly attractive in the fast-paced electronics industry.
Additionally, their magnetic properties allow them to be used in both high-frequency devices, such as wireless communication systems, and in power electronics. Noteworthy, however, is the challenge of integrating these ferrites into miniaturized devices, as poor scaling can lead to performance issues, making optimization key for future technologies.
Hexaferrites
Crystal Structures
Hexaferrites distinguish themselves by their complex crystal structures, often exhibiting various symmetries. This complexity contributes to their high magnetic anisotropy, resulting in superior magnetization directionality, a feature often sought in magnetic applications.
Due to the arrangement of iron ions within their lattice, hexaferrites showcase remarkable stability in magnetic properties compared to spinel ferrites, making them particularly appealing for harsh environmental conditions. This advantageous feature lends itself well for applications in telecommunication technologies where durability is a critical necessity.
Magnetic Properties
When it comes to magnetic properties, hexaferrites are known for their higher coercivity, which highlights their resistance to demagnetization. This characteristic makes them suitable for permanent magnet applications. Their ability to maintain a strong magnetic field over time is a noted benefit when considering long-term performance in devices requiring consistent magnetic force.
However, while their extraordinary coercivity is an advantage, it can complicate the engineering processes involved in demagnetizing them for temporary applications. This barrier occasionally limits their usability in designs that require alternating magnetic fields.
Technological Uses
In terms of technological uses, hexaferrites are often employed in microwave devices, magnetic recording media, and various radar systems due to their favorable magnetic properties. Their usage in high-frequency technology is increasingly vital; they provide high efficiency and performance for today's rapid communication demands.
While hexaferrites are gaining popularity, their relatively high production costs compared to standard ferrites could necessitate careful planning in new applications, particularly in consumer electronics.
Mixed Ferrites
Definition and Characteristics
Mixed ferrites often combine different metal ions, allowing for a fusion of properties from varying components into a single material. This deliberate mixing offers a trade-off between the magnetic characteristics and electrical properties, leading to a versatile material suitable for numerous environments.
An essential trait of mixed ferrites is their ability to tailor the magnetic and electrical response by adjusting the specific ratios of constituent elements. This customization proves particularly valuable in applications tailored to specific needs, improving devices wherever there's a requirement for both magnetism and conductivity. Nevertheless, standardization in producing these ferrites can be challenging, making quality control pivotal to consistency.
Synthesis Methods
The synthesis methods for mixed ferrites can vary, reflecting the diversity of compositions involved. Sol gel techniques, for instance, are popular for creating fine powders with homogenous distributions of metal ions. This method is advantageous for achieving specific properties, yet it may be limited by scale and complexity in production.
Another method, the traditional ceramic method, offers ease of integration into existing manufacturing processes, though it may not reach the same level of precision as sol gel techniques. Thus, while choices of synthesis methods present distinct advantages and disadvantages, the ultimate application needs must drive the pathway selected.
Examples
In the realm of mixed ferrites, examples abound, but those combining nickel and zinc—such as NiZn ferrites—are notably recognized for their broad usages in telecommunications and high-frequency applications. These ferrites marry affordability with performance, crucial for most modern electronics.
Conversely, products like MnZn ferrites are praised for their high magnetic permeability, yet they can suffer from frequency limitations due to eddy current losses. This dichotomy between utility and the potential drawbacks necessitates deep consideration when designing devices intended for specific tasks.
As we analyze these materials, it becomes clear that the careful classification of ferrites not only reveals their unique characteristics but also guides advancements and innovations in technology, reflective of the ongoing demand for optimized materials in the ever-evolving scientific landscape.
Magnetic Properties of Ferrites
The study of magnetic properties of ferrites is crucial for understanding how these materials perform in various applications. Ferrites exhibit unique behaviors in the presence of magnetic fields, which dictate their use in technology and industry. Therefore, exploring these properties not only highlights the underlying principles of magnetism but also showcases the multifaceted roles of ferrites in modern electronics, data storage, and environmental applications. These materials are not just passive recipients of magnetic fields; rather, they interact dynamically with magnetic forces, leading to substantial consequences in their operational capabilities.
Fundamental Concepts of Magnetism
Magnetization
Magnetization refers to the extent to which a material can be magnetized. For ferrites, this property is significant as it dictates their functionality in devices like inductors and transformers. The key characteristic of magnetization in ferrites is its response to an external magnetic field. When exposed to such fields, ferrites can align their internal dipoles, greatly enhancing their magnetic strength. This makes them a popular choice for applications requiring efficient magnetic energy storage and transfer.
The unique feature of magnetization is its dependence on the material’s structure and composition, particularly in the spinel and hexaferrite forms. A notable advantage is that these materials can achieve high saturation magnetization without excessively high energy input, making them cost-effective in production. However, their performance may diminish at elevated temperatures, which is a critical consideration for designs intended for varied environments.
Coercivity
Coercivity is a measure of the resistance a material has to becoming demagnetized. In ferrites, coercivity is pivotal because it determines how easily the material can retain its magnetic properties. A key characteristic of coercivity in ferrites is the ability to maintain magnetic states without significant energy loss, which proves beneficial in high-frequency applications where stability is essential.
The distinguishing factor here is the high coercivity levels observed in certain ferrites, which allows for their effective use in permanent magnets, memory storage solutions, and more. While higher coercivity enhances performance in many applications, it may complicate the manufacturing process, requiring precise control over the synthesis methods to yield the desired properties.
Remanence
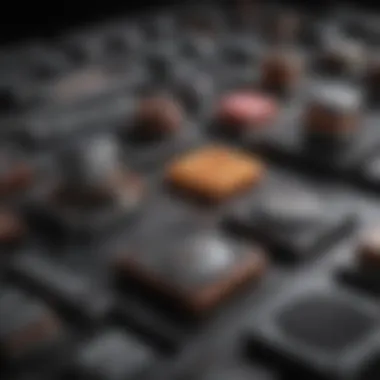
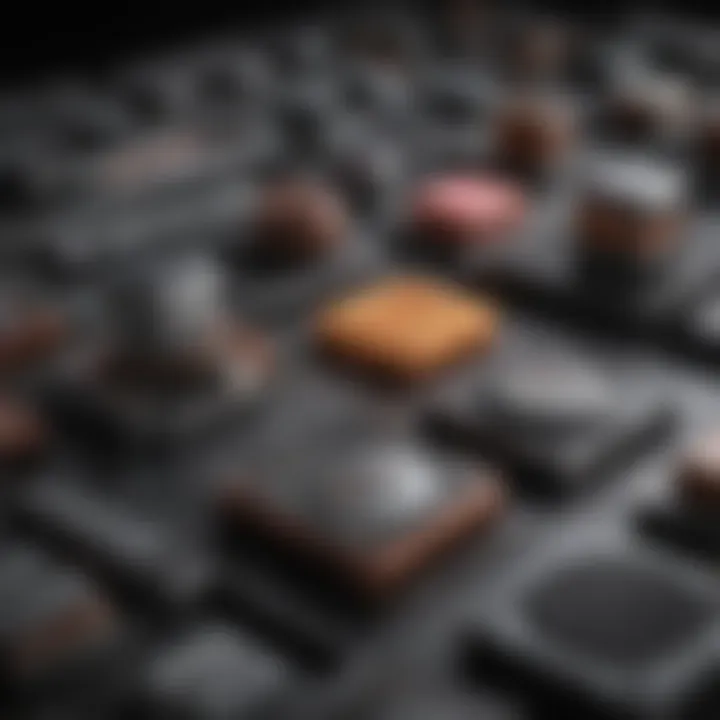
Remanence is the measure of the magnetization remaining in a material after an external magnetic field is removed. For ferrites, this property highlights their ability to hold magnetic memory, making it instrumental in applications like data storage. The key characteristic of remanence is that it directly influences the longevity and reliability of stored information.
A particular advantage of ferrites is the comparatively high remanence, making them effective for uses in sensors and inductors. However, one should consider that excessive remanence can lead to magnetic saturation, which constrains the material's overall performance in certain electronic circuits. Balancing remanence with other properties is essential when applying ferrites in innovative ways.
Hysteresis Behavior
Hysteresis Loop Analysis
Hysteresis loop analysis provides insights into how ferrites react to cyclic magnetization. By assessing the shape of the hysteresis loop, researchers can discern critical aspects of ferrite behavior under magnetic stress. The key characteristic of this analysis is its ability to visualize energy losses and magnetic saturation points, which are pivotal in determining operational efficacy.
A unique feature is that it helps in evaluating the design parameters for electronic components, allowing engineers to tailor ferrite materials to specific needs. However, an inherent disadvantage is the complexity of measurement, requiring precise instruments to effectively capture the hysteresis behavior of ferrites in various conditions.
Energy Losses
Energy losses in ferrites occur primarily during magnetization and demagnetization cycles. These losses represent a key challenge in the efficiency of devices like transformers and inductors. The notable characteristic of energy losses in ferrites is that they are unavoidable but can be mitigated through appropriate material selection and design choices.
The unique feature in this context is that while ferrites inherently exhibit some level of energy loss, advances in manufacturing and material science are continuously pushing to reduce these losses, ensuring optimal performance in energy-constrained environments. Nevertheless, excessive energy loss could lead to overheating and reduced lifespan of electronic components, making it essential to conduct thorough thermal analyses.
Influence of Temperature
Temperature has a profound impact on the magnetic properties of ferrites. As the temperature varies, so does the magnetization, coercivity, and remanence of the material. The key characteristic of temperature influence is that it alters the magnetic domain behavior within ferrites.
One distinct feature here is that certain ferrites possess temperature stability, allowing them to maintain their magnetic properties over a wider range of temperatures, making them more suitable for extreme conditions. However, if not accounted for, variations in temperature could drastically affect component efficiency and longevity. Understanding these fluctuations is crucial for engineers designing ferrite-based applications under different environmental conditions.
"The interplay of temperature, magnetization, coercivity, and remanence in ferrites underlines the complexity and beauty of their functionality in technology."
In summary, the magnetic properties of ferrites are foundational to their applications and effectiveness in various fields. An understanding of magnetization, coercivity, remanence, hysteresis behavior, and temperature influence provides significant insights that pave the way for advancing the use of these materials in innovative ways.
Applications of Ferrites
The modern world is heavily intertwined with the applications of ferrites, which are a class of ceramic compounds made mainly of iron oxide and other metal oxides. Their applications span across various sectors, including electronics, data storage, and even environmental technologies. Understanding these applications is crucial for any researcher or professional looking to capitalize on the unique properties of ferrites. These materials are not only effective but also provide economical solutions to numerous challenges in modern technology.
Ferrites in Electronics
Inductors and Transformers
Inductors and transformers represent one of the most significant uses of ferrites in the electronics sector. These components utilize the excellent magnetic properties of ferrites to effectively store and transfer energy. Ferrites are particularly advantageous due to their high magnetic permeability, which allows for larger inductance values in smaller sizes. This minimizes the impact on the design's footprint. When looking at the efficiency of these components, ferrites help improve performance, making them a go-to choice in modern circuit design.
The unique feature of ferrite cores is their ability to minimize energy losses during operation, primarily due to lower eddy current losses. However, ferrites do come with their downsides. Their temperature sensitivity can affect performance in high-temperature applications, which must be accounted for in design considerations.
Magnetic Cores
Magnetic cores are another integral aspect where ferrites shine. Used in transformers, inductors, and sensors, ferrite cores reduce losses significantly during magnetic flux changes. Their low electrical conductivity leads to much lower eddy current losses compared to metal cores, making them ideal for high-frequency applications. These cores contribute significantly to the overall functionality and efficiency of electronic devices.
One notable characteristic of magnetic cores is their density. Ferrites can be made very dense, enabling more compact designs in electronic devices. However, the brittleness of ferrites can be a limitation, particularly in environments where physical shocks can occur.
High-Frequency Devices
When it comes to high-frequency devices, ferrites play a critical role due to their tailored magnetic properties and low loss characteristics. In RF applications, ferrites are often used in antennas and filters, improving the efficiency of signal transmission. Their ability to support high frequencies without significant energy losses makes them indispensable in telecommunications.
What’s particularly noteworthy is how ferrites, by manipulating their composition, can achieve a wide range of frequencies and applications. Still, it is essential to recognize that the performance of high-frequency devices can degrade with environmental exposure, such as humidity or temperature changes. This variability requires careful engineering for long-term reliability.
Ferrites in Data Storage
Magnetic Recording Media
In data storage, ferrites have a significant part to play. Magnetic recording media primarily relies on the unique properties of ferrites to efficiently store data. With magnetic tape and hard drives, ferrites enable reliable recording through their stable magnetic characteristics. This stability is crucial for maintaining data integrity over time.
The advantage here is that ferrites provide a medium with reproducible magnetic properties, leading to more efficient read/write operations. However, the moving towards solid-state storage technologies presents a challenge for traditional ferrite-based magnetic media, compelling researchers to innovate continually.
Memory Devices
Ferrites are also utilized in various memory devices, such as magnetic RAM (MRAM). The standout feature of this application is the non-volatility of memory storage, allowing data retention even without power. This makes ferrites a favorable choice for further advancements, combining speed and power efficiency.
Challenges persist, though. Integration into existing microelectronics can be tricky, demanding detailed research and development for widespread use.
Future Trends
Looking ahead, the behavior of ferrites in the realm of data storage is evolving. Researchers are focusing on maximizing the storage capabilities and speeding up read/write times. The exploration of nanostructured ferrites has opened doors to potential breakthroughs, providing avenues for improving data density and stability.
This innovation is promising but comes with hurdles such as cost and scale-up for industrial applications.
Ferrites in Environmental Applications
Water Treatment
Ferrites, particularly those modified chemcially, have emerged as an effective solution for water treatment processes. They possess unique properties that facilitate the removal of heavy metals, thus improving water quality. Their efficiency in capturing contaminants makes them a beneficial option for technological advancements aimed at environmental sustainability.
The defining characteristic of ferrites for water treatment is their high surface area and reactivity, which enhances the purification process. However, there are considerations regarding the disposal and recycling of used ferrite materials to mitigate environmental impact.
Pollution Control
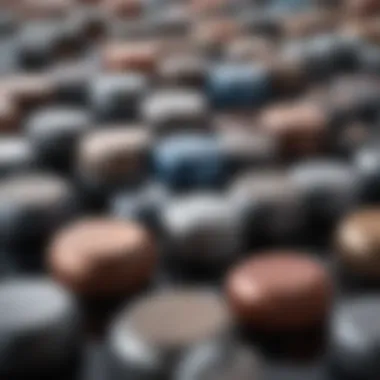
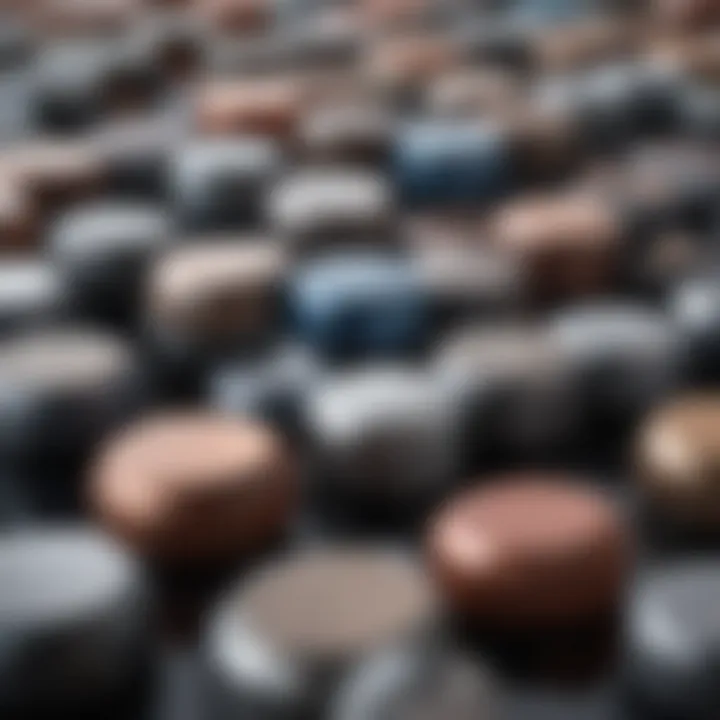
In pollution control, ferrites have been instrumental in catalytic processes that reduce harmful emissions. For instance, they are used in filters and sorbents to clean up exhaust gases from industrial activities. Their capability to bind with various pollutants makes ferrites a popular choice in this domain.
A concerning aspect is that while they can be highly efficacious, the long-term stability and maintenance of ferrite-based systems require continuous evaluation to ensure efficacy over time.
Green Technologies
Lastly, ferrites play a pivotal role in green technologies. Their application in renewable energy systems, such as inverters and power converters, underscores their importance in the transition towards cleaner energy solutions. The attractive feature of ferrites in these applications is their low environmental footprint and efficient energy processing capabilities.
Nevertheless, ongoing research is focused on improving the performance of ferrites in diverse environmental conditions, ensuring that they contribute positively in the long run.
Theoretical Framework of Ferrites
The theoretical framework behind ferrites plays a significant role in understanding their properties and applications. By delving into this knowledge, researchers, students, and professionals can harness ferrites' unique magnetism and electrical characteristics. This section illuminates the theoretical intricacies that underpin ferrites and how they contribute to advancements in technology and materials science. It sets the stage for analyzing the microscopic interactions within ferrites, showcasing their vital importance in current and future applications.
Magnetic Domain Theory
Domain Structure
Domain structure refers to the internal arrangement of magnetic moments within a ferrite material. Each domain is a distinct region where the magnetic moments are aligned in the same direction, while neighboring domains may have different orientations. A key characteristic of domain structure is that it greatly influences the overall magnetic behavior of the material.
This feature is crucial to this article as it underscores how ferrites transition between different states of magnetization. By examining domain boundaries and interactions, one can see patterns that dictate how ferrites respond under external magnetic fields, making this understanding beneficial for those working in electronics and information storage.
The unique aspect of domain structure is its contribution to minimizing the system's overall energy. When a magnetized ferrite is demagnetized, movement of domain boundaries allows for efficient energy loss management. Though advantageous, this behavior can sometimes lead to complications in the design of ferrite-based devices due to unwanted noise in high-frequency applications.
Magnetization Processes
Magnetization processes involve how a ferrite responds to an external magnetic field and the ensuing changes to its internal alignment. This phenomenon is pivotal for explaining the operational principles behind essential applications like inductors and transformers. The defining trait of magnetization processes is their reliance on external stimuli, which may range from modest fields to significant intensities. The benefit of understanding these processes lies in the optimization of ferrite materials for various conditions, granting engineers and scientists the ability to tailor ferrite devices for specific tasks.
A unique feature of magnetization processes is how they enhance performance at high frequencies, making ferrites indispensable in electronic components. However, the challenge arises when designing for low-frequency applications, where hysteresis losses can occur. This understanding is critical for improving current technologies while also paving the way for future innovations.
Practical Implications
Practical implications of magnetic domain theory and magnetization processes are vast in the realm of ferrite technology. They translate theoretical findings into applications, impacting manufacturing and performance of ferrite materials.
A central characteristic of these practical implications is their guide role for prototyping and experimenting with new ferrite designs. This aspect is particularly beneficial for industries relying on efficiency and performance, such as telecommunications, automotive, and renewable energies.
The key element here is how closely understanding these implications supports advancements in ferrite applications. They allow for feedback mechanisms throughout the development process, linking theoretical research to real-world scenarios. However, one must be aware that the cost of developing new models may be high, and the risks associated with experimental designs can present significant hurdles.
Quantum Mechanical Models
Quantum mechanical models are pivotal in comprehending the intricate behaviours of ferrites at the quantum level. They provide a framework that explains how electron spins and interactions contribute to magnetic properties. Probing into quantum mechanical aspects enables a deeper grasp of how ferrites operate under various physical conditions and interactions.
Electronic Spin
Electronic spin is a fundamental characteristic of ferrite materials that greatly influences their magnetic properties. It refers to the intrinsic angular momentum of electrons, which contributes to the overall magnetism of the material. The significance of electronic spin in this context is well-grounded, marking it as a critical aspect of understanding ferrite behaviour.
The benefit of focusing on electronic spin lies in its direct relationship with the creation of magnetic moments within ferrites. A unique feature of this spin configuration is its alignment with external magnetic fields, allowing ferrites to exhibit various ferromagnetic properties. Strengthening this understanding is vital for applications in electronics, where precise control of magnetic fields is often required. On the downside, the inherent complexities related to electron behaviour may introduce challenges in model representation and predictive capabilities.
Energy Band Theory
Energy band theory examines how energy levels in a ferrite can be understood in terms of quantum states and how these states interact with external forces like electric fields. This theoretical approach provides insights into the conductivity and magnetic behaviour of ferrites.
The central characteristic of energy band theory is its ability to explicate electron motion under the influence of external fields, making it relevant to the understanding of ferrites' practical applications in electronics.
A unique feature of this theory is its comprehensive approach to linking electronic properties with thermal excitations. This connection serves as an advantage when analyzing ferrite performance in high-temperature environments. However, complexities arise when applying, as assumptions made during modeling may not always hold true under real-world conditions.
Magneto-optical Effects
Magneto-optical effects refer to the interactions between light and the magnetic properties of ferrites, a topic of growing interest in material science and optics. The interplay of magnetism and optics opens new avenues for developing advanced technologies like optical communication and data storage systems.
A significant characteristic of magneto-optical effects is their potential applications in spintronic devices that exploit both electronic spin and optical phenomena. This dual functionality is particularly beneficial in creating faster and more efficient devices. However, a unique challenge in this domain is ensuring that the materials used can maintain stability and reliability during operation – an area that researchers continue to explore.
Understanding the relationship between magneto-optical effects and ferrites is key in tapping into the next generation of technological advancements.
Future Directions of Ferrite Research
Research in the field of ferrites is ever-evolving, reflecting the increasing appetite for high-performance materials in modern applications. Recognizing the potential of ferrites not just as static components, but as dynamic elements in advancing technology is vital. The upcoming sections will address how innovative applications, alongside the challenges faced in manufacturing and cost, shape the future landscape of ferrite technology. This framework helps to identify where focus and investment will yield the most impactful results.
Emerging Applications
Nano-ferrites
Nano-ferrites represent a cutting-edge advance in the world of ferrite technology. Their particle size, typically in the range of 1 to 100 nanometers, grants them unique properties that distinguish them from their bulk counterparts. One notable aspect of nano-ferrites is their increased surface area, which enhances reactivity and magnetism. This makes nano-ferrites an appealing choice for various applications, from magnetic resonance imaging to catalysis.
The smaller scale allows for tailored magnetic properties, enhancing performance in electronic components such as sensors and inductors. Even with these advantages, the synthesis of nano-ferrites often presents challenges, including stability and uniformity during production. Thus, careful consideration must be afforded when applying this technology.
Biocompatible Ferrites
In recent times, biocompatible ferrites have emerged as a substantial focus in medical applications. The key characteristic here is their ability to be safely used within biological systems, making them especially valuable in drug delivery and magnetic hyperthermia for cancer treatment. These ferrites possess unique properties like low toxicity and high magnetic susceptibility, making them more effective than traditional methods.
However, the advantages must be weighed against specific limitations, such as the need for precise formulations to ensure compatibility with various biological environments and regulatory standards. Hence, when deploying biocompatible ferrites, ongoing research into their long-term effects is necessary.
Alternative Energy Sources
The demand for clean energy sources is higher than ever, leading to the exploration of ferrites in renewable energy applications. Ferrites, known for their magnetic properties, can be employed as vital components in batteries and fuel cells, aiding in energy conversion and storage processes. Their resilience and efficiency in high-frequency applications are particularly noteworthy.
This technology is beneficial for projects aimed at sustainability, yet practical challenges remain, including the efficiency of energy conversion and the costs associated with large-scale production. Optimizing alternative energy sources through ferrites can result in significant breakthroughs in energy sustainability.
Challenges in Ferrite Technology
Material Limitations
Despite their many advantages, ferrites are not without limitations. One pressing issue is their brittleness, which can hinder their practical applications in environments with vibration or stress. This characteristic demands a careful balance in the design of ferrite components, as flexibility may be needed for specific applications. Addressing these material limitations is essential for advancing their use in various industries.
Manufacturing Techniques
The manufacturing process of ferrites is another area that presents hurdles. Traditional methods such as solid-state synthesis may not meet the high demands of today’s technology. New techniques, like co-precipitation and sol-gel methods, are gaining traction due to their ability to produce fine-tuned ferrite materials. However, these innovative techniques can escalate production times and costs, prompting the exploration for more efficient methods that minimize these downsides while upholding quality and performance.
Cost-effectiveness
Cost remains a key factor in determining the feasibility of ferrite applications across industries. While ferrites offer impressive properties, their market entry often hinges on the ability to produce them at a competitive rate. Research aimed at reducing production costs, perhaps by sourcing cheaper raw materials or streamlining manufacturing processes, must be prioritized to maintain their relevance in advancing technologies.
The future research into ferrites stands on the foundational understanding of their applications and challenges, setting the stage for innovations that could transform entire industries.