Deconstructing PLA Biodegradation: An In-Depth Analysis
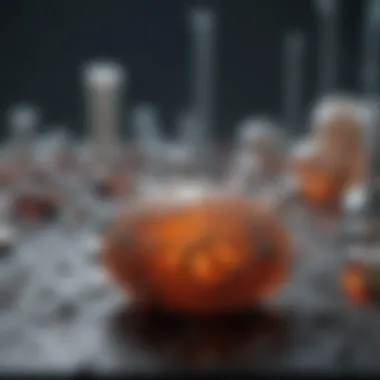
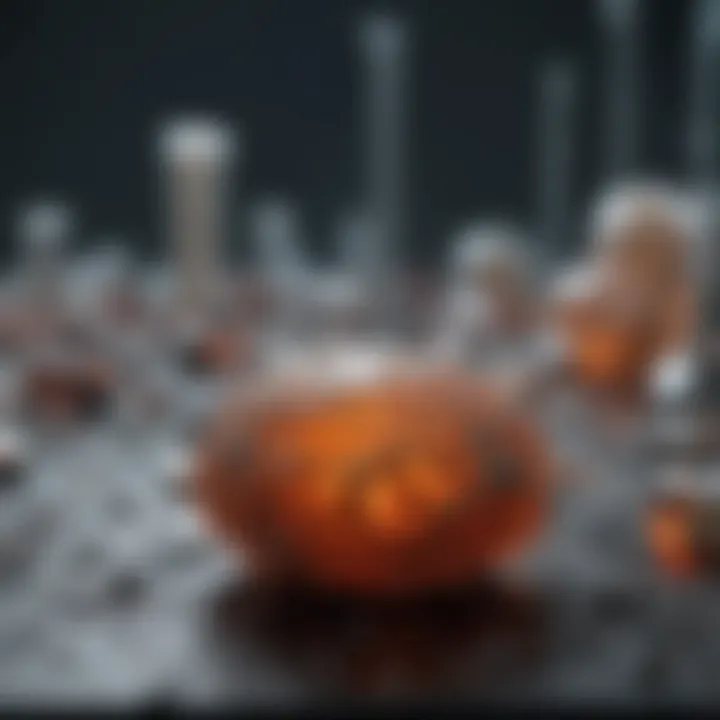
Intro
The increasing environmental concerns associated with plastic pollution have prompted significant research into alternative materials. One prominent candidate is Polylactic Acid (PLA), a biodegradable thermoplastic synthesized from renewable resources such as corn starch or sugarcane. This emphasis on sustainability invites a closer examination of PLA's biodegradation processes, exploring how it may mitigate the adverse effects of traditional plastics.
Understanding the nuances of PLA's degradation is vital for its industrial applications and environmental implications. The mechanisms that underpin its biodegradation involve a complex interaction between environmental factors and biological agents. These interactions guide the breakdown of PLA, ultimately transforming it into non-toxic byproducts that can be assimilated back into the ecosystem. This article intends to dissect these processes, revealing insights into how PLA can be a more sustainable alternative to conventional plastics in various sectors.
In the upcoming sections, a thorough analysis of the major findings will provide clarity on the biodegradation pathways, the impact of distinct environmental conditions, and the role of microorganisms.
Key Findings
Major Results
The exploration into PLA biodegradation has yielded several pivotal findings:
- PLA exhibits high biodegradability under controlled composting conditions, often achieving significant decomposition within 60 to 90 days.
- Microbial activity plays a crucial role in the degradation process, with specific strains such as Bacillus and Pseudomonas showing a marked ability to break down PLA.
- Environmental factors, including temperature, humidity, and pH levels, significantly affect the rate of biodegradation. A temperature range of 50-60 °C creates optimal conditions for microbial activity.
- Certain processing techniques, such as the addition of plasticizers or fillers, can enhance PLA's biodegradability, primarily by altering its physical properties and facilitating microbial access.
Discussion of Findings
The implications of these findings are far-reaching. The rapid biodegradation rates of PLA under specific conditions highlight its potential use in industries where rapid breakdown is beneficial, such as food packaging. Furthermore, the identification of effective microorganisms provides avenues for bioengineering and pollution remediation strategies.
The analysis also reveals a critical interplay between environmental conditions and microbial efficacy in PLA degradation. By adjusting these variables, industries can potentially optimize PLA waste management processes, reducing the overall environmental footprint.
Methodology
Research Design
The research surrounding PLA biodegradation employs a combination of laboratory experiments and field studies to assess degradation rates in various environments. By simulating different conditions, researchers can analyze the effectiveness and efficiency of PLA breakdown in real-world applications.
Data Collection Methods
Data collection involves measuring biodegradation rates through weight loss assessments, microbial colony-forming units, and analysis of byproducts through chemical testing. Observations from composting trials and controlled environments allow for a detailed evaluation of both the processes and their outcomes.
The quest for biodegradable alternatives like PLA highlights the need for integrated approaches to waste management, combining scientific inquiry with practical implementation.
This in-depth analysis of PLA biodegradation not only underscores the material's viability as a substitute for conventional plastics but also contributes to the ongoing discourse on sustainability and innovation in material science.
Intro to PLA Biodegradation
The increasing concern over plastic pollution has led to an intensified focus on sustainable materials. Polylactic acid (PLA) stands out as a significant alternative to traditional plastics. Understanding the biodegradation of PLA is crucial in assessing its environmental impact. This section introduces the fundamental concepts of PLA biodegradation while emphasizing its relevance in contemporary discussions surrounding sustainability.
Biodegradation refers to the process by which organic substances are broken down by microorganisms into simpler substances. For PLA, this means transforming it into harmless byproducts that can be assimilated into natural cycles. The topic's importance cannot be understated, as the world grapples with the consequences of widespread plastic usage.
Defining Polylactic Acid
Polylactic acid is a biodegradable thermoplastic. It is created through the polymerization of lactic acid, which is often derived from renewable resources such as corn starch or sugarcane. The unique aspect of PLA is its ability to decompose naturally, a trait that sets it apart from many conventional plastics that can persist in the environment for centuries.
When discussing PLA, it is essential to highlight its properties. PLA is known for its transparency, elasticity, and high tensile strength. These attributes make it an appealing choice across various applications, from packaging to medical uses. However, its biodegradability remains the focal point in dialogues surrounding sustainability. The vision of replacing traditional plastics with biodegradable options like PLA holds great promise.
Historical Background
The history of PLA is rooted in innovation and the quest for sustainable materials. It was first synthesized in the early 20th century but gained significant traction only in the late 20th and early 21st centuries. The rise of environmental awareness brought PLA to the forefront of discussions about alternatives to petroleum-based plastics.
Various companies have invested in developing PLA applications, particularly as technology improves. The recognition of the harmful effects of plastic waste pushed researchers and manufacturers to explore bioplastics. Today, PLA is widely adopted in many industries, marking a shift towards more environmentally conscious practices.
The historical development of PLA teaches valuable lessons about the importance of innovation in addressing pressing global challenges. As we understand the evolution of this material, it becomes clearer that the journey towards sustainability requires continuous effort, research, and development.
The Chemistry of PLA
The chemistry of Polylactic Acid (PLA) is numerous and complicated but essential for grasping its role in biodegradation. To understand the behavior of PLA in the environment, one must first comprehend its molecular structure and formation processes. The transformation of monomers into a polymer through polymerization connects with the material's properties and, consequently, its degradation behavior.
Monomer Structure and Polymerization
PLA originates from the fermentation of renewable resources, particularly corn starch or sugarcane. The fundamental building block of PLA is lactic acid. When lactic acid molecules undergo condensation, they form a polymer chain known as polylactic acid. The process can be understood as combining small units into a larger whole.
The formation of PLA involves two main types of polymerization: ring-opening polymerization and direct condensation.
**Key aspects to consider:
1.
- The molecular weight of PLA affects its thermal properties and biodegradation rate.
- The stereochemistry of lactic acid (L- or D-lactic acid) influences crystallinity and, thus, the mechanical properties of PLA.
This understanding of monomer structure and polymerization is crucial because it lays the groundwork for determining how PLA interacts with the environment during its biodegradation process.

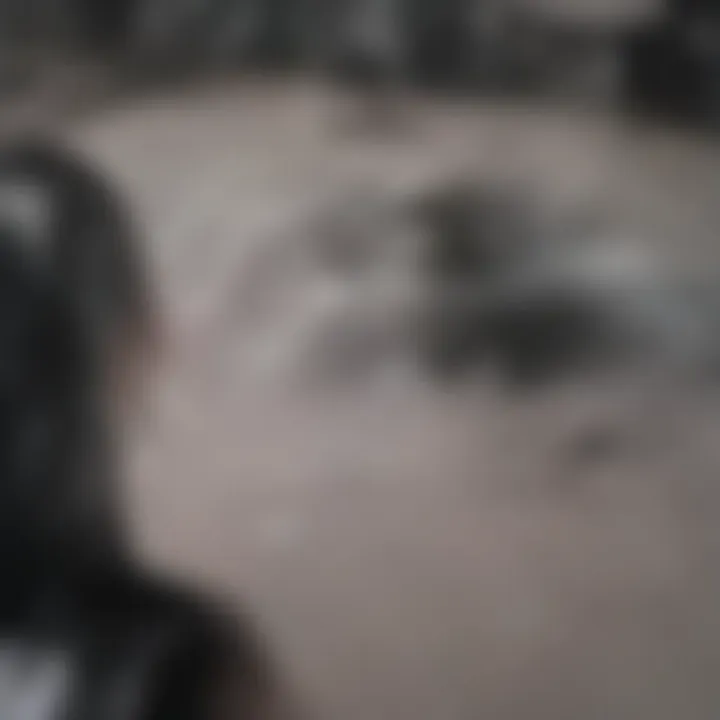
Different Types of PLA
PLA is not a singular entity; it exists in various forms that can significantly influence its applications and degradation behavior. Understanding these distinctions is paramount for selecting suitable types of PLA for specific industrial uses and research purposes.
Standard PLA
Standard PLA is the most straightforward type of polylactic acid. Its primary characteristic lies in its high purity and consistent physical properties. It has a high tensile strength and good clarity, which makes it a common choice for numerous applications like packaging and disposable tableware.
**Advantages:
- It is compostable under industrial conditions, fitting well in sustainable practices.
However, the biodegradation proceeds slowly in natural environments. This slow rate can be a disadvantage when fast decomposition is necessary.
PLA Blends and Composites
PLA blends and composites involve mixing PLA with various additives to enhance its properties. The blending improves impact resistance and flexibility, making these materials suitable for more demanding applications.
**Key characteristic:
- The flexibility and toughness are enhanced by the incorporation of materials like polyhydroxyalkanoates (PHAs).
While this flexibility is an advantage, issues related to compatibility and processing techniques could arise. This can complicate the recycling process or affect degradability.
PLA Copolymers
PLA copolymers represent another significant branch of polylactic acid. These materials involve the copolymerization of lactic acid with other monomers, leading to variations in properties. The inclusion of other monomers can drastically alter the thermal and mechanical behavior of the resulting material.
**Important aspect:
- They may offer enhanced properties depending on the ratio and type of monomers used.
The addition of different comonomers can improve the biodegradation rate as the polymer chain becomes more accessible to microbial action, thus potentially enhancing environmental performance.
Understanding these categories of PLA contributes to a larger comprehension of the implications in biodegradation processes. The chemistry behind PLA forms the cornerstone for exploring its environmental aspects and viability as an alternative to conventional plastics.
Comprehensive knowledge about the chemistry of PLA and its variances equips researchers and industries with essential information on how to model PLA's performance and degradation in environmental conditions.
Environmental Context of PLA
The environmental context of Polylactic Acid (PLA) biodegradation is critical in understanding the broader implications of its use as an alternative to traditional plastics. PLA, made from renewable resources, is celebrated for its potential benefits in reducing plastic waste. However, to appreciate its applications fully, one must consider various environmental factors that influence its biodegradation process. These elements include the distinction between biodegradability and compostability, as well as the comparison of PLA with conventional plastics, both of which merit thorough exploration.
Biodegradability vs. Compostability
Biodegradability refers to the ability of a material to break down into natural elements through the actions of microorganisms, such as bacteria and fungi, over time. PLA is regarded as biodegradable under certain conditions, typically found in industrial composting facilities. In these environments, optimal temperature, humidity, and microbial activity are present to ensure a faster breakdown of PLA into non-toxic constituents. This breakdown process leads to the formation of water, carbon dioxide, and biomass, effectively reducing the environmental impact of the material.
Compostability, on the other hand, while closely related, has specific criteria attached to it. A material must meet standardized testing for compostability to be categorized as such. This includes breaking down within a set timeframe—usually 90 days—under controlled composting conditions, maintaining stability without leaving harmful residues. PLA can be compostable, provided that it is disposed of in the right facilities designed for such a purpose. It is important to note that not all PLA products guarantee compostability, especially those that are mixed with non-biodegradable materials or treated with various additives.
"Understanding the nuances between biodegradability and compostability is essential for effective waste management and environmental sustainability."
Comparison with Conventional Plastics
When compared to conventional plastics, PLA shows several notable differences, particularly in terms of degradation rates and environmental impacts. Conventional plastics, such as polyethylene and polypropylene, are derived from fossil fuels. These materials can take hundreds of years to degrade, if not longer, contributing to significant environmental pollution and accumulation in landfills.
In contrast, PLA has a much shorter degradation timeline. Although it often needs specific conditions to biograde effectively, the environmental footprint is considerably lower when processed correctly. Additionally, PLA produces fewer toxic byproducts compared to conventional plastics during its degradation process, further enhancing its profile as a more sustainable option.
The advantages of PLA can outweigh those of traditional plastics in certain applications. However, some challenges remain, including the requirement for appropriate industrial composting facilities and the chance of contamination when mixed with other waste streams. Understanding these distinctions is crucial for both consumers and industry stakeholders in making informed choices regarding sustainable materials.
As the world continues to grapple with the urgent need to reduce plastic waste, PLA offers a promising alternative. Its biodegradability and potential for composting can contribute to a reduction in pollution, aligning well with current sustainability goals.
Mechanisms of PLA Biodegradation
The mechanisms of PLA biodegradation are crucial for understanding its potential as a sustainable material. This section will explore the chemical and biological processes that allow PLA to break down into simpler compounds. Understanding these mechanisms provides insight into how PLA can be effectively utilized in various applications while minimizing environmental impact.
Chemolysis and Hydrolysis Processes
Chemolysis and hydrolysis play significant roles in the degradation of PLA. Chemolysis refers to the chemical breakdown of PLA under certain conditions, such as exposure to high temperatures or specific catalysts. Hydrolysis, on the other hand, involves the reaction of PLA with water, leading to the production of lactic acid, the monomer from which PLA is derived. This process typically occurs under moist conditions, making it a key factor in environments like compost heaps or marine settings.
These processes are essential because they underscore the material's ability to break down without leaving behind significant toxic residues. The rate at which these reactions occur can depend on various factors such as temperature, humidity, and the presence of catalysts. Understanding these parameters is vital for optimizing conditions that enhance PLA degradation, potentially leading to more eco-friendly practices in product development.
Microbial Degradation Mechanisms
Microbial degradation is another important aspect of PLA biodegradation. Microbes, including bacteria and fungi, play a pivotal role in the breakdown of PLA materials, contributing significantly to the decomposition process.
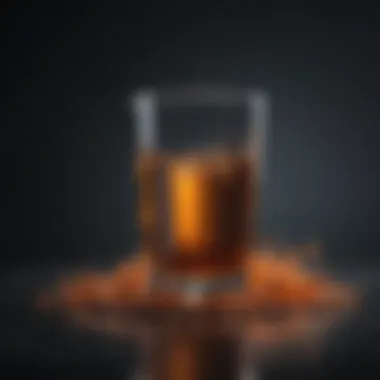
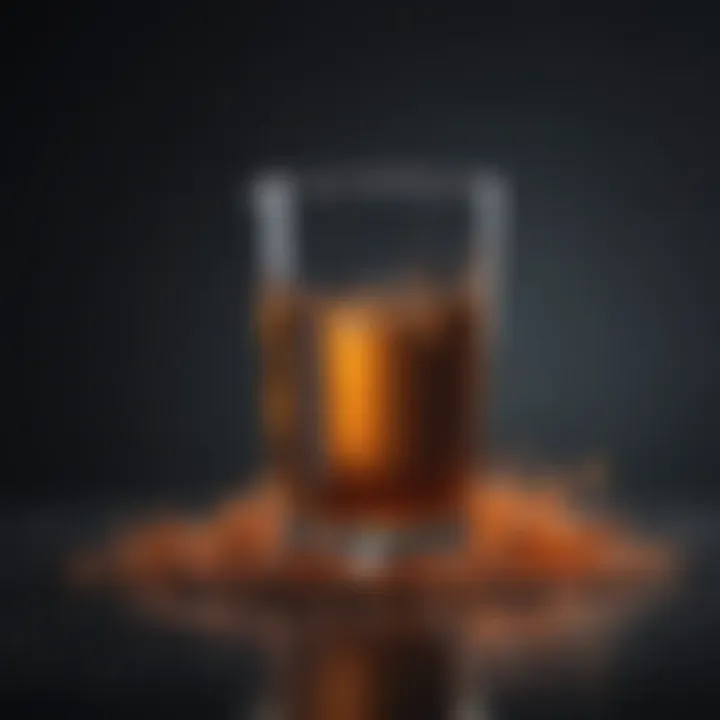
Role of Bacteria in PLA Breakdown
Bacteria are the primary agents in the microbial degradation of PLA. Their ability to secrete specific enzymes allows them to break the polymeric structure of PLA into smaller, more manageable fragments. A key characteristic of bacteria in this context is their adaptability. Many bacteria can thrive in varying environmental conditions, which enhances their effectiveness in degrading PLA in diverse ecosystems.
The unique feature of bacteria in this degradation process is their rapid reproduction and metabolic processes. These features enable them to substantially accelerate the breakdown of PLA materials when compared to abiotic processes alone. While bacterial degradation can be highly efficient, it may also produce byproducts that require further assessment regarding their environmental impact.
Fungi and Their Contribution
Fungi also contribute significantly to PLA degradation, complementing the action of bacteria. Fungi secrete a wider variety of enzymes than bacteria, allowing them to break down more complex structures, including those found in PLA composites. This characteristic makes fungi particularly effective in degrading PLA in environments rich in organic matter.
The unique advantage of fungal involvement lies in their capability to utilize a variety of substrates for growth, including decomposed PLA. This ability can lead to more efficient biodegradation under specific environmental conditions. However, the slow growth rate of fungi compared to bacteria may limit their rapid effectiveness in certain situations.
In summary, the mechanisms of PLA biodegradation reveal a complex interaction between chemical and biological processes, highlighting the importance of both chemolysis and microbial activity. Understanding these mechanisms will aid in the development of PLA-based products designed for better environmental compatibility.
Overall, the contribution of both bacteria and fungi to the degradation of PLA illustrates the diverse approaches nature employs to recycle materials and promote sustainability. By comprehensively understanding these mechanisms, researchers can better harness their potential for enhancing the biodegradability of PLA in future applications.
Factors Influencing PLA Biodegradation
Understanding the factors that influence PLA biodegradation is crucial for advancing its use as a sustainable material. This section will thoroughly analyze how specific elements, including temperature, humidity, pH levels, and oxygen availability, affect the biodegradation process of polylactic acid. By delving into these aspects, we can better comprehend the conditions that optimize or inhibit the breakdown of PLA, which is essential for its effective deployment in various applications.
Influence of Temperature and Humidity
Temperature plays a significant role in the enzymatic processes involved in PLA biodegradation. Higher temperatures can facilitate a quicker breakdown due to enhanced microbial activity. However, it is essential to note that excessively high temperatures may denature the enzymes involved, thereby hindering degradation. Conversely, lower temperatures might slow down microbial processes, leading to prolonged persistence of PLA in the environment.
Humidity is equally important as it directly affects moisture levels in the environment where PLA is situated. Sufficient moisture enables microbes to thrive and degrade PLA effectively. When humidity is too low, microbial growth can be limited, and the degradation slows. Both high temperature and adequate humidity create an optimal environment for biodegradation, demonstrating the interdependence of these factors.
pH Levels and Their Effects
The pH of the surrounding environment significantly impacts the biodegradation of PLA. Most microorganisms that break down biodegradable plastics prefer a neutral to slightly acidic pH range for optimal activity. If the environment is too acidic or too alkaline, it may impair microbial function, hindering the effectiveness of PLA breakdown.
Research indicates that maintaining a pH between 6 to 7.5 tends to foster a conducive environment for microbial growth and activity, effectively enhancing biodegradation rates. Additionally, variances in pH can also influence the physical properties of PLA, affecting its solubility and susceptibility to microbial attack.
Oxygen Availability
Oxygen availability is a critical factor in the biodegradation of PLA. Aerobic processes, where oxygen is present, generally promote faster degradation compared to anaerobic processes, which are oxygen-deficient. In aerobic conditions, bacteria can utilize oxygen to metabolize PLA, resulting in quicker breakdown. Furthermore, the byproducts of aerobic degradation are often less harmful than those that arise during anaerobic conditions, highlighting the environmental importance of adequate oxygen levels.
On the other hand, in environments with low oxygen, PLA may still degrade, but the process could be significantly slower. This situation leads to the production of methane and other byproducts that may pose environmental risks. Therefore, for effective biodegradation of PLA, ensuring access to oxygen in the waste management processes is vital.
In summary, the factors influencing PLA biodegradation are interconnected and complex. Understanding the interplay of temperature, humidity, pH, and oxygen levels is essential for developing strategies to optimize the decomposition of PLA in various applications. By focusing on these factors, industries can enhance their sustainability efforts and contribute to reducing plastic pollution effectively.
Applications of PLA in Various Industries
The utilization of Polylactic Acid (PLA) spans various sectors, driven by its biodegradable nature and potential to reduce plastic waste. Understanding these applications is essential as it underscores the role of PLA in promoting sustainability and addressing environmental issues associated with conventional plastics. Each industry employs PLA in ways that showcase its unique properties while also highlighting the benefits and considerations of using this material.
Agriculture and Horticulture
In agriculture, PLA is gaining popularity for its use in biodegradable mulch films and planting pots. These applications provide significant advantages over traditional plastic materials, as they degrade naturally in soil, minimizing pollution. The biodegradable mulch films help to suppress weeds, retain moisture, and enhance soil temperature. As a result, they contribute to improved crop yields and faster growth cycles. Furthermore, after their intended use, these films decompose, enriching the soil with organic matter.
Benefits of PLA in agriculture include:
- Reduction of plastic waste in fields
- Enhanced soil health through natural decomposition
- Lower environmental impact during cultivation
However, there are challenges to consider. PLA's biodegradation rate is highly dependent on environmental factors such as temperature and humidity. Therefore, understanding how PLA behaves in different climates is vital to its successful implementation. Moreover, the cost may be higher than that of conventional plastics, which could affect widespread adoption.
Packaging Solutions
The packaging industry is one of the largest consumers of PLA, primarily due to its eco-friendly properties. PLA-based packaging has emerged as a popular alternative to traditional plastics in food containers, bags, and other packaging materials. With increasing consumer demand for sustainable options, businesses are turning to PLA for their packaging needs.
Advantages of using PLA in packaging include:
- Compostability, leading to reduced landfill waste
- Lower carbon footprint compared to petroleum-based plastics
- Versatility in design and functionality
PLA provides excellent barrier properties, preserving the freshness of products while being environmentally friendly. Nevertheless, challenges arise in the form of durability and performance at various temperatures. For instance, PLA may not withstand high heat, which can limit applications in some food packaging scenarios. Therefore, producers must navigate these limitations while also considering consumer expectations regarding product quality and shelf life.
Medical Applications
In the medical field, PLA is notable for its application in surgical sutures, implants, and drug delivery systems. Its biocompatibility and biodegradability make PLA a favorable choice for short-term medical devices. The degradation of PLA in the body can coincide with tissue regeneration, providing a natural healing process for patients.
Key applications of PLA in medicine include:
- Surgical sutures that dissolve after healing, eliminating the need for removal
- Implants that gradually degrade, reducing the risk of complications associated with permanent devices
- Drug delivery systems that release medication over time, improving patient adherence and outcomes
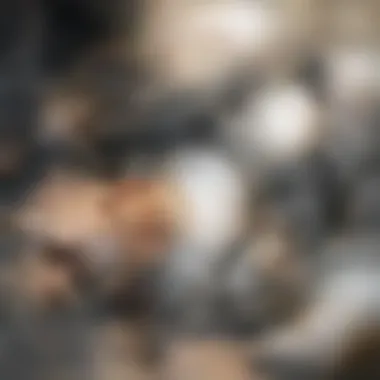
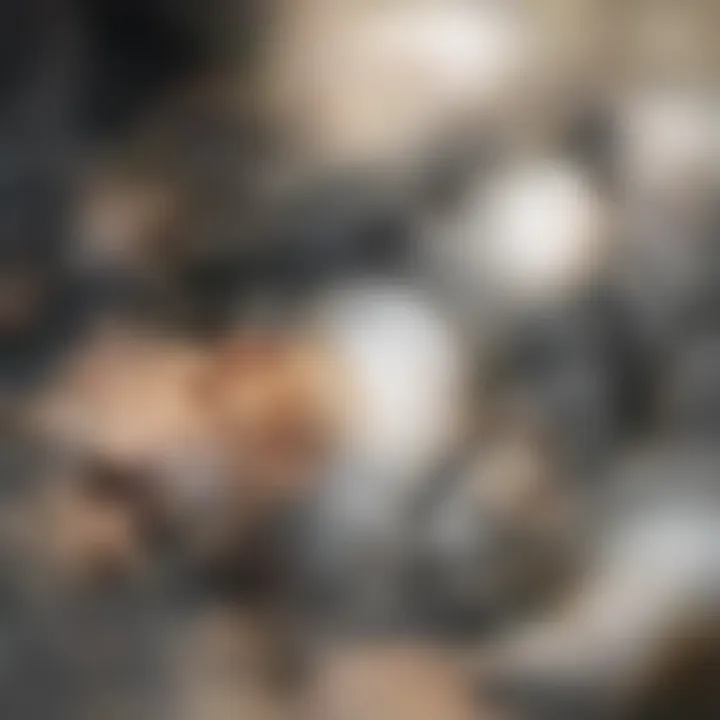
While the advantages are substantial, considerations for PLA in medical applications include its breakdown rates and the resulting byproducts. Understanding how PLA degrades over time in the body remains a crucial aspect for researchers and developers. This understanding is essential not only for the efficacy of medical devices but also for ensuring safety in patient treatment.
"The growing embrace of PLA across diverse industries highlights its promise as a sustainable alternative in combatting plastic pollution."
Overall, the applications of PLA serve to illustrate its potential as a multifaceted material with wide-ranging benefits. As industries continue to explore its capabilities, ongoing research will be crucial to addressing the challenges and enhancing its performance in various environments.
Challenges in PLA Biodegradation
Understanding the challenges in PLA biodegradation is essential for assessing its viability as a sustainable alternative to conventional plastics. While polylactic acid is derived from renewable resources and offers many benefits, the path to its widespread implementation is fraught with obstacles. This section delves into the critical elements that affect the efficiency and effectiveness of PLA biodegradation, focusing particularly on industrial scalability and the issues of toxic byproducts.
Industrial Scalability of Biodegradation Processes
The potential for PLA to serve as an alternative to traditional plastics heavily relies on the scalability of biodegradation processes. One fundamental issue is the difference between laboratory conditions and real-world environments. At a lab scale, researchers can effectively demonstrate how PLA breaks down in ideal conditions. However, achieving similar results on an industrial scale remains challenging. The complexities arise in replicating the necessary environmental factors.
- Microbial Culture Management: Cultivating the right strains of bacteria and fungi that effectively degrade PLA requires specific conditions. The selection of microbial communities must be tailored to different environments, and this variation complicates the scaling process.
- Process Optimization: Industrial facilities need to optimize the conditions under which biodegradation occurs, such as temperature, humidity, and oxygen levels. These parameters must be precisely regulated to ensure maximum breakdown rates, which can be difficult to achieve consistently.
- Economic Viability: Cost considerations cannot be overlooked. The expenses associated with waste collection, transportation, and treatment processes must be manageable for widespread acceptance. If the costs outweigh the benefits, industries may be slow to adapt.
Addressing these factors will require investments in research and technology to answer the scalability questions. Significant advancements here could enable more effective utilization of PLA in industries seeking sustainable practices.
Toxic Byproducts and Environmental Concerns
While PLA is biodegradable, the breakdown process does not come without concerns. The formation of toxic byproducts during degradation poses a risk to ecosystems. Understanding these potential byproducts is vital for evaluating PLA’s sustainability. Several factors contribute to this concern:
- Anaerobic Decomposition: In environments lacking oxygen, PLA may decompose into potentially harmful substances, which could adversely affect soil and water quality.
- Chemical Composition: As PLA degrades, it can produce lactic acid and other intermediates. Although lactic acid is less harmful than many pollutants, any accumulation in specific environments could lead to unintended ecological shifts.
- Long-term Impacts: The long-term effects of PLA breakdown products in various environments are not fully understood. Regulatory bodies will need more extensive studies to evaluate whether these byproducts could become a concern over extended periods.
These elements necessitate caution and thorough research. Balancing the benefits of using PLA against the potential environmental risks requires careful analysis and proactive measures.
"Understanding the complex interplay between industrial factors and environmental impacts is crucial for the evolution of PLA biodegradation processes."
In summary, while PLA presents various opportunities, overcoming the challenges of industrial scalability and managing the risk of toxic byproducts is critical. Addressing these issues will help ensure PLA's position as a reliable and sustainable alternative in the broader landscape of plastic materials.
Future Perspectives on PLA and Sustainability
The discussion around polylactic acid (PLA) biodegradation cannot be complete without considering future perspectives and sustainability. As the world increasingly grapples with plastic pollution, the potential of PLA as a renewable alternative becomes crucial. Examining the trends and innovations in PLA technology offers insights into how it can solidify its role in sustainable practices. Key elements to consider include the technological advancements, regulatory frameworks, and consumer attitudes towards biodegradable products. Each of these aspects will shape the trajectory of PLA in the coming years.
Innovations in PLA Technology
Innovations in PLA technology are vital to enhancing its biodegradability and expanding its applications. Recent research focuses on improving the performance characteristics of PLA for various industries.
- Enhanced Formulations: The development of PLA blends with other biodegradable polymers increases the overall strength and functionality, making them suitable for more demanding applications.
- Nanocomposites: Incorporating nanomaterials has shown promise in improving thermal and mechanical properties of PLA. This allows it to compete more effectively against conventional plastics in markets like packaging and automotive.
- Tailored Processing Techniques: New methods, such as extrusion with supercritical fluids, are being explored to optimize PLA’s physical structure, improving its degradation profile and making it more appealing to manufacturers.
These innovations not only advance PLA’s competitiveness but also underscore its potential to serve as an eco-friendly alternative to traditional plastics.
Regulatory Framework and Policy Implications
The role of policy in the propagation of PLA technology and its management cannot be underestimated. As governments and organizations implement stricter regulations on plastic usage, the regulatory framework will heavily influence the market dynamics of PLA.
- Standards and Certification: Establishing comprehensive certification processes for biodegradable materials can create a level playing field, ensuring that claims about biodegradability are valid and reliable.
- Incentives for Sustainable Options: Policies that encourage the use of renewably sourced materials, such as tax reductions or subsidies, can expedite the adoption of PLA in industries that are traditionally reliant on petrochemical plastics.
- International Collaboration: Future efforts must also consider international standards to fortify the global market for PLA, promoting a more unified approach to tackling plastic pollution.
Understanding the regulatory landscape will allow researchers and consumers to navigate and influence the implementation of PLA in a responsible manner.
Role of Consumer Awareness
Consumer awareness plays a critical role in driving demand for sustainable alternatives like PLA. Education on the benefits and proper disposal methods of biodegradable products can lead to more informed choices.
- Public Campaigns: Initiatives to raise awareness about the environmental impacts of plastic pollution can encourage consumers to choose PLA products over conventional options.
- Transparency in Labeling: Clear and concise labeling on products regarding their biodegradability and compostability can build trust with consumers, making them more likely to support companies that prioritize sustainability.
- Engagement via Social Media: Platforms such as Reddit and Facebook can serve as tools for engaging communities around sustainable practices, influencing broader consumer behavior.
End and Implications for Research
Understanding polylactic acid (PLA) biodegradation is essential to advance the circular economy and reduce environmental impacts. Reducing plastic pollution is increasingly important, and PLA presents a viable alternative to conventional plastics. This section synthesizes key findings from the article and emphasizes implications for future research initiatives.
The biodegradation of PLA involves complex interactions between environmental factors and microbial processes. These interactions determine the rate and efficiency of degradation. For instance, temperature, humidity, and pH have significant effects. Optimizing these conditions can enhance biodegradability and make industrial processes more efficient. Understanding these parameters helps industries adopt PLA more effectively in their operations, thus promoting sustainability.
Moreover, the challenges associated with PLA biodegradation, such as potential toxic byproducts, cannot be overlooked. Addressing these challenges is crucial for convincing industry stakeholders and consumers of PLA’s safety and efficiency.
"The transition from conventional plastics to biodegradable alternatives like PLA is an urgent priority for environmental sustainability."
Summary of Findings
This article has explored the multifaceted aspects of PLA biodegradation. Key insights include:
- ** PLA as a Sustainable Alternative:** Derived from renewable resources, PLA has significant advantages over traditional fossil fuel-based plastics in terms of biodegradability and environmental impact.
- ** Mechanisms of Degradation:** Various mechanisms, such as chemolysis and microbial degradation, drive the breakdown of PLA in different environments. Understanding these mechanisms is critical to enhancing PLA's effectiveness in real-world applications.
- ** Environmental Influences:** Factors like temperature, humidity, and pH greatly influence the degradation rate of PLA, underscoring the need for specific conditions to optimize biodegradability.
- ** Industrial Applications:** PLA is effectively utilized across several sectors, including agriculture, packaging, and medicine, demonstrating its versatility as a biopolymer.
- ** Challenges Identified:** Key challenges exist including scalability of industrial processes for PLA degradation and the potential for toxic byproducts which require scrutiny.
Calls for Future Research Directions
Future research directions could significantly enhance the scientific understanding and practical applications of PLA. Important areas include:
- Enhanced Formulations: Developing advanced PLA formulations that tailor biodegradation rates suitable for specific applications could increase the material’s usability in various industries.
- Microbial Interactions: Further studies on the role of specific microbes in the degradation of PLA could yield insights for improving biodegradability in diverse environments.
- Environmental Assessment: Conducting comprehensive environmental impact assessments for PLA in comparison to other materials could strengthen its case as a leading sustainable alternative.
- Policy Development: Collaborating with policymakers to establish guidelines for PLA usage and waste management practices will ensure that PLA norms are included in regulatory frameworks.
- Consumer Education: Programs aimed at increasing consumer awareness about PLA's benefits and proper disposal methods can drive adoption and support sustainability efforts.
In summary, while PLA presents a promising avenue for addressing plastic pollution, systematic research is necessary to fully realize its potential and overcome existing challenges.