Exploring Steam Turbine Cogeneration Systems
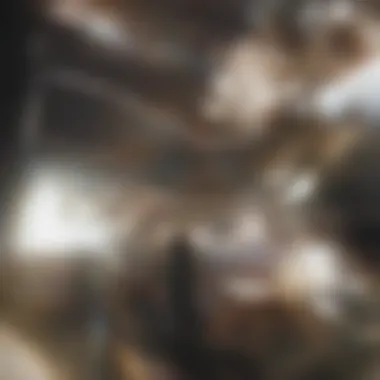
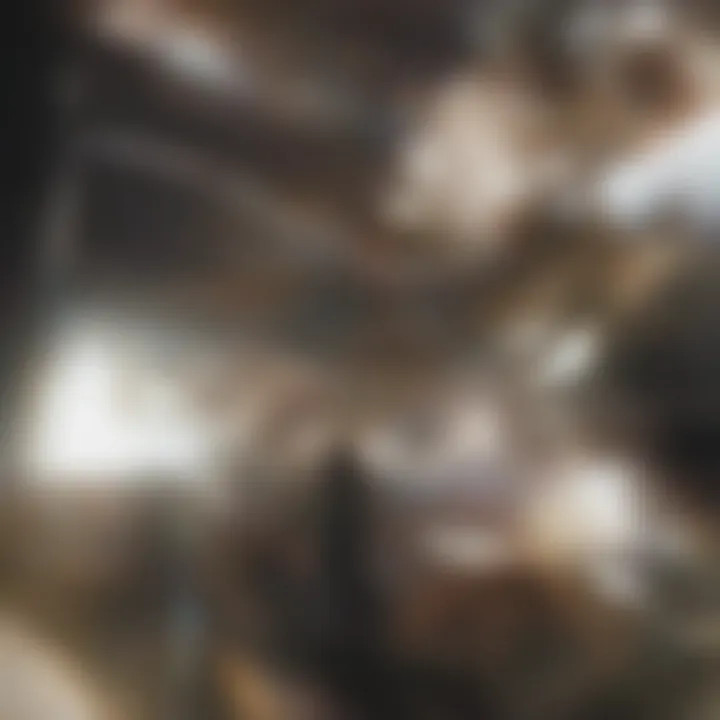
Intro
Steam turbine cogeneration systems serve an essential role in efficient energy production. These systems generate both electricity and useful thermal energy from the same energy source, significantly enhancing overall efficiency. The technology is widely applied across various industries, including manufacturing and utility sectors. Understanding the principles behind these systems helps professionals optimize energy use, reduce costs, and minimize environmental impact.
Key Findings
Major Results
Throughout this examination of steam turbine cogeneration systems, several key findings emerge:
- Efficiency Gains: The cogeneration process can achieve efficiencies of 60% or more, compared to standalone generation methods, which typically do not exceed 40%.
- Environmental Benefits: By utilizing waste heat that would otherwise be lost, cogeneration systems effectively lower greenhouse gas emissions, contributing to sustainability goals.
- Flexibility of Applications: These systems are applicable in various contexts, ranging from industrial processes to district heating systems.
Discussion of Findings
The advantages noted above underline why steam turbine cogeneration is gaining traction. With dwindling resources and rising costs of traditional energy, industries are driven to adopt more holistic approaches to energy management. The findings illustrate a clear trend: incorporating cogeneration not only improves efficiency but also aligns with environmental standards and regulations.
Methodology
Research Design
The exploration of steam turbine cogeneration systems involved a detailed analysis of existing literature and case studies. Additionally, interviews with industry professionals provided practical insights into operational challenges and solutions. By synthesizing academic research with real-world experiences, a comprehensive understanding of these systems was developed.
Data Collection Methods
Data was collected from multiple sources:
- Academic journals focusing on energy efficiency and cogeneration technologies.
- Industry reports detailing operational metrics and case studies of cogeneration implementations.
- Interviews with engineers and operations managers in sectors employing steam turbine cogeneration.
Aggregating information from these varied sources ensured that the exploration reflects both theoretical underpinnings and practical applications. This dual perspective promotes a more robust understanding of steam turbine cogeneration systems.
Preamble to Cogeneration Systems
The topic of cogeneration systems is crucial to understanding how energy efficiency can be maximized in industrial processes. Cogeneration, often referred to as combined heat and power (CHP), has the potential to significantly enhance energy utilization by capturing and making use of heat that would otherwise go to waste. This process not only optimizes fuel use but also reduces overall emissions, thus contributing positively to environmental sustainability.
Cogeneration systems operate on a simple yet effective principle: they simultaneously produce electricity and useful heat from the same energy source. The integration of these two processes allows facilities to achieve high levels of efficiency, often exceeding 80% in terms of energy recovered. This is a stark contrast to traditional methods where electricity and heat are generated separately, leading to higher fuel consumption and greater emissions.
The importance of cogeneration is further highlighted by its versatility. It can be applied in various sectors, including industrial production, district heating, and even in commercial buildings. With these applications, cogeneration systems can meet local energy demands while providing environmental and economic benefits. In this context, understanding both the technological aspects and the operational principles of steam turbine cogeneration systems enriches the broader perspective on energy conservation and management.
Definition of Cogeneration
Cogeneration refers to the simultaneous generation of electricity and useful thermal energy from a single fuel source. This process allows for more effective energy use compared to conventional energy generation methods. In cogeneration systems, the waste heat produced during electricity generation is used to provide heating or hot water. This results in a more efficient energy conversion process and can lead to lower operational costs.
Cogeneration can occur using various types of fuels, including natural gas, biomass, and coal. The captured heat can be utilized for heating processes, space heating, or even for driving absorption chillers, which produce cooling. The flexibility of cogeneration systems makes them a valuable solution in transition towards a more sustainable energy future.
Historical Development of Cogeneration Technologies
The concept of cogeneration has been around for over a century. Early examples can be traced back to the late 19th century when steam engines were commonly used to generate both electricity and heat for industrial applications. The development of steam turbine technology in the early 20th century marked a significant evolution in cogeneration systems, allowing for more efficient heat and power production.
Throughout the decades, advancements in technology, materials, and system designs have further improved the efficiency and reliability of cogeneration systems. The oil crises in the 1970s intensified the interest in energy conservation, leading to increased adoption of cogeneration systems across various industries. Today, innovations such as combined cycle gas turbine systems and heat recovery technologies continuing to push forward the capabilities of cogeneration.
Overview of Steam Turbines
Steam turbines play a crucial role in cogeneration systems, serving as the engine that transforms thermal energy into mechanical energy. Understanding steam turbines is fundamental to grasping the operational efficiency and overall functionality of cogeneration setups. By utilizing steam as the primary working fluid, steam turbines can not only generate electricity but also provide useful heat, which is essential in various industrial processes.
The significance of steam turbines in the context of cogeneration cannot be overstated. Their design allows for high efficiency in power generation, with efficiency levels often exceeding those of other types of turbines. This is particularly beneficial in applications where both electric power and steam are needed, reducing waste and maximizing resource utilization.
Furthermore, steam turbines contribute to the overall sustainability of energy systems. They facilitate the integration of renewable energy sources, thus playing a vital part in reducing the carbon footprint of industrial operations. When evaluated alongside traditional power generation methods, steam turbines can help organizations lower greenhouse gas emissions and optimize energy consumption.
Basic Working Principle
The basic working principle of steam turbines relies on the thermodynamic properties of steam. Steam is generated in a boiler where water is heated under pressure. This steam then expands through the turbine, causing the blades to rotate. The rotational energy is converted to mechanical energy, which can either be used to drive a generator for electricity or to perform mechanical work in various industrial applications.
The key here is the conversion of thermal energy in the steam into kinetic energy. The efficiency of this conversion is influenced by several factors, including the temperature and pressure of the steam and the design of the turbine itself.
Types of Steam Turbines
Understanding the different types of steam turbines is essential for selecting the right technology for a specific cogeneration application. There are various configurations of steam turbines, with each type having unique characteristics and functionalities.
Impulse Turbines
Impulse turbines operate based on changing the momentum of steam jets. When steam is directed at the blades, it imparts kinetic energy, producing rotation. A key characteristic of impulse turbines is their simplicity of design and construction. They are relatively easy to maintain, making them a popular choice in many industrial applications.
The unique feature of impulse turbines is their ability to function effectively over a wide range of operating conditions. This flexibility is beneficial for facilities with variable energy demands. However, impulse turbines may not achieve the same efficiency levels as some other designs under specific circumstances.
Reaction Turbines
Reaction turbines differ fundamentally by utilizing the pressure drop across the turbine blades. As steam flows through the turbine, drop in pressure imparts energy to the blades. A significant characteristic of reaction turbines is their compact design, allowing for a greater amount of output from a smaller physical footprint.
The crucial advantage of reaction turbines is their higher efficiency, especially at lower steam pressures. This characteristic can make them an attractive option when aiming to optimize overall energy output in cogeneration systems. However, their complexity in design can make them more challenging to maintain compared to impulse turbines.
Condensing and Non-Condensing Types
Condensing steam turbines return unused steam to the boiler in a condensed form, maximizing the energy extracted from the thermal cycle. They are typically used in applications where electricity generation is prioritized, and excess heat can be reclaimed. The significant advantage here is higher efficiency in energy extraction.
In contrast, non-condensing turbines release steam at lower pressures, directing it for heating purposes in systems such as district heating. This type of turbine is advantageous for settings where both heat and electricity are needed, making them relevant in many industrial scenarios.
The choice between condensing and non-condensing turbines relies on the specific operational goals of the cogeneration system. Each type brings a unique set of benefits and trade-offs that cater to different operational needs.
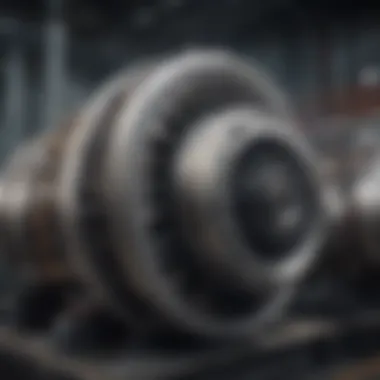
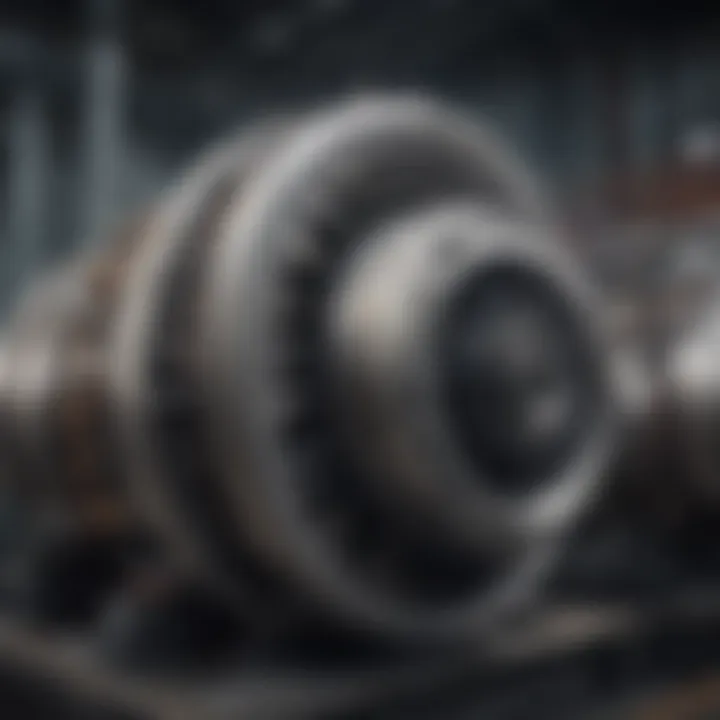
"Selecting the right type of steam turbine is crucial for the effectiveness and efficiency of cogeneration systems."
Understanding these types enhances decision-making processes in designing and implementing effective cogeneration solutions.
Components of Steam Turbine Cogeneration Systems
The functionality and efficiency of steam turbine cogeneration systems are deeply reliant on their main components. Understanding these elements enhances one’s grasp of how cogeneration achieves both heat and power generation simultaneously. These systems must be thoughtfully designed, integrating each component effectively to maximize overall performance.
Steam Generation System
The steam generation system is the cornerstone of cogeneration technology. It usually consists of a boiler that generates steam through combustion of fuel such as natural gas, biomass, or coal. The type of fuel determines the system's efficiency and emissions profile.
- Types of Boilers: The most common types include fire-tube and water-tube boilers, each with its own advantages regarding heat excess or steam output.
- Pressure and Temperature: Operating pressure and temperature must be carefully calibrated to optimize steam quality. Superheated steam increases turbine efficiency but requires advanced materials and design.
A well-designed steam generation system enhances thermal efficiency by reducing waste heat and improving fuel utilization.
Turbine and Generator Integration
The relationship between the turbine and generator is crucial. The turbine converts thermal energy from steam into mechanical energy, which is then turned into electrical energy by the generator. This integration demands careful alignment and optimization to ensure energy is not wasted.
- Types of Turbines: Impulse turbines and reaction turbines serve different functional needs; impulse turbines are typically better for high pressure while reaction works well under lower conditions.
- Control Systems: Advanced control systems are essential for real-time monitoring of performance, adjusting flow rates, and managing system responses under various operational conditions.
"The turbine and generator must work in perfect harmony to convert the maximum amount of thermal energy into electrical energy."
Heat Recovery Systems
In cogeneration systems, the heat recovery system serves to capture and utilize waste heat from the turbine exhaust. This process significantly improves the overall efficiency of the system.
- Heat Exchangers: These devices transfer excess heat to water, providing useful thermal energy for space heating or industrial processes.
- Combined Heat and Power (CHP): Incorporating a CHP system increases energy output while maximizing fuel efficiency. This often leads to lower operating costs and reduced emissions.
Understanding heat recovery not only enhances system efficiency but also promotes sustainable practices by reducing environmental impact.
In summary, the components of steam turbine cogeneration systems are interlinked. Each element, from steam generation to heat recovery, works synergistically to provide a robust solution for energy needs, contributing to operational effectiveness and sustainability.
Operational Principles of Steam Turbine Cogeneration
Understanding the operational principles of steam turbine cogeneration is crucial in comprehending how these systems function effectively in various applications. These principles form the backbone of both the design and operational aspects of cogeneration setups. They comprise the thermodynamic cycle details and performance metrics essential for optimizing system efficiency.
Cogeneration is appealing because it maximizes energy output from fuel. By using steam turbines in these systems, facilities can generate electricity while capturing waste heat for heating or industrial processes. This dual energy output leads to significant energy savings and reduced environmental impact.
Thermodynamic Cycle Analysis
Thermodynamic cycles are foundational to analyzing steam turbine cogeneration systems. The most common cycle used is the Rankine cycle, which consists of four significant processes: heating, vaporization, expansion, and condensation.
- Heating: Water is heated in a boiler, turning it into steam. This process typically occurs at high pressure, increasing the steam's potential energy.
- Vaporization: The heated water becomes steam. This is where the bulk of energy transformation takes place.
- Expansion: The steam expands through the turbine, converting thermal energy into mechanical energy, generating electricity in the process.
- Condensation: After passing through the turbine, the steam is cooled and condensed back into water, cycling back to the boiler.
Understanding these processes aids in identifying inefficiencies and enhancing system performance.
Efficiency and Performance Metrics
Evaluating the efficiency and performance of cogeneration systems involves analyzing two fundamental thermodynamic laws: the First Law and the Second Law of Thermodynamics. Both provide important insights into energy transfer and system optimization.
First Law of Thermodynamics
The First Law of Thermodynamics states that energy cannot be created or destroyed, only transformed from one form to another. In the context of cogeneration systems, this law helps quantify the energy inputs and outputs of the system.
- Key Characteristic: This principle highlights energy balance in a closed system, essential in evaluating the overall efficiency of steam turbines.
- Benefits: By adhering to this law, engineers can design systems that effectively utilize available energy, minimizing waste.
- Unique Feature: The material and energy efficiency depend heavily on proper integration of components, such as the boiler and turbine, ensuring high energy conversion rates.
Second Law of Thermodynamics
The Second Law addresses the direction of energy transfer, emphasizing that energy systems tend to move towards increased entropy. In cogeneration systems, it guides the understanding of irreversible processes like heat loss and performance limits.
- Key Characteristic: It indicates that not all input energy can be transformed into useful work, highlighting the importance of waste heat recovery.
- Benefits: Incorporating this principle reinforces the need for effective heat recovery systems, leading to better overall performance.
- Unique Feature: Its implications are critical for system design. Integrating a heat recovery steam generator (HRSG) enhances the overall thermal efficiency by capturing waste heat.
Understanding these operational principles enables engineers to design cogeneration systems that are not only efficient but also economically viable. By focusing on energy optimization, facilities can balance operational costs and environmental impacts.
Design Considerations for Cogeneration Systems
Design considerations for cogeneration systems are pivotal for ensuring the efficiency and effectiveness of energy production. This section explores vital elements like sizing, integration with existing infrastructures, and compliance requirements. Each of these considerations holds significant weight in the operational success of cogeneration setups, impacting energy output, operational costs, and environmental sustainability.
Sizing and Capacity Planning
Proper sizing of cogeneration systems is crucial. If the system is under-sized, it may not meet energy demands, leading to inefficiencies and potential downtimes. Conversely, an over-sized system may incur unnecessary costs and resource wastage. The planning process involves systematic evaluations of energy needs, peak load conditions, and utilization factors. Attention to factors such as load duration curves can aid in accurately forecasting energy needs.
Furthermore, manufacturers like Siemens and General Electric recommend using sophisticated modeling tools. These tools assist in predicting operational performance over time. The goal is to create a design that balances initial investment with long-term returns, fostering a sustainable energy environment.
Integration with Existing Systems
Integrating cogeneration systems into existing infrastructures presents both challenges and opportunities. Successful integration requires an understanding of how new components will interact with the current system. Evaluating existing layouts helps identify potential modifications needed, ensuring a seamless blend of new technologies with legacy systems.
The alignment of operations in thermal and electrical systems can lead to enhanced overall efficiency. It may also necessitate adjustments to control systems to accommodate dual-output operations. Energy providers often rely on specialized software for effective integration, ensuring that both traditional and cogeneration sources work in harmony.
Regulatory and Compliance Issues
Compliance with regulations is another significant aspect of cogeneration system design. Regulations can vary widely by region but generally focus on emissions standards, safety protocols, and equipment specifications. Adhering to these regulations is not just a legal obligation but also speaks to the larger commitment to environmental stewardship.
Organizations often engage with local regulatory authorities early in the design process. This proactive approach can help identify potential hurdles and develop a pathway for compliance that aligns with operational goals. It is beneficial for designers to stay updated with evolving regulations, as these can influence equipment choice and operational parameters.
In sum, understanding and effectively implementing design considerations for cogeneration systems is essential. It requires a holistic view of sizing, integration, and regulatory compliance. This careful planning ultimately assures optimal performance and sustainability.
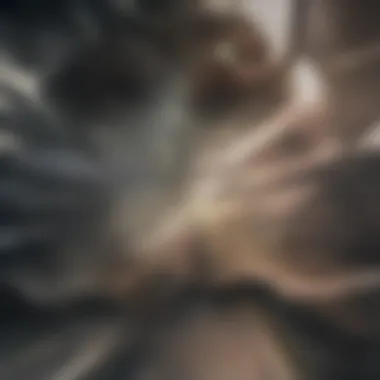
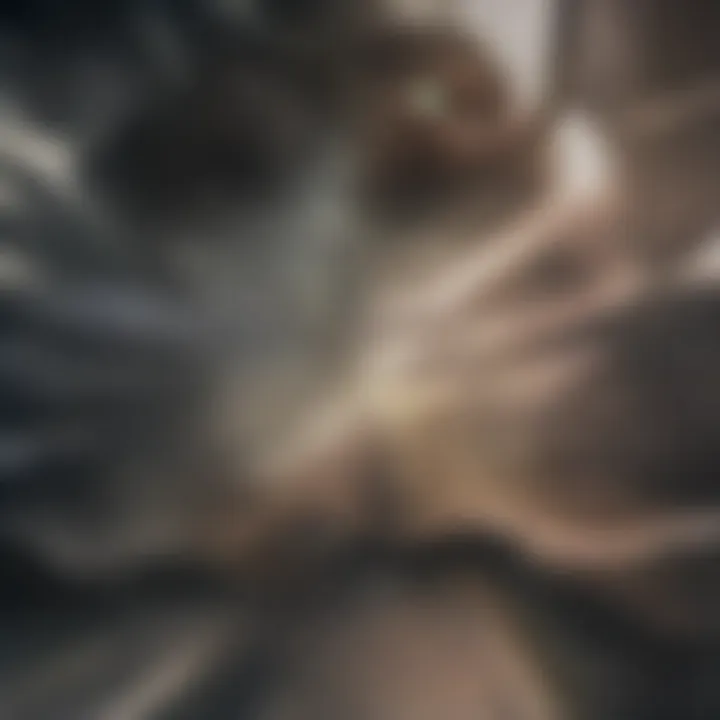
Economic Analysis of Cogeneration Systems
The economic analysis of cogeneration systems plays a pivotal role in their adoption and implementation within various sectors. In this section, we will explore the intricacies of financial evaluations associated with steam turbine cogeneration systems. Understanding these economic elements is essential for engineers, decision-makers, and investors looking to optimize energy production while minimizing costs. Cogeneration offers the potential for significant cost savings and efficiency benefits, which must be weighed carefully against investment and operational expenditures.
Cost-Benefit Analysis
Cost-benefit analysis is a fundamental aspect of evaluating cogeneration systems. This method compares the total expected costs against the anticipated benefits over a system’s lifetime. The primary costs to consider include:
- Initial Capital Investment: This encompasses the purchase of equipment, installation expenses, and any necessary infrastructure upgrades.
- Operational and Maintenance Costs: Ongoing expenses such as labor, repairs, and supplies must also be factored in.
- Fuel Costs: Since cogeneration systems rely heavily on fuel sources, fluctuations in fuel prices can significantly impact profitability.
On the benefit side, the advantages of cogeneration are clear:
- Reduced Energy Costs: Producing electricity and thermal energy simultaneously leads to lower overall energy expenses.
- Increased Efficiency: Cogeneration systems can achieve overall efficiencies exceeding 80% by utilizing waste heat, thus maximizing fuel use.
- Revenue Generation: Systems can often sell excess electricity back to the grid, creating an additional revenue stream.
Ultimately, conducting a thorough cost-benefit analysis informs decision-makers about the viability of cogeneration systems. It allows stakeholders to assess potential savings and returns on investment, thereby facilitating informed choices about system design and operation.
Financial Incentives and Subsidies
Governments and organizations often provide financial incentives to encourage the adoption of cogeneration technologies. These incentives significantly influence the economic landscape for potential investors. Key financial supports include:
- Tax Credits: Many regions offer tax reductions for investments in energy-efficient technologies, including cogeneration systems.
- Grants and Rebates: Some governments provide upfront financial assistance to offset initial capital costs, making projects more accessible.
- Low-Interest Loans: Financing options that carry lower interest rates can ease the financial burden on companies willing to invest in cogeneration systems.
These financial incentives can effectively improve the return on investment and make cogeneration systems more attractive alternatives to conventional energy solutions. Understanding the landscape of available subsidies aids stakeholders in identifying opportunities for cost reduction. By leveraging these financial tools alongside comprehensive cost-benefit analyses, companies can enhance the economic feasibility of steam turbine cogeneration projects.
"Incorporating financial incentives can turn a marginal investment into a highly attractive opportunity."
The synergy of cost-benefit analysis and financial incentives is vital to demonstrating the economic viability of steam turbine cogeneration. This intersection supports informed decision-making, enabling stakeholders to embrace advancements in technology while contributing to sustainability efforts.
Environmental Impact of Cogeneration
The environmental impact of cogeneration systems has become an essential consideration as global awareness of climate change and resource depletion increases. Steam turbine cogeneration systems provide a solution to produce both electrical power and useful thermal energy concurrently, which enhances overall efficiency. The reduction in fuel consumption and emissions benefits both the environment and the end-user economically. This section delves into the significant environmental aspects of cogeneration systems, specifically focusing on greenhouse gas emissions and sustainable energy practices.
Reduction of Greenhouse Gas Emissions
One major environmental advantage of steam turbine cogeneration systems is their capability to minimize greenhouse gas emissions. By using the same fuel source for multiple energy outputs, cogeneration helps to lower the overall carbon footprint compared to separate heat and power generation systems. The efficiency of cogeneration systems often exceeds 80%, which is substantially better than that of traditional power plants, which may only reach about 33% efficiency.
As the world grapples with increasing levels of carbon dioxide and other dangerous gases, cogeneration plays a role in several key ways.
- Less Fuel Consumption: Using a single fuel source for both electricity and heat decreases the total amount of fuel burned.
- Reduced Emissions: Fewer emissions are produced, corresponding to the reduced fuel consumption.
- Improved Performance: High efficiency translates to lower emissions per unit of energy produced, therefore contributing positively to environmental goals.
Research indicates that integrated systems can result in significant reductions in CO2 emissions. This shift not only supports regulations aimed at cutting greenhouse gases but also aligns with corporate social responsibility initiatives and sustainable development goals.
Sustainable Energy Practices
Sustainable energy practices are pivotal in today's energy landscape, and cogeneration systems contribute significantly to this movement. These systems utilize renewable or alternative sources of energy whenever feasible, such as biomass, biogas, and solar thermal energy, which can supplement the operational efficiency and reduce environmental harm.
Key sustainable practices associated with cogeneration include:
- Resource Utilization: Cogeneration optimizes the use of available resources, turning waste heat into usable energy, which is crucial for resource conservation.
- Energy Resilience: By diversifying energy sources, cogeneration enhances the reliability of energy supply in specific applications like district heating and industrial processes.
- Synergies with Renewable Energy: Integration with renewable sources reduces dependency on fossil fuels, thus promoting a cleaner energy ecosystem.
"Cogeneration stands out as a pivotal technology in progressing towards sustainable energy systems, as it significantly raises overall system efficiency while mitigating environmental impacts."
In summary, the environmental impact of cogeneration systems is significant. This technology not only contributes to reducing greenhouse gas emissions but also fosters robust sustainable energy practices, aligning with broader ecological goals. As industries and governments focus on sustainability, understanding these impacts is crucial for future energy strategies.
Applications of Steam Turbine Cogeneration Systems
Steam turbine cogeneration systems play a significant role within modern energy frameworks. Their ability to simultaneously produce electricity and useful heat has made them invaluable across various industries. In fact, cogeneration is often seen as a way to enhance energy efficiency while decreasing environmental impact. This section highlights three primary applications: industrial applications, district heating systems, and power generation applications.
Industrial Applications
The industrial sector is a primary beneficiary of steam turbine cogeneration systems. These systems enable industries such as chemicals, paper, and food processing to utilize their waste heat effectively. By capturing and reusing thermal energy, facilities can achieve significant reductions in fuel consumption.
Some specific benefits include:
- Cost Savings: Reduced energy costs from utilizing recovered waste heat.
- Increased Efficiency: Enhanced operational efficiency by minimizing energy waste.
- Environmental Benefits: Lower greenhouse gas emissions due to reduced fuel consumption.
These advantages position steam turbine cogeneration as a compelling solution for industries aiming to improve their energy management strategies. The integration of cogeneration systems allows firms to align with sustainability goals while achieving economic viability.
District Heating Systems
District heating systems are another key application of steam turbine cogeneration. These systems distribute heat generated from a central source to multiple buildings, effectively creating a network. The use of cogeneration in district heating enhances overall efficiency and reliability.
Key elements of this application include:
- Centralized Production: Producing heat and power in one facility reduces operational complexity.
- Flexibility: Systems can supply varying amounts of heat as demand fluctuates.
- Community Benefits: Helps provide energy security for communities by reducing dependence on fuel imports.
Overall, the cogeneration approach in district heating systems proves effective in not only meeting local energy demands efficiently but also in supporting local economies.
Power Generation Applications
Finally, steam turbine cogeneration systems are instrumental to power generation. These setups cater to both base-load and peak-load power generation needs. By integrating heat recovery, the cogeneration system becomes more efficient than traditional power plants.
This application presents several advantages:
- Higher Efficiency: Cogeneration can achieve overall energy efficiencies exceeding 80% by utilizing both electricity and heat.
- Diverse Fuel Availability: Power generation plants can operate on various fuels, including natural gas and biomass.
- Grid Stability: By providing additional power, cogeneration systems enhance overall grid resilience, especially during peak demand periods.
Recent Advancements in Cogeneration Technology
The realm of cogeneration technology is evolving rapidly, offering improved methods for energy efficiency and sustainable practices. The development of new technologies impacts both industrial applications and environmental sustainability. Recent advancements focus on innovative designs and integrations that enhance performance while reducing emissions. This section examines these key elements, illustrating the benefits and considerations of current trends in cogeneration technology.
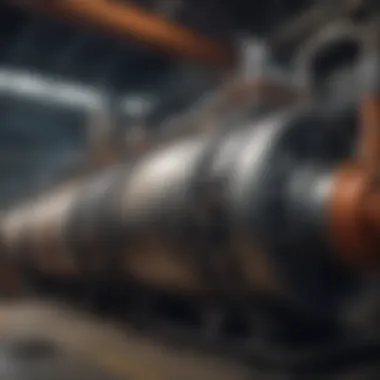
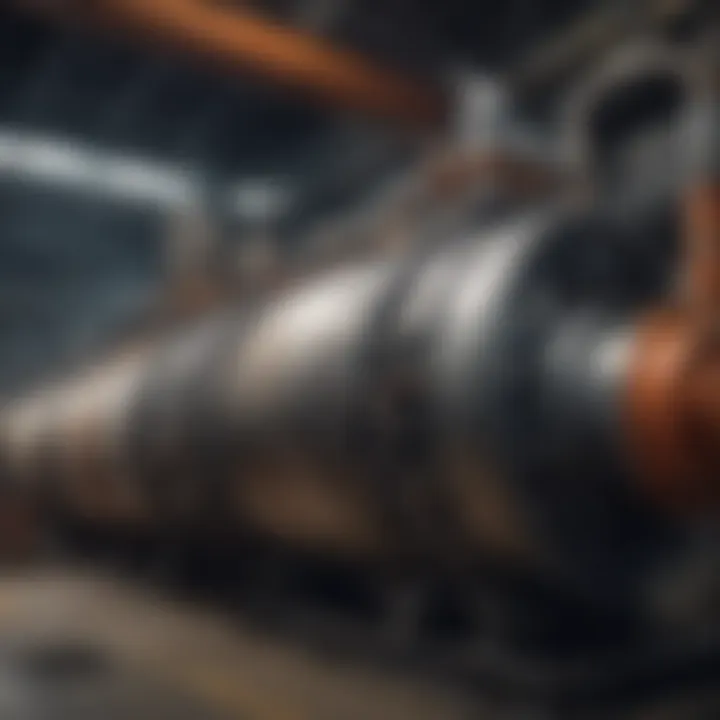
Innovative Steam Turbine Designs
Innovative steam turbine designs have emerged as a significant advancement in cogeneration systems. These designs are focused on enhancing the thermal efficiency of turbines, which is crucial for energy savings. Higher efficiency means more energy can be converted from steam, translating into less fuel consumption.
Enhancements in turbine design include the following:
- Variable Geometry Turbines: These allow for adjustments in turbine blades to optimize performance at various load conditions. This adaptability maximizes the energy extracted from steam under different operational circumstances.
- Advanced Materials: The use of new materials can improve heat resistance and durability. This leads to longer operational lifespans and reduced maintenance costs.
- Compact Turbine Configurations: Innovative layouts minimize the footprint of the machinery, making installations more flexible and reducing space requirements.
As industries strive for competitiveness, these advanced designs offer not just improved operational efficiencies but also lower operating costs over time. Further research continues to push boundaries in this area, suggesting future steam turbines could operate at even lower emissions and higher efficiency rates.
Integration with Renewable Energy Sources
The integration of steam turbine cogeneration systems with renewable energy sources signifies a pivotal step towards sustainable energy strategies. By combining traditional steam generation processes with renewable inputs, such as solar thermal energy or biomass, systems can transform the way energy is produced.
This combination presents several benefits:
- Enhanced Reliability: Using a mix of energy sources can provide a more stable supply. This is particularly relevant during fluctuating demands or variability in renewable energy supply.
- Reduced Greenhouse Gas Emissions: By utilizing cleaner energy sources, the overall carbon footprint of cogeneration systems can be significantly lowered. This integration allows industries to meet regulatory standards while pursuing sustainability goals.
- Increased Energy Resilience: Systems that incorporate renewables can adapt better to market fluctuations, offering cost advantages over time.
The ongoing trend towards integrating renewable sources into cogeneration systems reflects an alignment with global energy demands and environmental preservation. Additional innovations in energy storage technology will further optimize these integrations, ensuring reliable energy delivery even when renewable sources are non-operational.
The synergy between steam turbine cogeneration and renewable energies presents a forward-looking approach, addressing both economic and environmental imperatives in today’s energy landscape.
Challenges and Limitations
The Challenges and Limitations of steam turbine cogeneration systems play a vital role in understanding their broader implications and functionality. These systems promise enhanced efficiency and environmental benefits; however, certain obstacles can affect their successful implementation and financial sustainability. Recognizing these challenges is crucial for stakeholders aiming to optimize cogeneration systems in various settings.
On one hand, embracing steam turbine cogeneration systems can lead to significant energy savings and reduced carbon footprints. On the other hand, without addressing these challenges, potential benefits may not be fully realized.
Technical Challenges in System Implementation
Implementing steam turbine cogeneration systems involves various technical challenges, which must be carefully navigated. First, the complexity of the technology itself requires skilled personnel capable of managing and maintaining the systems. Engineers must have a solid understanding of thermodynamics and fluid mechanics. This knowledge is critical, as faults in design or operation can lead to inefficiencies or system failure.
Second, the integration of cogeneration systems with existing infrastructure presents its own set of difficulties. Many older facilities may not be suitable for the integration of new technologies due to outdated equipment or poor design. This lack of compatibility can necessitate extensive retrofitting or replacement of components, which can be costly and time-consuming. Additionally, upgrades might require careful planning to ensure minimal disruption to current operations.
Moreover, the scalability of cogeneration systems can be another technical hurdle. Not all facilities have the same energy demands, and what works for one may not be effective for another. Thus, achieving optimal sizing and capacity is essential, as oversizing can lead to wasted resources, while undersizing can result in inadequate power generation.
Economic Feasibility Concerns
Economic feasibility is a critical consideration when evaluating steam turbine cogeneration systems. The initial capital investment can be substantial. This includes funding for the turbines, boilers, generators, and associated infrastructure. Many organizations weigh the potential return on investment against the upfront costs. This often leads to cautious decision-making, especially in industries with tight profit margins.
In addition to initial investments, ongoing operational costs should also be factored into the economic analysis. These costs encompass maintenance, fuel, and labor. A miscalculation here can lead to scenarios where the anticipated savings do not materialize, jeopardizing project viability.
The availability of financial incentives and subsidies can vary by region, influencing the attractiveness of cogeneration investments. Lack of consistent policies or uncertainties in governmental support can discourage companies from making moves toward cogeneration.
Despite these challenges, some organizations find that the long-term savings and environmental benefits can outweigh initial economic concerns. Thus, weighing both the economic and technical challenges in light of broader goals is necessary to inform whether steam turbine cogeneration is a strategic path forward for specific applications.
"Understanding and addressing both technical and economic challenges is essential for maximizing the efficiency of cogeneration systems and enhancing their overall viability in energy production."
As we study these challenges and limitations in further detail, it becomes evident that while hurdles exist, they are not insurmountable. Proper planning, investment in technology, and strategic implementation can lead to significant advancements in cogeneration outcomes.
Future Trends in Cogeneration Systems
The future trends in cogeneration systems are crucial in understandng how these systems will evolve and impact energy efficiency. As industries seek to optimize operations and reduce environmental footprints, innovation in cogeneration technology is gaining momentum. Future trends can enhance efficiency, support sustainable energy practices, and integrate with broader energy systems.
Smart Grids and Digital Integration
The advent of smart grids is transforming how energy is distributed and managed. Smart grids utilize digital technology to monitor and control the flow of electricity. This integration facilitates real-time data exchange between power generators, distributors, and consumers. For cogeneration systems, this means improved demand response capabilities. By using digital communication and automation, cogeneration facilities can adjust their output based on demand fluctuations, maximizing efficiency.
Moreover, integration with smart grids helps in optimizing maintenance protocols. Predictive analytics can be used to forecast system failures before they occur, reducing downtime. For industry operators, utilizing smart grid technology leads to
- Increased operational efficiency
- Reduced energy costs
- Enhanced load management
Digital integration allows cogeneration systems to interact with various energy sources, including renewable energies like solar and wind. This interaction will support a more resilient and flexible energy network, making it vital for future development.
Policy Directions and Regulatory Frameworks
Government policies are pivotal in shaping the future of cogeneration systems. Regulatory frameworks can stimulate investments in cleaner technologies, encouraging businesses to adopt cogeneration. Standards might focus on emissions reductions, energy efficiency, and renewable energy usage. By adapting regulations to include cogeneration, authorities can create incentives for industries to transition towards sustainable practices.
Considerations for policy development might include:
- Incentives for Installation: Financial support for businesses that integrate cogeneration solutions.
- Emissions Standards: Setting limits on allowable emissions to promote cleaner technologies.
- Renewable Energy Supports: Inclusion of cogeneration in renewable energy certificates to promote hybrid systems.
By creating a conducive regulatory environment, governments can encourage the uptake of cogeneration systems, ultimately driving advancements in the industry.
"The integration of smart grids and supportive policies will be a cornerstone for the success of future cogeneration systems."
Culmination
The conclusion section synthesizes the key insights and overarching themes presented throughout the article on steam turbine cogeneration systems. It serves as a vital recap for readers, reinforcing why understanding these systems is essential for a range of professionals, including engineers, researchers, and industrial managers.
Summary of Key Points
In summary, steam turbine cogeneration systems represent a critical intersection of efficiency and sustainability in energy production. They harness waste heat for additional power generation, maximizing resource utilization. Key points discussed include:
- Defining cogeneration and its historical context.
- An overview of steam turbines and their operational principles.
- Detailed components that make up cogeneration systems, including steam generation, turbine integration, and heat recovery mechanisms.
- An analysis of system efficiency anchored in thermodynamic principles.
- A discussion surrounding design considerations, economic analysis, environmental impacts, and real-world applications ranging from industrial setups to district heating.
These focal points underscore the importance of cogeneration in improving energy efficiency and reducing the carbon footprint of power generation.
Implications for Future Research
Looking ahead, future research may explore several avenues to enhance the effectiveness of steam turbine cogeneration systems. Topics may include:
- Innovative technologies that further increase operational efficiency and reduce emissions.
- The integration of renewable energy sources, which could bolster the sustainability profile of cogeneration systems.
- Advanced control systems and smart grid technologies that can optimize energy production and distribution in real time.
- Policy frameworks that encourage investment in cogeneration technologies, potentially influencing regulations to favor cleaner energy solutions.
By examining these areas, scholars and practitioners can contribute to the ongoing evolution of cogeneration systems, ensuring they meet the demands of a rapidly changing energy landscape.