Understanding PLA: Comprehensive Insights into Biopolymer
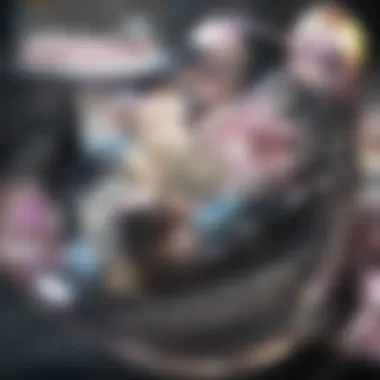
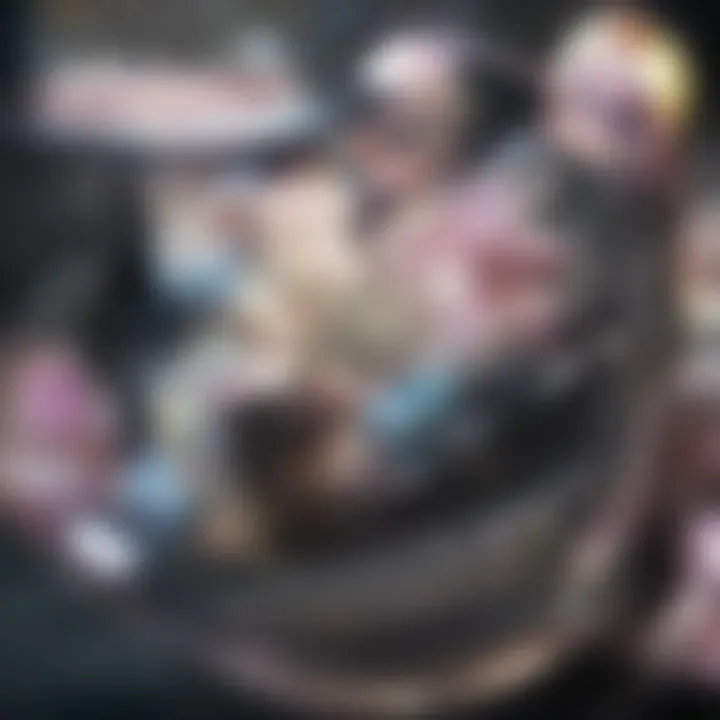
Intro
Polylactic Acid, often abbreviated as PLA, is a biopolymer that finds itself at the intersection of sustainability and technology. Derived from renewable resources, PLA stands out in the realm of materials due to its unique properties. As concerns grow regarding environmental sustainability, this material garners attention for its potential applications across various industries. In this section, we will lay the groundwork for understanding PLA by highlighting its significance, properties, and the manufacturing processes that bring it to life.
Key Findings
Major Results
- PLA is primarily produced from corn starch or sugarcane, making it renewable and biodegradable.
- It exhibits properties such as transparency, flexibility, and resistance to UV light, broadening its applications.
- The production process involves fermentation and polymerization, resulting in a versatile material.
- Key industries utilizing PLA include packaging, textiles, and biomedical devices.
- PLA's environmental profile shows a lower carbon footprint compared to traditional petroleum-based plastics.
Discussion of Findings
The findings indicate that PLA has the potential to significantly replace conventional plastics. Its biodegradable nature helps address issues associated with plastic waste. Furthermore, the energy used in its production is less than that for oil-based plastics. However, it is important to consider the sourcing of raw materials. The competition for land use between food production and bioplastic production is a relevant topic among researchers and policymakers. Thus, while PLA shows promise, careful examination of its entire lifecycle is necessary to ensure that its benefits align with broader environmental goals.
Methodology
Research Design
This article utilizes a multidisciplinary approach, drawing from chemistry, environmental science, and industrial engineering. This design allows for a holistic view of PLA’s synthesis, properties, and impact.
Data Collection Methods
Data was compiled through a combination of literature reviews, case studies, and industry reports. Peer-reviewed journals provided primary research data on PLA's properties and manufacturing processes. Additionally, interviews with industry stakeholders offered insights into current applications and future trends.
"Understanding the full scope of PLA’s implications requires a collaborative approach, drawing from various fields to decipher its environmental and economic impact."
What is PLA?
Polylactic Acid (PLA) is gaining significant attention as a prominent biopolymer derived from renewable resources. Understanding its core characteristics, historical significance, and current utilization is essential for appreciating its role in various industries and its potential for sustainability. This section elaborates on the definition, overview, and historical context of PLA, shedding light on how it has evolved and why it matters today.
Definition and Overview
PLA is a biodegradable thermoplastic made from fermented renewable resources, primarily cornstarch or sugarcane. Its importance lies in both its physical properties and chemical structure that allow its use in a variety of applications. Unlike traditional petrochemical-based plastics, PLA is seen as a more eco-friendly option due to its lower environmental impact throughout its life cycle.
PLA exhibits favorable characteristics such as high transparency, low thermal conductivity, and good mechanical strength. It can be processed through various methods including injection molding and 3D printing. These attributes make it highly applicable in sectors ranging from packaging to biomedical devices.
An essential aspect of PLA is its ability to decompose in industrial composting facilities, where specific conditions facilitate microbial action, leading to its breakdown into natural elements. This feature aligns with the increasing demand for sustainable materials in the face of global plastic pollution concerns.
Historical Context
The development of PLA is rooted in advancements in polymer science and sustainable practices. The first notable production of PLA dates back to the 1930s when a chemist named Wallace Carothers began researching polylactic acids. However, significant commercialization efforts didn't occur until the 1990s. Research from companies such as Cargill and Dow in the late '80s laid the groundwork for large-scale production.
Interest in PLA grew substantially as environmental awareness increased in the 21st century. The emergence of the bioplastics market is directly linked to PLA’s properties that allow it to replace conventional plastics. Made principally from renewable resources, PLA fits into the health and ecology discourse, pushing forward the narrative of minimizing carbon footprints.
To sum up, understanding PLA is crucial not only for its material properties but also for its role as a catalyst for change in the plastics industry. Its historical development parallels the growing urgency for sustainable alternatives in a world filled with environmental dilemmas.
"PLA not only offers a glimpse into the future of materials science but also aligns with the principles of sustainability and eco-friendliness, making it a pivotal player in modern industry."
In the sections to follow, we will delve into the chemical composition of PLA, its manufacturing processes, and its varied applications, as well as its impact on the environment and future developments.
Chemical Composition of PLA
Understanding the chemical composition of Polylactic Acid (PLA) is fundamental to appreciating its applications and advantages. PLA, a biodegradable thermoplastic derived from renewable resources, displays unique properties that contribute to its versatility in various industries. The composition directly impacts its functionality, processing, and end-of-life criteria. Key elements to focus on include its molecular structure, stereochemistry, and the raw materials used in its production.
Basic Structure
PLA is primarily composed of lactic acid monomers, which are linked through ester bonds to form long polymer chains. The polymerization process can occur via two main methods: anionic polymerization and ring-opening polymerization. The basic repeating unit of PLA is the d-lactic acid or l-lactic acid. The proportion of these two forms influences the copolymer's characteristics, such as crystallinity and mechanical properties.
- Homopolymer vs Copolymer:
- Homopolymer PLA consists solely of one type of lactic acid monomer, usually leading to enhanced clarity and simplicity in processing.
- Copolymer PLA, which is a blend of different lactic acid monomers, exhibits varied properties such as improved flexibility and impact resistance.
The molecular weight of PLA also plays a crucial role. Higher molecular weights generally enhance tensile strength and thermal resistance, while lower molecular weights improve processability but may compromise strength. Manufacturers must balance these factors depending on the intended application.
Stereochemistry
Stereochemistry refers to the spatial arrangement of atoms in the molecular structure, which can greatly influence the polymer's properties. PLA can exist in three stereochemical forms: L-lactic acid, D-lactic acid, and their racemic mixture. This variation introduces important characteristics:
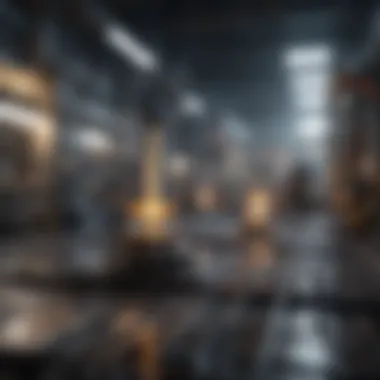
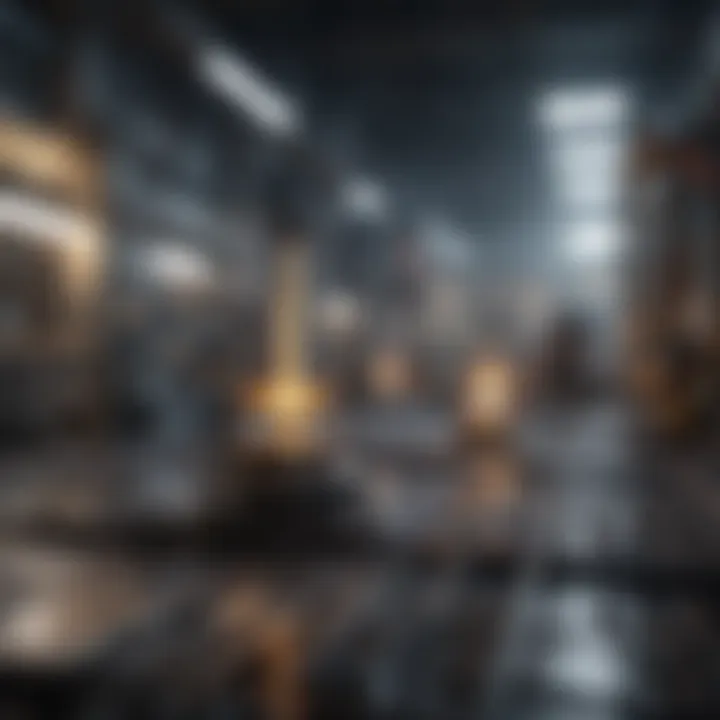
- L-lactic Acid: Predominant in most commercial PLA, it provides superior physical performance, such as tensile strength and clarity.
- D-lactic Acid: Less common, but when blended with L-lactic, it can enhance the overall mechanical properties by affecting the crystallization behavior.
- Racemic Mixture: A balanced combination of L and D-lactic acid. This form tends to have a lower melting temperature and is less crystalline, which affects how it can be processed and its final physical properties.
The resulting polymers from these components affect not only the mechanical attributes of PLA but also its biodegradability and rate of degradation under environmental conditions. The stereochemistry of PLA is vital for tailoring its properties to suit specific applications, such as in packaging or biomedical uses.
"Understanding the basic structure and stereochemistry of PLA is crucial for optimizing its performance in diverse applications."
Manufacturing Processes
Understanding the manufacturing processes associated with Polylactic Acid (PLA) is vital for appreciating its versatility and impact in various industries. These processes define the quality, efficiency, and sustainability of PLA production, influencing its adoption across sectors.
Polymerization Techniques
Polymerization techniques are critical steps in PLA manufacture, as they directly affect the properties of the final product. There are primarily two methods of polymerization: ring-opening polymerization (ROP) and condensation polymerization.
- Ring-Opening Polymerization: This method is the most common for PLA production. It involves the use of lactide, which is formed by the dehydration of lactic acid. The lactide is then subjected to catalysts to initiate the polymerization process. This technique allows for better control over molecular weight and polymer structure.
- Condensation Polymerization: This process involves the direct polymerization of lactic acid monomers. It generally requires higher temperatures and does not yield as high a molecular weight as ROP. However, it is less expensive and can be simpler to execute in smaller manufacturing setups.
Each of these techniques has its own advantages and limitations. ROP is favored for large-scale production due to its ability to create high-performance PLA products, while condensation polymerization can be useful for producing lower-grade material or in situations where cost is a major constraint.
Production Scale Considerations
Scaling up PLA production involves several considerations that influence both economic viability and environmental impact. Some factors to evaluate include:
- Economies of Scale: Larger production facilities can reduce costs through mass production. However, initial investments are high.
- Supply Chain Logistics: Sourcing raw materials, primarily from renewable resources such as corn or sugarcane, can affect production scale. Fluctuations in supply can disrupt manufacturing.
- Market Demand: Understanding the demand is essential for determining the scale of production. Overproduction can lead to waste, while underproduction may limit market opportunities.
Changes in technology also play a significant role in production scalability. Advances in biopolymer processing and manufacturing equipment can lead to more sustainable practices, benefiting both producers and the environment. Companies must stay abreast of trends in technology and market demands to optimize their production strategies.
"The choice of polymerization technique and production scale can significantly influence the functional properties and market success of PLA products."
In summary, manufacturing processes for PLA encompass complex decisions that involve balancing technical feasibility with market and environmental considerations. Attention to polymerization methods and production scale is necessary for the successful implementation and application of PLA in various industries.
Applications of PLA
The applications of Polylactic Acid (PLA) are vast and versatile. This biopolymer demonstrates significant utility in various industries, offering both functional advantages and environmental benefits. Its renewability and compatibility with existing manufacturing processes position it as a favorable alternative to conventional plastics. Understanding the applications of PLA aids in recognizing its potential to influence sustainability and innovation in material science.
Packaging Solutions
One of the primary applications of PLA is in the packaging industry. PLA's biodegradability and compostability appeal to manufacturers aiming to reduce their environmental footprint. Products packaged with PLA can decompose within months under the right conditions. This characteristic makes it an excellent choice for single-use items such as food containers, cutlery, and bags.
The clarity and strength of PLA also enhance its functionality. Consumers enjoy the aesthetic while being environmentally conscious. Furthermore, PLA can be processed similarly to traditional plastics, allowing companies to pivot without substantial alterations to their production lines.
Key advantages of PLA in packaging include:
- Compostable nature reduces landfill waste.
- Greater consumer appeal as eco-friendly material.
- Possibility to maintain shelf life of products.
However, considerations such as moisture sensitivity must be addressed. PLA can lose its integrity in humid environments, which limits its applications in certain areas.
Textile Industry
PLA also makes significant strides in the textile sector. Its use is increasingly prevalent in producing fibers for clothing, home textiles, and nonwoven fabrics. The biodegradability of PLA fibers presents an opportunity to create environmentally friendly garments.
In the textile industry, PLA can be blended with other fibers to enhance properties such as moisture management and durability. PLA offers a soft feel and dynamic drapability, appealing to both consumers and manufacturers. Innovations in yarn spinning have also expanded its use in various fabric types, contributing to comfort and performance.
Specific applications in textiles include:
- Activewear that combines performance and sustainability.
- Home furnishings, like curtains and bedding, that align with eco-friendly practices.
Nevertheless, PLA’s thermal stability can vary. Fabrics may require care during washing as high temperatures can lead to deformation.
Medical Applications
The medical field is another area where PLA exhibits substantial potential. Biodegradable medical implants, sutures, and drug delivery systems can leverage PLA's properties. The ability of PLA to degrade safely in the body over time presents new possibilities for non-invasive procedures and patient care.
PLA's compatibility with the human body is well-documented. It can be formulated to release medications gradually, offering targeted therapeutic effects. This property decreases the need for repeated surgeries or interventions. Surgical meshes and pins have also seen integration of PLA, offering a blend of strength and biocompatibility.
Key benefits of PLA in medical applications include:
- Reduced risk of foreign body reactions.
- Potential for decreased patient recovery times.
- Minimized need for follow-up surgeries.
Nonetheless, challenges in mechanical strength and degradation rates exist. Further research may be necessary to improve the performance of PLA-based materials in this sensitive sector.
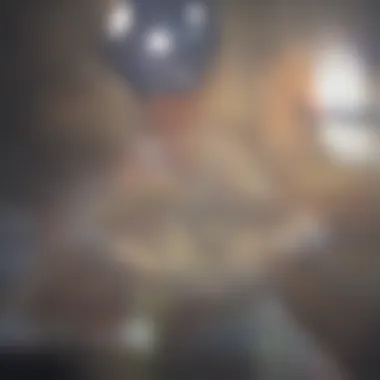
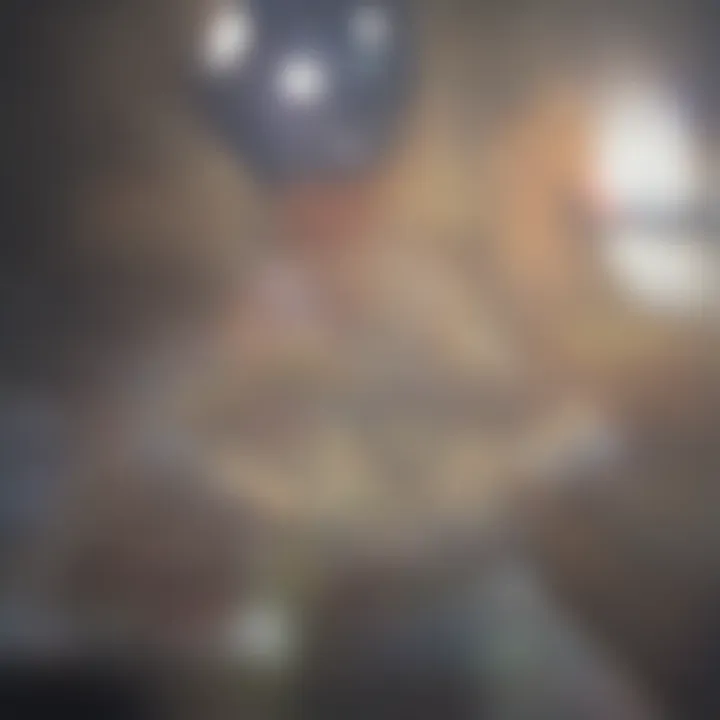
In summary, the applications of PLA are diverse, each with its own unique benefits and considerations. This biopolymer's role in sectors such as packaging, textiles, and medicine illustrates its potential to support sustainable practices while providing practical solutions. As the demand for environmentally conscious materials grows, PLA's significance will likely become more pronounced.
Environmental Impact
The environmental impact of Polylactic Acid (PLA) is a crucial area of exploration in understanding its overall significance. As industries increasingly shift towards sustainable practices, PLA stands as a strong candidate due to its bio-based origin. This section examines key elements such as the material’s biodegradability and its implications on the entire life cycle of manufacturing and usage.
Biodegradability
PLA boasts a notable advantage with its excellent biodegradability. Unlike conventional plastics, which can linger in landfills for centuries, PLA breaks down into natural substances under appropriate composting conditions. Specifically, in industrial composting environments with controlled temperature and moisture levels, PLA can decompose within a few months.
This characteristic positions PLA favorably in addressing the pervasive problem of plastic waste. Reducing landfill contributions is essential in combating pollution and minimizing the ecological footprint of manufacturing processes. However, it’s important to note that biodegradability is contingent on the environment where PLA is disposed. Home composting may not yield the same results as industrial facilities. Thus, public awareness and adequate infrastructure are critical for maximizing this benefit.
"PLA’s capacity for biodegradation emphasizes the necessity of proper disposal systems to fully leverage its environmental benefits."
Life Cycle Assessment
Life Cycle Assessment (LCA) is another vital aspect in evaluating PLA's environmental impact. This systematic approach assesses the environmental and resource implications throughout the product’s life, from raw material extraction to manufacturing, distribution, usage, and final disposal.
Conducting an LCA on PLA can reveal insights into its carbon footprint compared to traditional plastics, helping stakeholders make informed decisions. Factors considered include:
- Raw Material Sourcing: PLA is made from renewable resources, primarily corn or sugarcane, which significantly reduces reliance on fossil fuels.
- Manufacturing Energy: While production does require energy, innovations in renewable energy integration are continuously improving efficiencies.
- End-of-Life Management: The LCA must account for the disposal methods available, emphasizing the advantages of composting versus traditional landfill disposal.
Given that numerous variables affect LCA outcomes, it’s crucial to utilize up-to-date data and practices to ensure accurate assessments. Research must consistently evolve to provide a clearer picture of PLA's long-term impact on sustainability and environmental health.
Benefits of Using PLA
The benefits of using Polylactic Acid (PLA) are manifold and pivotal, particularly in the context of sustainability and eco-friendly practices. As industries look for alternatives to conventional plastics, PLA offers a viable solution due to its unique properties and advantages. Its derived nature from renewable resources positions it as a key player in reducing dependency on fossil fuels. This section will delve into the significant benefits of PLA, focusing on specific elements like renewability and its contribution to a lower carbon footprint.
Renewability
PLA is predominantly made from corn starch or sugarcane, making it a renewable resource. This characteristic is crucial in today's world where the depletion of fossil fuels poses a major threat. Unlike traditional plastics, which are derived from non-renewable sources, PLA offers a sustainable solution.
- Sourcing: By utilizing agricultural products, PLA production supports sustainable farming practices. This creates a system where the feedstock can be replanted and harvested without exhausting natural resources.
- Biodiversity Support: The use of agricultural resources encourages diverse farming practices, which can contribute positively to local ecosystems.
- Economic Benefits: The local economic boost from growing crops for PLA production brings opportunities for farmers and reduces the need for importing raw materials, which can be environmentally taxing.
By shifting to renewable sources for material production, industries can significantly lower their environmental impact over time.
Low Carbon Footprint
Another critical benefit of PLA is its low carbon footprint throughout its lifecycle. This factor is significant in combating climate change and minimizing greenhouse gas emissions.
- Production Efficiency: The manufacturing process of PLA generates substantially lower amounts of carbon dioxide compared to petroleum-based plastics. This is largely due to the energy sources used in the production.
- End-of-Life Options: PLA is compostable under industrial conditions, which leads to a reduction in solid waste in landfills. When composted, PLA breaks down into carbon dioxide, water, and organic materials, completing a sustainable cycle. This reduces the overall carbon emissions associated with waste management.
- Lifecycle Analysis: Studies show that the total greenhouse gas emissions from PLA, from production to disposal, are significantly lower than comparable petroleum-based plastics. This finding highlights PLA's potential in contributing to greenhouse gas reduction strategies.
"The utilization of PLA in various industries can facilitate a shift towards more sustainable practices and offer tangible benefits in carbon reduction."
The low carbon footprint of PLA illustrates its role in a future that prioritizes environmental considerations.
Challenges and Limitations
Understanding the challenges and limitations of Polylactic Acid (PLA) is crucial for industries considering its application. Despite its many benefits, PLA is not without its drawbacks. Recognizing these limitations can inform research and development efforts, guiding improvements in material properties and expanding its usage across various sectors. In this section, two main aspects will be discussed: mechanical properties and thermal stability.
Mechanical Properties
PLA exhibits unique mechanical properties that can be both advantageous and disadvantageous. It tends to be stiff and strong, making it suitable for applications where rigidity is necessary. However, the brittleness of PLA can pose significant challenges in practical use. This brittleness results in lower impact resistance when compared to other materials like polyethylene (PE) or polypropylene (PP). For instance, products made from PLA may fracture under stress or during handling, compromising their usability in certain environments.
Key points regarding the mechanical properties of PLA include:
- Tensile Strength: PLA offers good tensile strength, which can be beneficial in many structural applications.
- Flexural Modulus: Its high flexural modulus contributes to stiffness, but it may also lead to a reduced ability to withstand bending without breaking.
- Fatigue Resistance: The material’s fatigue endurance can be inadequate for applications requiring repetitive stress.
To improve mechanical properties, various strategies are being explored, including blending PLA with other polymers to enhance ductility or using fillers to increase toughness. These modifications can lead to a more versatile material, paving the way for wider applications while addressing existing limitations.
Thermal Stability
Thermal stability is another essential aspect of PLA that researchers and manufacturers must consider. PLA has a relatively low thermal degradation temperature, making it unsuitable for high-temperature applications. The decomposition temperature of PLA is around 60 to 65 degrees Celsius, which means that exposure to higher temperatures can lead to loss of structural integrity.
important factors regarding thermal stability include:
- Heat Deflection Temperature (HDT): The heat deflection temperature for PLA is considerably lower compared to many conventional plastics. This can limit its use in applications involving heat exposure.
- Processing Conditions: When manufacturing with PLA, care must be taken with processing conditions, as high temperatures during processing can cause degradation.
- Environmental Exposure: PLA is sensitive to environmental conditions, such as humidity and temperature fluctuations, which can affect its performance over time.
Improving the thermal stability of PLA can significantly enhance its applicability. Research into copolymers and additives is ongoing to address these challenges. The goal is to produce PLA formulations that can withstand typical conditions encountered in various applications without compromising its advantageous properties.
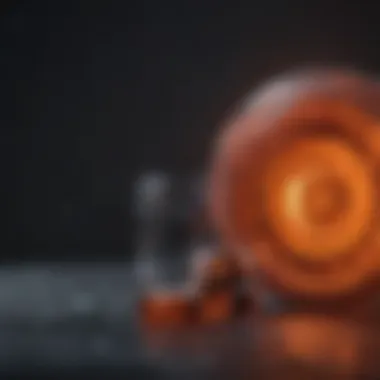
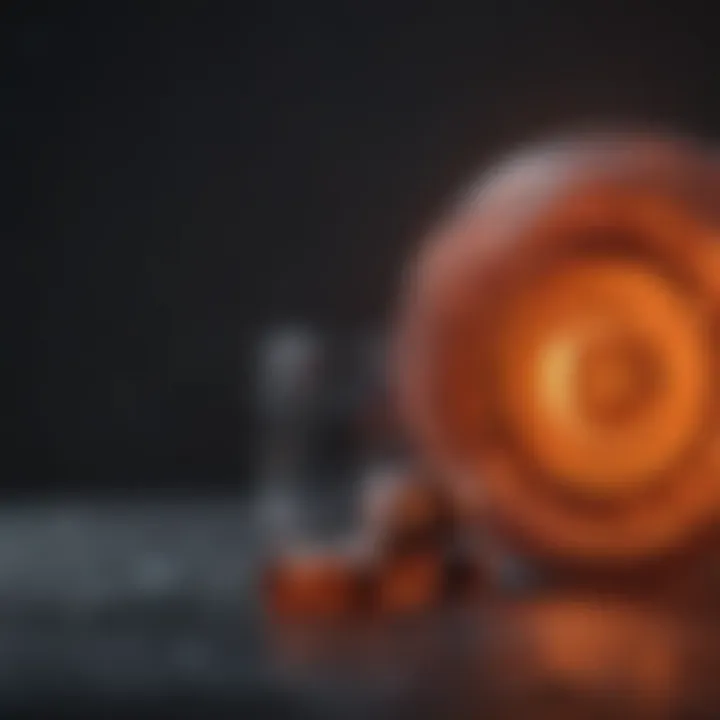
"Understanding the challenges of PLA not only highlights its limitations but also serves as a guide for advancements in biopolymer technology."
In summary, while PLA presents promising benefits for sustainability and versatility, its mechanical and thermal challenges must be addressed to unlock its full potential. Continued research in this domain will contribute to the development of more resilient and adaptable bio-based materials.
Future Developments in PLA Research
Future developments in Polylactic Acid (PLA) research hold significant potential for enhancing its utility in various applications. As global focus shifts toward sustainability, the role of PLA as an eco-friendly alternative to traditional plastics gains importance. Researchers are investigating methodologies to improve PLA's properties, making it more applicable across industries. Advancements in polymer technology and understanding of market trends are crucial elements in this pursuit.
Advancements in Polymer Technology
Current research in polymer technology aims at overcoming some of the challenges associated with PLA. This includes modifying its mechanical strength and thermal stability to enhance performance in demanding applications. Innovations such as copolymerization and the incorporation of additives may result in composites with improved resilience and functionality. Furthermore, emerging techniques like 3D printing are also being integrated with PLA, facilitating the production of intricate designs that were previously unachievable.
- Copolymerization: This technique allows for the blending of PLA with other polymers, tailoring properties to meet specific requirements.
- Additives: Incorporating certain additives could improve mechanical properties and thermal resistance, broadening the scope of PLA applications.
"Research indicates that strategic modifications in PLA may close the performance gap with conventional plastics, potentially revolutionizing its market presence."
By focusing on innovation in these areas, researchers aim to maximize the potential of PLA, pushing it towards wider acceptance in the manufacturing sector and beyond.
Market Trends
The market for PLA is evolving. Increased awareness of environmental issues drives demand for biopolymers. There is a growing preference for renewable materials over petroleum-based products. This shift reflects in consumer behavior as well as corporate strategies. Many companies integrate sustainability into their business models, viewing PLA as a viable option.
Current trends show increasing applications of PLA in sectors such as:
- Packaging: Manufacturers are looking for biodegradable alternatives to reduce plastic waste.
- Textiles: Brands explore the use of PLA in fabrics that offer both performance and eco-friendliness.
- Medical Devices: The biocompatibility of PLA makes it attractive for use in medical applications, from sutures to drug delivery systems.
Staying attuned to these market movements is essential for stakeholders in the industry. Establishing partnerships and collaborating in research could also enhance the visibility of PLA in different markets. As research continues, the eventual goal is to position PLA as a mainstream polymer, achieving both ecological benefits and commercial viability.
Comparative Analysis with Other Biopolymers
Comparative analysis of Polylactic Acid (PLA) with other biopolymers is essential in understanding its unique properties and advantages. This analysis allows researchers and industry professionals to evaluate the performance of PLA against its counterparts, guiding decisions about material selection based on specific application needs. PLA stands out in its renewability and biodegradability, characteristics that are increasingly important in a world focused on sustainability.
One primary reason for conducting a comparative analysis is to identify the specific strengths and shortcomings of PLA in relation to other materials. Comparing PLA with biopolymers like Polyhydroxyalkanoates (PHA) and Polyethylene Terephthalate (PET) reveals a multitude of factors including processing conditions, mechanical properties, and economic feasibility. Such knowledge empowers stakeholders to optimize their products and innovate new applications for these bioplastics.
PLA vs. PHA
When comparing PLA to PHA, several key differences emerge. PHA is known for its excellent biodegradability and flexibility, making it suitable for a wide range of applications. However, PLA is more rigid and has higher tensile strength, which can be advantageous in structural applications. Additionally, the production costs for PHA tend to be higher due to the complexity of its manufacturing process, while PLA can be produced from relatively inexpensive feedstocks such as corn starch or sugarcane.
The environmental impacts of both materials also differ. Both PLA and PHA are compostable, but PLA often faces challenges related to recycling. Many facilities are not equipped to deal with PLA, while PHA can be processed more easily. In terms of market acceptance, PLA has already established a stronger presence, especially in packaging applications.
PLA vs. PET
The comparison between PLA and PET showcases another significant relationship in biopolymer selection. PET is widely used in various industries, especially for packaging and textiles, due to its strength and durability. However, PET is derived from fossil fuels and is not biodegradable, leading to environmental concerns.
In contrast, PLA is entirely renewably sourced and offers the advantage of being biodegradable under certain conditions. However, PLA typically has lower thermal stability than PET, which limits its application in high-heat contexts.
Moreover, the recycling and disposal pathways differ considerably. While PET can be mechanically recycled, PLA requires specific composting conditions to break down effectively, leading to challenges in waste management. As consumer preferences shift towards eco-friendly alternatives, PLA’s advantages in sustainability may offer it an edge over PET in the long run.
Overall, comparative analysis with other biopolymers reinforces the complexity of material choice in industry today. By understanding the properties and contexts of materials like PHA and PET against PLA, manufacturers can make informed decisions that align with both performance and sustainability objectives. This analysis is pivotal in guiding research, development, and market strategies as the world moves towards greater reliance on bioplastics.
Culmination
The conclusion serves as a critical component of this discussion on Polylactic Acid (PLA). It synthesizes all findings, helping readers understand the significance of PLA in modern applications and its relevance in sustainability. By summarizing key insights, it reinforces the importance of PLA's chemical properties, manufacturing processes, and practical uses.
In addition, this section allows for reflection on the complex nature of PLA compared to other biopolymers like PHA and PET. Recognizing its unique attributes presents an opportunity to appreciate its role within a larger ecological context. This inspection leads to a deeper understanding of how PLA can contribute to environmental sustainability and innovate industries ranging from packaging to textiles.
Ultimately, a well-crafted conclusion provides clarity, tying together the diverse strands of information shared throughout the article. It highlights not only the achievements but also the limitations of current PLA applications and opens the door for future advancements. As research progresses, it is evident that attention to PLA's potential can yield significant benefits in various sectors, thus maximizing its impact:
- Biodegradable solutions in packaging.
- Innovative textiles for sustainable fashion.
- Medical devices that are both effective and environmentally friendly.
This underscores the crucial notion that fostering ongoing research and development will yield indispensable insights into PLA's capabilities.
Summary of Findings
A thorough exploration of Polylactic Acid has uncovered several noteworthy findings. PLA is recognized as a biopolymer primarily derived from renewable resources such as cornstarch. Its significant attributes include:
- Biodegradability: PLA decomposes under industrial composting conditions, making it a preferable alternative to conventional plastics.
- Versatility in applications: From packaging to biomedical devices, its adaptability highlights PLA's wide usability.
- Sustainability factors: Its production from renewable resources aligns with global sustainability goals, decreasing reliance on fossil fuels.
The analysis also outlines its limitations, including certain mechanical properties and thermal stability issues that can affect its implementation in various applications. Despite these challenges, PLA remains a prominent choice in the quest for sustainable alternatives.
Implications for Future Research
Future research into PLA is paramount for several reasons. As industries increasingly shift toward sustainable practices, understanding PLA's full potential becomes essential. Key areas for investigation include:
- Enhancing mechanical properties: Research aimed at improving PLA's strength and thermal stability can broaden its applications.
- Developing new blends: Creating composite materials that combine PLA with other sustainable substances can lead to improved functionalities.
- Lifecycle analysis: Evaluating the complete lifecycle of PLA, including production and disposal, can provide insights into its overall impact on the environment.