Understanding Hemodialysis Membranes: In-Depth Analysis
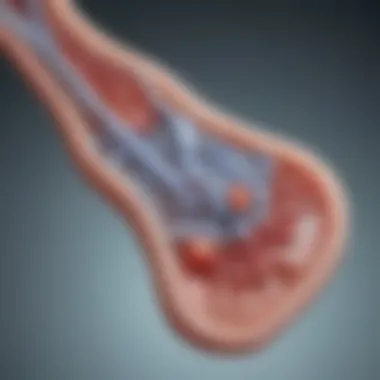
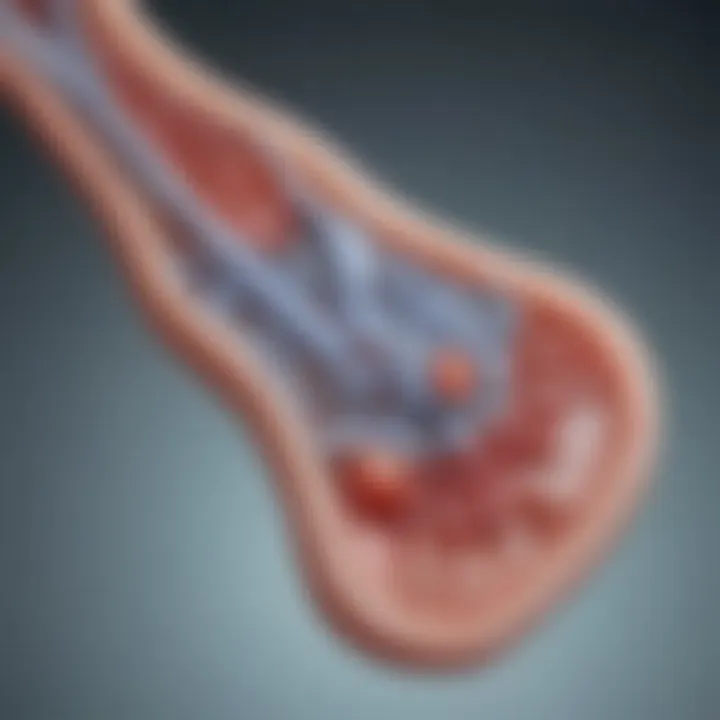
Intro
Hemodialysis membranes play a crucial role in renal replacement therapy, enabling effective treatment for patients with kidney failure. These membranes serve as semi-permeable barriers, allowing for the selective transfer of substances between blood and dialysate. Understanding these membranes is essential for healthcare professionals and researchers alike, as advancements in technology and material science can significantly impact patient outcomes. This article will cover various types of hemodialysis membranes, their materials, and mechanisms, providing a comprehensive overview for a discerning audience.
Key Findings
Major Results
Research indicates that hemodialysis membranes have evolved significantly over the last few decades. Various types of membranes are now available, each offering distinct advantages and challenges. The development of synthetic materials, such as polysulfone and polyamide, has improved biocompatibility and permeability.
The main types of membranes include:
- High-flux membranes: These allow for the passage of larger molecules, enhancing the removal of toxins from the blood.
- Low-flux membranes: These are more selective, primarily used for the removal of smaller waste products.
- Biocompatible membranes: Designed to minimize adverse reactions, these include surface modifications that enhance interaction with blood components.
Each type of membrane has specific physiological implications that directly affect dialysis efficacy and patient well-being.
Discussion of Findings
In-depth analysis shows that high-flux membranes provide significant benefits for certain patient populations. They enable the efficient clearance of middle molecules, which are often associated with poor outcomes. However, the choice of membrane must be customized based on individual patient needs, as some may have contraindications or specific clinical considerations.
"The advancements in membrane technology have altered the landscape of dialysis treatment, offering tailored options for various disease states."
Additionally, ongoing research is vital to continuously improve membrane functionality, longevity, and patient safety. Further studies will likely explore nanotechnology and other innovative approaches to advance this field.
Methodology
Research Design
This article adopts a descriptive research design, synthesizing existing literature on hemodialysis membranes. Relevant studies were selected based on their contributions to understanding the various properties, mechanisms, and clinical implications of these membranes.
Data Collection Methods
Data was gathered through a systematic review of peer-reviewed journals, clinical guidelines, and technical reports. Sources include:
- Academic databases such as PubMed and Scopus
- Renowned medical websites like Wikipedia, Britannica, and relevant medical associations.
Research findings were also corroborated through expert opinions shared in forums like reddit.
Through this approach, the article aims to present a detailed yet clear overview of hemodialysis membranes, catering to professionals and researchers in the field.
Prolusion to Hemodialysis Membranes
Hemodialysis membranes serve as a critical component in renal replacement therapy. Their primary function is to filter waste products from the blood of patients with kidney failure. Understanding the properties and functions of these membranes is vital for practitioners in nephrology and related fields. This introductory section will articulate the significance of hemodialysis membranes, including their design, performance, and the implications for patient outcomes.
Definition and Functionality
Hemodialysis membranes are semipermeable barriers made primarily from synthetic or modified natural materials. They separate blood from a dialysate solution, allowing movement of solutes while keeping blood cells and larger proteins contained. The basic goal is to remove toxins like urea and creatinine, and to balance electrolyte levels in the bloodstream. The efficiency of these membranes directly influences the effectiveness of hemodialysis treatment, making their functionality a focal point in dialysis technology.
Key functionalities of hemodialysis membranes include:
- Diffusion: This is the process of balancing solute concentrations between blood and dialysate. Substances move from an area of higher concentration to one of lower concentration.
- Ultrafiltration: This is the removal of excess fluid. It occurs due to the pressure gradient between blood and dialysate, helping to manage fluid overload in patients.
- Surface Area: A larger membrane surface area typically enhances efficiency, allowing for more extensive filtration during each treatment session.
The optimal design balances factors such as permeability, selectivity, and durability to ensure safe and effective patient care. In considering these elements, clinicians can select appropriate membranes tailored to patient needs.
Historical Development
The evolution of hemodialysis membranes can be traced back to the early 20th century. Initially, natural materials such as cellulose were utilized. These early membranes presented limitations, particularly regarding biocompatibility and filtering efficiency.
As technology advanced, synthetic membranes emerged. This shift began in the 1950s with the introduction of polyacrylonitrile, a major breakthrough that led to improved performance. Organizations and researchers kept refining materials through the late 20th century, paving the way for modern high-flux membranes that allow for effective solute clearance.
Today, various types of membranes exist, including cellulose-based and synthetic options, each with distinct performance characteristics. The focus on enhancing biocompatibility and minimizing adverse reactions has driven the research and development of these technologies. Notably, the advent of biocompatible materials like polysulfone has resulted in considerable improvements in patient care and outcomes.
In summary, comprehending the history and function of hemodialysis membranes provides essential insights for medical professionals and researchers. This knowledge lays the groundwork for ongoing advancements and refinements that drive better health outcomes for patients undergoing hemodialysis.
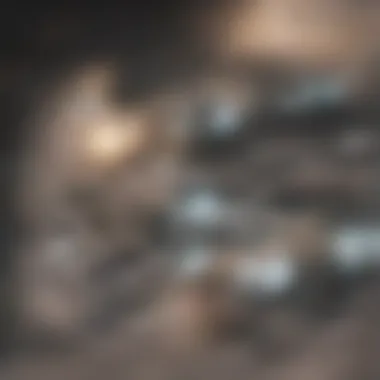
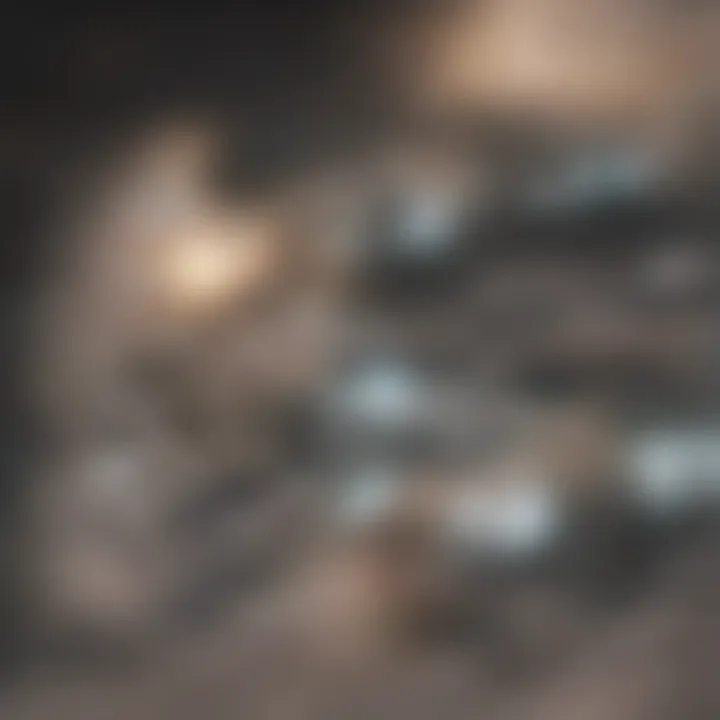
Types of Hemodialysis Membranes
The classification of hemodialysis membranes is integral to understanding the options available in renal replacement therapy. The differentiation between membrane types is crucial for tailoring treatment to the patient's needs, ensuring effective dialysis while minimizing complications. This section elaborates on three primary categories of membranes: cellulose-based membranes, synthetic membranes, and the comparison between high-flux and low-flux membranes.
Cellulose-based Membranes
Cellulose-based membranes have been used in hemodialysis for decades. They are derived from naturally occurring cellulose, making them relatively inexpensive and widely available. These membranes offer distinct characteristics, including adequate clearance of small solutes, though their performance can be limited when it comes to clearing larger molecules.
The advantages of cellulose-based membranes include good biocompatibility and a well-established safety profile. However, their drawbacks include a higher risk of clotting due to their hydrophobic properties and a lower filtration rate compared to synthetic alternatives. The uniqueness of these membranes lies in their firm yet flexible structure, which accommodates varying blood flow rates during treatment.
Synthetic Membranes
Synthetic membranes represent a significant advancement in hemodialysis technology. Composed of biocompatible polymers, these membranes are designed to enhance solute clearance and reduce the risk of adverse reactions. Synthetic membranes allow for a broader range of pore sizes, enabling them to effectively remove both small and larger molecules from the bloodstream.
The benefits of synthetic membranes include improved flux rates and better patient outcomes. For instance, polysulfone and polyethersulfone are common materials used in synthetic membranes, providing enhanced transport of solutes and reduced accumulation of harmful substances in patients. The increased biocompatibility minimizes inflammatory responses, promoting a more favorable dialysis experience.
High-flux vs Low-flux Membranes
Understanding the distinction between high-flux and low-flux membranes is essential for selecting the appropriate dialysis treatment. High-flux membranes enable a greater permeation rate, facilitating the removal of larger molecules and a more efficient clearance of middle molecules, which are often associated with dialysis-related complications.
On the other hand, low-flux membranes tend to have lower permeability and are suitable for patients where the clearance of small solutes suffices. They may present fewer complications during treatment, making them preferable for certain patient populations. In summary, the choice between high-flux and low-flux membranes should consider the specific medical requirements of the patient, balancing efficacy against safety.
The choice of hemodyalisis membrane can significantly affect patient outcomes, making it crucial to understand their differences and applications.
Material Composition
The composition of hemodialysis membranes plays a significant role in their performance, biocompatibility, and overall efficiency in renal replacement therapy. Understanding the materials used in these membranes allows for better selection and optimization for patient care. The choice of material can influence the extent of toxin removal, the filtering characteristics of the membrane, and the response of the patient’s immune system.
Polymer Materials
Polymer materials are fundamental in the design of hemodialysis membranes. Common polymers include polysulfone, polyethersulfone, and polyamide. Each of these polymers brings distinct advantages. For instance, polysulfone membranes offer high flux rates, which facilitate the movement of solutes across the membrane, making them ideal for patients needing efficient treatment. Moreover, the thermal and chemical stability of these polymers ensures their longevity and effectiveness during repeated uses.
The choice of polymer directly impacts the performance metrics of the membrane, such as permeability and selectivity. This allows healthcare providers to tailor treatments based on individual patient needs, achieving better outcomes.
Membrane Structure and Pore Size
The structure and pore size of a membrane are critical parameters that dictate how effectively a hemodialysis membrane can perform its function. Membranes can be designed with varying pore sizes, which affects what substances can pass through. Typically, membranes have a pore size range between 1 to 100 nanometers.
With larger pores, a membrane can remove larger molecules, but it may also allow some unwanted substances to pass. Conversely, smaller pores offer increased selectivity but might impede the flow of certain necessary solutes.
"Optimizing pore size according to the patient's clinical needs can be a key determinant in enhancing dialysis efficacy."
In summary, the material composition of hemodialysis membranes, focusing on polymer choice and structural characteristics, underpins their function in dialysis. Selecting suitable materials and designing effective pore structures is vital for the development of advanced hemodialysis technology.
Mechanism of Action
The mechanism of action of hemodialysis membranes plays a crucial role in the effectiveness of the hemodialysis process. Understanding how these membranes function can help in optimizing patient outcomes in renal replacement therapy. Their primary goal is to facilitate the clearance of metabolic waste products and excess electrolytes from a patient’s bloodstream. The main processes involved are diffusion and ultrafiltration, both of which will be discussed in detail.
Diffusion and Ultrafiltration
Diffusion is the process by which solutes move from an area of higher concentration to an area of lower concentration. In hemodialysis, this means that waste products in the blood, such as urea and creatinine, naturally pass through the semi-permeable membrane into the dialysate, which has a lower concentration of these substances. This movement is essential for effective detoxification during dialysis sessions.
Ultrafiltration, on the other hand, is the movement of water across the membrane. Here, hydrostatic pressure is applied, which forces water and small solutes through the pores of the membrane into the dialysate. This process is vital, especially in cases of fluid overload in patients, as it aids in removing excess fluids from the body.
The combination of diffusion and ultrafiltration allows for efficient waste removal and fluid management in patients undergoing hemodialysis. Understanding these processes can inform clinicians about proper membrane selection and dialysis settings for individual patients.
Role of Dialysate
The dialysate is a specially formulated fluid that plays a significant role in the hemodialysis process. It creates a concentration gradient necessary for diffusion to occur. The composition of the dialysate is crucial; it typically includes electrolytes, buffers, and other substances that mirror the body's natural fluid balance. This composition allows for optimal removal of toxins and regulation of electrolyte levels during the dialysis procedure.
- Key components of dialysate include:
- Electrolytes such as sodium and potassium
- Bicarbonate for buffering
- Glucose, depending on fluid management needs
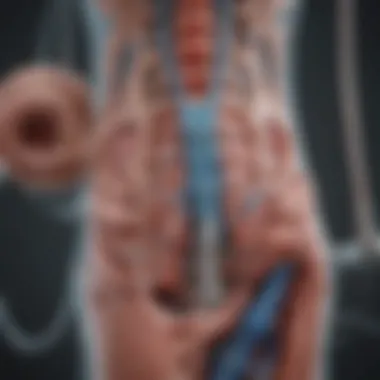

Choice of dialysate can greatly influence the overall effectiveness of hemodialysis. It requires careful consideration based on each patient's metabolic needs. Adjustments to the dialysate can help enhance the clearance rates of specific toxins and control fluid balance. Thus, understanding the role of dialysate alongside the mechanism of action of membranes can lead to improved treatment protocols and patient outcomes.
The efficiency of diffusion and ultrafiltration directly impacts the overall success of hemodialysis treatments, making the understanding of these mechanisms imperative for all clinicians involved in renal care.
Performance Evaluation
Performance evaluation is crucial in understanding the effectiveness and safety of hemodialysis membranes. This assessment helps determine how well these membranes function in removing toxins and excess fluid from the blood during dialysis treatments. Evaluating performance can provide insight into various factors, including clinical outcomes, patient responses, and improvements needed in membrane technology.
Clinical Efficacy
Clinical efficacy refers to the effectiveness of hemodialysis membranes in real-life medical conditions. Many studies have focused on the ability of these membranes to provide adequate clearance of small and middle-sized solutes, such as urea and creatinine. Research shows that high-flux membranes can remove larger molecules more effectively than low-flux membranes, contributing to better patient outcomes.
Factors impacting clinical efficacy include:
- Membrane type: Differences between cellulose-based and synthetic membranes significantly affect solute clearance.
- Blood flow rates: Higher flow rates may improve the efficiency of solute removal, but this must be balanced against patient comfort.
- Dialysis duration: Longer dialysis sessions can result in better solute clearance. However, the patient’s overall health must be assessed to determine the appropriateness of extended sessions.
Understanding these variables allows healthcare professionals to customize treatment plans for individual patients, enhancing overall renal care.
Biocompatibility and Safety
The biocompatibility of hemodialysis membranes is another essential aspect to assess. It refers to how the material interacts with blood components. A biocompatible membrane minimizes adverse reactions, such as inflammation or thrombus formation, ensuring a safer dialysis process.
Key considerations in evaluating biocompatibility include:
- Material composition: Synthetic materials generally offer better biocompatibility than traditional cellulose membranes. For instance, polyethersulfone (PES) is often favored for its lower inflammatory response.
- Surface modification: Techniques, such as hydrophilization, enhance a membrane’s surface properties, reducing protein adsorption and subsequent inflammatory reactions.
- Long-term safety: Continuous monitoring during and after dialysis is essential to identify any potential long-term effects related to membrane use.
Performance evaluation, encompassing both clinical efficacy and biocompatibility, is vital for ensuring optimal patient outcomes. It also drives ongoing research and innovation in hemodialysis technology to meet the evolving needs of patients with renal failure.
"The key to improving hemodialysis therapy lies not just in the technology itself, but also in understanding how these technologies affect patient health and quality of life."
This comprehensive approach allows professionals to tailor hemodialysis treatments, ultimately enhancing patient care and safety.
Patient Considerations
Understanding patient considerations in hemodialysis is paramount. This segment emphasizes the diverse elements impacting patients undergoing this therapy. By tailoring the approach to each patient's needs, healthcare providers can enhance treatment efficacy and quality of life.
Hemodialysis Patient Population
The demographic that requires hemodialysis showcases significant variance. It includes patients with acute kidney injury or chronic kidney disease. Factors such as age, underlying health conditions, and lifestyle greatly influence treatment decisions. For instance, older individuals may have distinct physiological characteristics that necessitate careful selection of dialysis membranes to minimize risks.
Patients with complex comorbidities, such as diabetes or cardiovascular issues, also require customized approaches to ensure safe and effective treatment.
Additionally, patient education plays a vital role. Knowledge about their condition and treatment allows patients to engage actively in their care, thus improving compliance. Understanding the hemodialysis process also alleviates anxiety, fostering a sense of control over their medical journey.
"Patient engagement is key to successful hemodialysis therapy."
Factors Influencing Membrane Selection
Choosing the right membrane is critical in optimizing patient care. Various factors influence this decision:
- Patient’s Clinical Profile: The membrane selected should align with specific health conditions. For example, patients predisposed to clotting may warrant membranes that minimize thrombogenicity.
- Biocompatibility: Membranes must be well-tolerated by the body's immune system to prevent adverse reactions during treatment.
- Dialysis Type: The choice between high-flux and low-flux membranes should reflect the patient's hemodialysis regimen. High-flux membranes enhance the clearance of larger solutes, which might benefit certain patients.
- Age and Size: Membrane surface area may vary based on the patient’s size. This ensures adequate treatment without overloading smaller patients.
- Resource Availability: Practical considerations, such as the availability of specific membrane types in a clinical setting, also affect selection.
Clinical Applications
The clinical applications of hemodialysis membranes are pivotal in managing renal failure. These membranes play an essential role in filtering waste products, excess fluids, and toxins from the blood. Understanding their application is important for both health professionals and patients alike. The right choice of membrane can significantly influence treatment outcomes, enhancing the efficacy and safety of hemodialysis.
Key considerations in clinical applications include:
- Patient characteristics: Patient size, age, and overall health can affect the type of membrane used.
- Type of renal failure: Acute versus chronic conditions may require different membrane specifications.
- Treatment goals: Different clinical endpoints might necessitate varying membrane permeability and material.
The incorporation of advanced membrane technologies can also improve patient comfort and treatment efficiency, revolutionizing traditional dialysis practices.
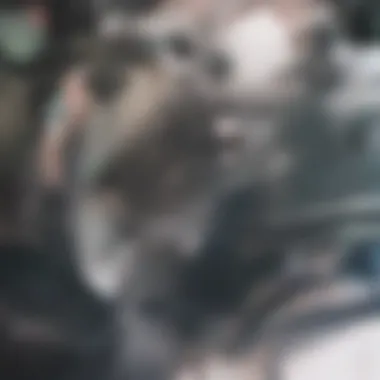
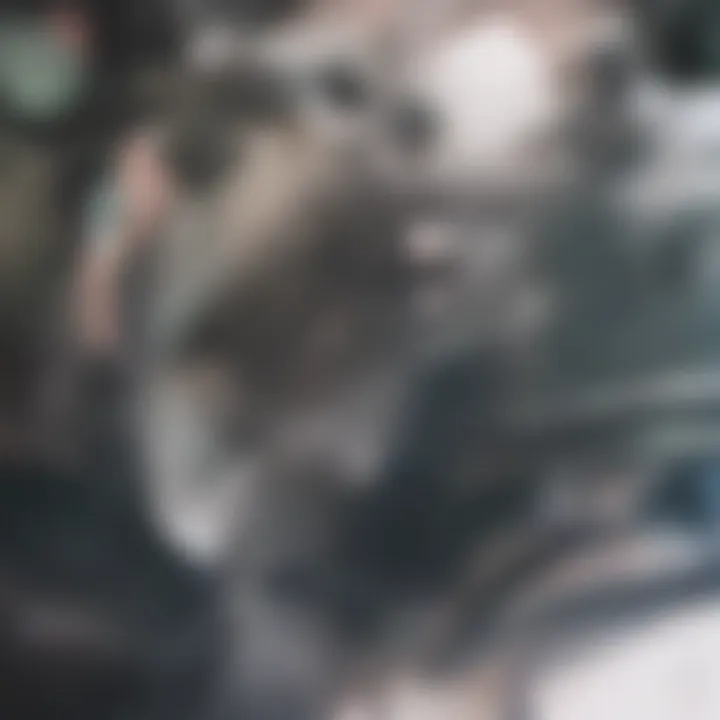
Advancements in Hemodialysis Membrane Technology
The advancements in hemodialysis membrane technology represent a critical area of research and development in renal replacement therapy. As kidney disease becomes more prevalent worldwide, the need for effective treatment options intensifies. Innovations in membrane design not only improve the efficiency of dialysis but also enhance patient comfort and safety. New materials and technologies aim to reduce the complications traditionally associated with hemodialysis, thus making the procedure more viable for a wider range of patients.
Innovations in Membrane Materials
Recent developments in membrane materials have focused on creating membranes that facilitate better solute removal and provide superior biocompatibility. Manufacturers are increasingly turning to synthetic polymers, which offer several advantages over traditional cellulose-based materials.
- Enhanced Selectivity: New synthetic membranes allow for targeted removal of toxins, improving the overall efficiency of the dialysis process.
- Lower Inflammatory Response: Biocompatible materials minimize the inflammatory response in patients, which is crucial for long-term dialysis.
- Durability and Lifespan: Improvements in the structural integrity of these materials ensure longer-lasting membranes, reducing the need for frequent replacements.
These innovations are essential for personalizing and optimizing treatment. For example, a polyethersulfone (PES) membrane has become a popular choice due to its favorable performance characteristics. Its robust nature allows for better filtration rates, contributing to quicker and more effective dialysis.
Emerging Nanotechnology Applications
Nanotechnology is making its mark in the field of hemodialysis by offering advanced solutions to existing challenges associated with membrane technology.
- Nanostructured Membranes: These membranes exhibit unique properties that significantly improve their performance. They exhibit better permeability and selectivity for different solutes.
- Antimicrobial Coatings: Incorporating nanoparticles relevant for antimicrobial applications can reduce the risk of infections during dialysis, a real concern for patients.
- Smart Membranes: Research is also exploring membranes that can respond to changing chemical environments. Such membranes may adapt to enhance clearance rates of specific substances based on patient needs.
The integration of nanotechnology holds promise for revolutionizing hemodialysis. Not only do these advancements aim to improve the efficacy of the treatment, but they also strive for enhanced quality of life for patients undergoing regular dialysis.
"Advancements in hemodialysis membrane technology are not just about better materials; they represent a holistic response to challenges faced by patients."
Regulatory and Quality Standards
Regulatory and quality standards play a critical role in ensuring safety and effectiveness in hemodialysis membranes. These standards establish the criteria that these vital components must meet to be deemed acceptable for clinical use. The integrity of these guidelines influences patient outcomes directly, making the understanding of them essential for healthcare professionals and researchers.
The focus on regulatory standards affects various stages of the development and manufacturing of membranes. It aids in setting benchmarks for membrane performance, biocompatibility, and manufacturing practices. Compliance with established regulations helps in minimizing complications associated with hemodialysis, such as infections or adverse reactions. In short, adherence to these standards is paramount for safeguarding patient health.
FDA Guidelines for Hemodialysis Membranes
The Food and Drug Administration (FDA) establishes comprehensive guidelines that hemodialysis membranes must follow. These guidelines encompass everything from material selection to testing protocols and post-market surveillance. The FDA evaluates not just the mechanical properties of the membranes, but also their biocompatibility.
Some key elements of FDA regulations include:
- Pre-market Notification: Manufacturers must submit a 510(k) application, demonstrating that their product is as safe and effective as existing devices.
- Quality System Regulations: Manufacturers need to adhere to stringent quality management standards during production.
- Product Recalls: In case of unforeseen issues, the FDA has protocols for the recall of non-compliant products.
These regulations ensure that all hemodialysis membranes on the market have undergone rigorous evaluation. By adhering to FDA guidelines, manufacturers can contribute to better overall outcomes in kidney disease management.
International Standards Organizations
Apart from FDA guidelines, various International Standards Organizations operate in collaboration with national bodies to formulate standards for hemodialysis membranes. An example is the International Organization for Standardization (ISO), which develops standards aimed at ensuring quality and safety on a global scale.
Such international standards cover:
- Testing Methods: Guidance on how to assess membrane performance under different conditions, ensuring consistency across products.
- Safety Criteria: Standards that help in determining acceptable levels of extractable substances from the membranes that could potentially lead to toxicity.
- Manufacturing Practices: Best practices that manufacturers should implement for quality assurance during production processes.
By acknowledging these international guidelines, manufacturers can expand their market reach while maintaining a commitment to high standards. This global perspective is vital for hemodialysis technology, providing a framework that promotes continuous improvement and innovation.
In summary, regulatory and quality standards are not merely procedural formalities but foundational elements that uphold the efficacy and safety of hemodialysis membranes. Understanding these standards is crucial for all stakeholders involved in renal care.
Culmination
The conclusion of this article holds paramount significance, as it synthesizes the critical elements discussed throughout regarding hemodialysis membranes. Membranes play a vital role in renal replacement therapy, directly impacting patient outcomes and quality of life. By addressing their types, materials, mechanisms, and advancements, this article provides essential insights for both practitioners and researchers in the field of nephrology.
Future Directions in Hemodialysis Research
Future research in hemodialysis membranes is essential for improving patient care. Innovations could focus on enhancing membrane biocompatibility. This includes reducing adverse reactions during dialysis procedures. Studies may also explore advanced materials that can increase efficiency in solute removal. Biologically inspired designs could replicate natural filtration processes, potentially transforming current practices. Moreover, ongoing investigations into the long-term impacts of different materials will be crucial for developing guidelines.
Some promising areas include:
- Smart membranes that can actively respond to changes in blood composition.
- Stem cell technology that explores regenerating damaged kidneys.
- Personalized dialysis approaches tailored to individual patient needs.
Final Thoughts on Membrane Optimisation
Optimizing hemodialysis membranes presents an opportunity to improve therapeutic outcomes. It is crucial to strike a balance between performance and biocompatibility. A well-optimized membrane can enhance the clearance of waste products while minimizing inflammatory responses. The ongoing challenge lies in addressing variability among patient populations, which can influence membrane selection.
Considerations when optimizing membranes include:
- Material selection aligned with patient-specific characteristics.
- Regular assessments of membrane performance and safety in clinical settings.
- Collaborations among scientists, clinicians, and industry to drive innovations.