A Deep Dive into the ELISA Test Methodology
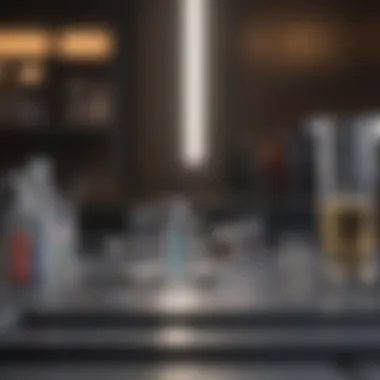
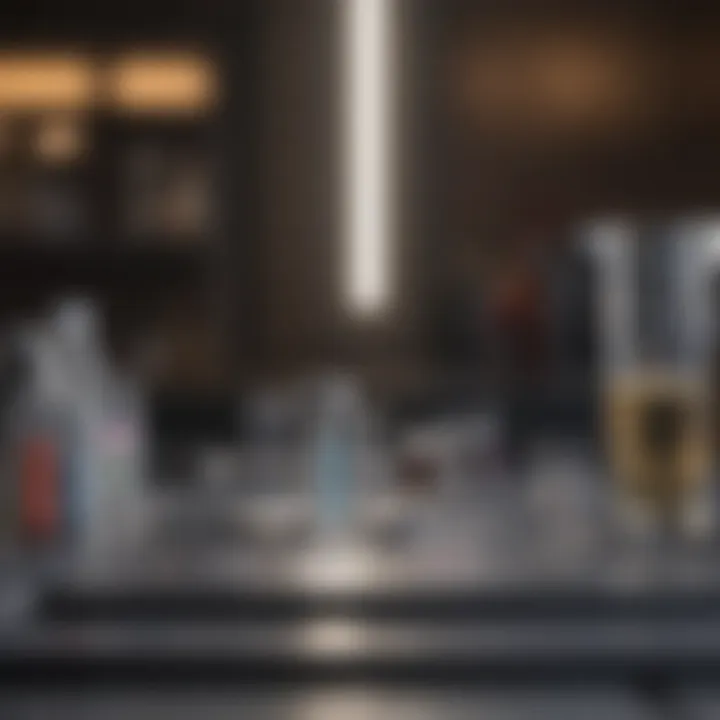
Intro
The ELISA test (Enzyme-Linked Immunosorbent Assay) has become a cornerstone in the laboratory for detecting and quantifying biomolecules. This powerful tool is used across various domains including clinical diagnostics, biotechnology research, and even in environmental assessments. But what makes this test so pivotal?
At its core, the ELISA method revolves around its ability to utilize antigen-antibody interactions to yield meaningful results. Understanding the workings of this test not only lays the groundwork for its application but also highlights its significance in modern scientific endeavors.
In this article, we explore the ELISA test in depth. From the foundational principles and various formats to the varied applications and the pros and cons, our journey will provide a holistic view. If you're a student, researcher, educator, or a professional navigating through the complexities of biomolecular detection, you will find this guide valuable.
Letās roll up our sleeves and dive into the key findings of this comprehensive overview.
Prologue to the ELISA Test
The ELISA test, an acronym for Enzyme-Linked Immunosorbent Assay, is a crucial analytical method used in various scientific fields. This introduction lays the groundwork for understanding how the ELISA test operates and its importance in clinical diagnostics and research.
By detecting and quantifying specific biomolecules, ELISA serves as a reliable tool that can provide insights into a range of conditions, helping professionals make informed decisions. Its accessibility and ease of use make it a staple in laboratories, ensuring it remains at the forefront of diagnostic tests.
Defining ELISA
To grasp the significance of the ELISA test, it is essential to clarify its definition and core components. The ELISA is designed to identify the presence of antibodies or antigens in a sample, utilizing the highly specific interaction between these biomolecules to produce quantifiable results.
In essence, an ELISA test typically involves:
- A sample containing the biomolecule of interest (like blood or serum).
- An antigen or antibody that is specific to the target biomolecule.
- An enzyme linked to the antibody that, when a substrate is applied, leads to a measurable color change.
The beauty of this test lies in its capability to offer both qualitative and quantitative analysis of these biomolecules, enabling a deeper understanding of biological processes.
Historical Context and Development
The development of the ELISA test traces back to the late 1970s, a period when researchers began to realize the potential of enzyme-linked antibodies. Pioneers in immunology were eager to find efficient ways of measuring biomolecules, leading to this innovative assay method.
In the early stages, the ELISA test was primarily used to detect viral infections and screen for antibodies. Over time, its applications expanded, finding utility in the detection of various diseases, allergen testing, and even in food safety. Such versatility highlighted its importance, transforming it into one of the go-to techniques in modern laboratories.
By understanding its historical backdrop, one can appreciate how far the ELISA test has come and how it continues to evolve, driven by advancements in technology and scientific understanding.
"The beauty of ELISA lies in its simplicity and adaptability, making it a cornerstone of laboratory diagnostics and research."
As we delve deeper into the principles and components of the ELISA test in the subsequent sections, the foundational knowledge gained from this introduction will serve to enhance the understanding of its mechanisms, applications, and limitations.
Principles of the ELISA Test
The principles of the ELISA test comprise the backbone of its functionality, allowing it to excel as a diagnostic and analytical tool across many fields. Understanding these principles is crucial for comprehending both its utility and limitations in real-world applications. The primary strength lies in its ability to detect specific biomolecules, such as proteins, hormones, or antibodies, in various samples. This specificity arises from the unique interactions between antigens and antibodies, a concept at the heart of immunological assays.
Mechanism of Action
The ELISA test operates on a well-defined mechanism that facilitates the quantification of targeted substances. It begins with the attachment of an antigen to a solid surface, typically a microtiter plate. Following this, any unbound sites on the plate are blocked to prevent non-specific binding. The sample containing antibodiesāproduced in response to the antigenāthen gets added. If the antibody of interest is present, it binds to the antigen on the plate. A secondary antibody, usually conjugated with an enzyme, is then introduced. This secondary antibody is designed to attach to the primary antibody, forming a complex.
Once this complex is formed, the next step involves the addition of a substrate that the enzyme can react with, generating a measurable signal, often colorimetric. The intensity of this signal correlates directly with the amount of antigen present in the original sample, which enables quantification.
Types of ELISA Techniques
The ELISA methodology branches out into several types, each tailored to meet specific needs and applications. Understanding these varieties sheds light on their respective advantages and areas of suitability.
Direct ELISA
Direct ELISA is characterized by a straightforward approach where the sample and an enzyme-linked antibody are added directly to the antigen-coated plate. The key characteristic of direct ELISA is its simplicity; there is no requirement for a secondary antibody. This often results in reduced time and complexity, making it a popular choice when rapid results are essential. However, its major drawback is the potential for lower sensitivity, mainly if the primary antibody exhibits modest binding affinity.
Indirect ELISA
In contrast, Indirect ELISA utilizes a two-step detection process involving a primary antibody and a secondary enzyme-linked antibody. This method amplifies the signal and thus improves the sensitivity of the assay. A noteworthy characteristic of Indirect ELISA is its flexibility; varying secondary antibodies can be employed depending on the target. While it often yields stronger signals and greater sensitivity compared to direct ELISA, it adds complexity to the procedure.
Sandwich ELISA
Sandwich ELISA stands out by requiring two different antibodies; one captures the antigen while the other, linked to an enzyme, quantifies it. This format excels when measuring larger proteins or complex mixtures, as the two antibodies must bind to the same antigen epitopes. The strength of Sandwich ELISA lies in its specificity and sensitivity; it reduces interference from other substances in a sample, making it a reliable choice for clinical settings. However, a limitation is the necessity for the capture antibody to be highly specific to the target antigen, which can restrict its applications.
Competitive ELISA
In Competitive ELISA, the target antigen from the sample competes with a labeled antigen for binding sites on a limited number of antibody molecules. The key characteristic of this format is that it measures the amount of antigen inversely; the more antigen in the sample, the less labeled antigen is bound. This assessment method is particularly beneficial when the sample contains low concentrations of the target. However, a trade-off exists, as this technique often has a steeper learning curve and may require specific calibration curves for accurate quantification.
"The ground rules for interpreting ELISA results hinge not just on the assay type, but also on the specific characteristics of the antigens and antibodies involved."
Each type of ELISA thus serves a unique niche, with trade-offs in terms of complexity, sensitivity, and specificity tailored toward particular applications.
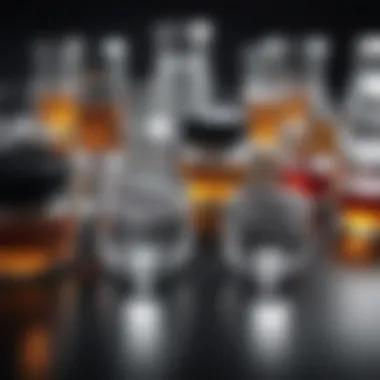
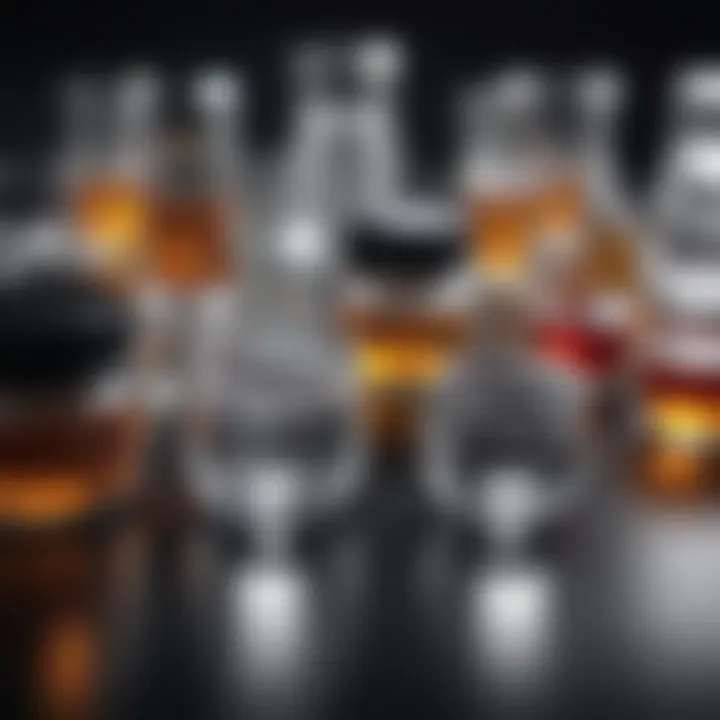
Components of the ELISA Test
The ELISA test, a staple in both lab and clinical settings, isn't just a simple procedure. Its efficacy hinges on several key components that work synergistically to ensure accurate results. Knowing these elements is crucial, as each plays a distinct role in the detection and quantification of specific biomolecules. The following sections will delve into the main components involved in the ELISA test: antigens and antibodies, enzymes and substrates, and plates and coating methods.
Antigens and Antibodies
At the heart of the ELISA test are antigens and antibodies. Simply put, antigens are the substances that the test aims to detectātypically proteins from pathogens, allergens, or hormones. On the flip side, antibodies are the body's response to such antigens; they bind to these foreign substances to neutralize them.
The specificity of this binding is what makes ELISA a powerful tool. For example, if you are attempting to detect the presence of a virus in a patientās serum, the test relies on antibodies that are tailored to bind exclusively to the viral proteins. If the sample contains the target antigen, it will latch onto the antibodies, leading to a measurable signal. This specificity is critical because it minimizes the risk of false positives.
From a practical standpoint, the source of antibodies is something to consider. While monoclonal antibodiesāproduced from a single clone of cellsāoffer high specificity, polyclonal antibodies, which are derived from multiple sources, provide a broader range of detection. Thus, selecting the right type of antibodies can significantly influence the accuracy and reliability of the test results.
Enzymes and Substrates
Next in line are enzymes and substrates, a dynamic duo that catalyzes the detection reaction in an ELISA test. Commonly, enzymes such as horseradish peroxidase or alkaline phosphatase are used. These enzymes facilitate the conversion of specific substrates into measurable products, which usually leads to a color change observable through spectrophotometry.
The interaction between the enzyme and the substrate produces a signal, with the intensity of the color correlating directly to the concentration of the target antigen. For instance, if you're using horseradish peroxidase, the substrate might turn from colorless to a vibrant hue when the enzymatic reaction occurs. This colorimetric change allows for straightforward quantification of the antigen in the sample.
However, one must keep in mind that the selection of substrates also influences sensitivity and specificity. The wrong match might lead to background noise, making it challenging to discern meaningful data. Thus, understanding how different enzymes interact with various substrates can help in optimizing ELISA performance.
Plates and Coating Methods
Last but not least, the choice of plates and coating methods plays a pivotal role in the design and success of an ELISA assay. Typically, these are polystyrene plates that allow for effective binding of the antigen or antibody. The surface of these plates can be treated to enhance binding capacity, making them even more functional for specific assays.
Coating involves attaching the target antigen to the plate, allowing for subsequent steps in the assay to unfold smoothly. The binding can be optimized by adjusting pH and ionic strength, among other factors, ensuring that the antigen remains available for detection without losing its structural integrity.
"A well-coated plate is fundamental to any reliable ELISA test. It not only enhances the binding of the proteins, but also aids in reducing the variability often seen in such assays."
Selecting the right assay design can make or break your experiment. The overall effectiveness of the ELISA hinges on understanding these components and how they interact within the broader framework of this versatile testing method.
ELISA Test Procedures
The procedures involved in the ELISA test form a critical backbone for obtaining accurate and reliable results. Each step of this process is carefully designed to ensure that the test maintains its high sensitivity and specificity. Understanding the intricacies of these procedures equips practitioners and researchers to conduct tests effectively while minimizing pitfalls. The quality of the samples, the conditions during incubation, and the methods employed for detection all play demonstrable roles in the outcome of the test.
Preparation of Samples
Preparation of samples is foundational to any good laboratory procedure, especially in the context of the ELISA test. The samplesāoften blood, serum, or plasmaāmust be collected and handled with care to avoid contamination. The integrity of the biomolecules sought after hinges on how well these samples are prepped.
For instance, certain antibodies may degrade if exposed to heat or prolonged periods in suboptimal conditions; therefore, maintaining a cold chain is vital. When preparing samples, practitioners should consider factors like dilution ratios and the nature of the sample itself. If the sample contains particulate matter or is cloudy, it might need centrifugation before it can be used in the assay. This ensures that the wells of the plates receive a homogeneous specimen, allowing for consistent results across the board.
In addition, itās worth noting that the quantification of analytes within the samples relies on precision. Standardization of the assay conditions, including buffer composition, pH, and ionic strength, will help maintain consistency throughout. Ultimately, effective sample preparation sets the stage for a smooth testing process, far reducing variabilityāa good practice that pays dividends in accuracy.
Incubation Process
The incubation process in ELISA is another vital step where the efficacy of the tests is put to the test, literally. After samples and reagents are added to the designated wells of the test plate, they must be incubated for a specific duration, usually around one to two hours for typical reactions. The environmental conditionsātemperature, presence of shaking, and timeāare meticulously controlled because they directly influence the binding affinity between the antigens and antibodies.
Itās important to follow the protocol specifics; some tests may require overnight incubation at lower temperatures, which may not be as intuitive at first glance. For example, if the antibody is particularly slow in reacting, a longer incubation could be beneficial. On the flip side, excessive incubation times may lead to nonspecific binding, inflating the actual results.
One common technique is to utilize a shaker to enhance mixing during the incubation phase. However, the intensity must be calibrated to avoid damaging sensitive components in the assay. Ultimately, this incubation phase aims to ensure all binding reactions reach an equilibrium, which is key to the reliability of the test outcomes.
Detection Methods
After the incubation process, the final step in the ELISA is the detection of the antigen-antibody complex formed. The selection of detection methods can greatly differ based on the type of ELISA being employed.
Common approaches include:
- Colorimetric Detection: where a chromogenic substrate is used, producing a measurable color change correlated to the amount of antigen present.
- Fluorescent Detection: makes use of fluorophore-labeled antibodies, where the intensity of fluorescence is measured. This method can be more sensitive than colorimetric approaches.
- Chemiluminescent Detection: another high-sensitivity method that emits light when substrates react. It is often faster and can be more sensitive than colorimetric methods.
The method of choice often hinges upon the specific requirements of the assay. For example, in high-throughput applications, where speed is essential, fluorescence or chemiluminescence may be preferable. As you might expect, thorough calibration and validation of the detection methods are non-negotiable. This ensures that the results obtained can be reliably interpreted.
The fidelity of the ELISA test is governed by the meticulous execution of its proceduresāfrom sample preparation to detection methodsāeach stage critical to producing dependable results.
Through adept understanding and execution of these procedures, researchers and clinicians can leverage ELISA's strengths while mitigating its inherent limitations.
Applications of ELISA
Understanding the myriad applications of the ELISA test is crucial, particularly as we navigate contemporary scientific landscapes. The versatility of ELISA allows it to play an essential role in various fields, from clinical diagnostics to food safety. This examination sheds light on how these applications contribute to our ongoing health and safety concerns, emphasizing their impact in real-world scenarios.
Clinical Diagnostics
In the realm of clinical diagnostics, ELISA tests are indispensable. They are often the backbone of many diagnostic frameworks, primarily used for the detection of antibodies in response to infections or diseases. For instance, when a patient shows symptoms suggestive of an infectious disease, an ELISA can swiftly ascertain the presence of specific antibodies. This not only helps in diagnosing the disease but also in monitoring the patient's healthcare journey.
The ability of ELISA techniques to accurately quantify biomolecules makes them particularly effective for monitoring therapy responses. For example, evaluating HIV patientsā immune responses through antibody detection can show how well a treatment plan is working. Furthermore, routine screenings for various pathogensāsuch as hepatitis or Lyme diseaseārely heavily on these tests because of their high sensitivity and specificity.
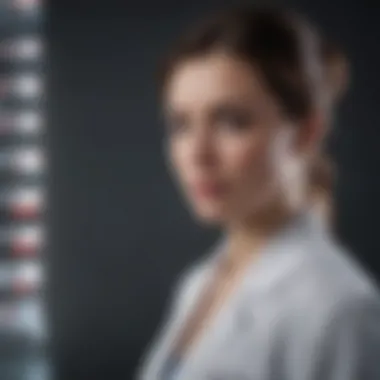
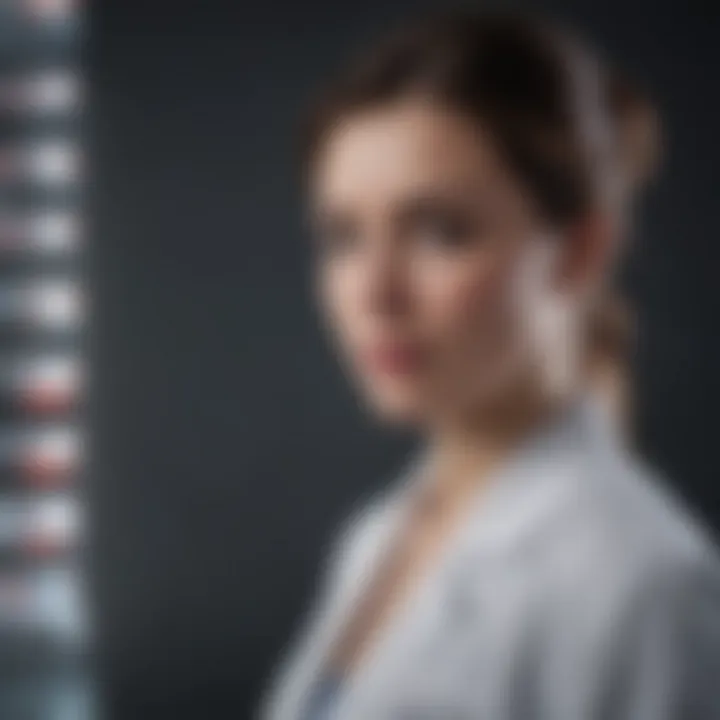
"ELISA stands at the crossroad of clinical science and patient care, integral to guiding treatment choices and assessing disease states."
Advantages in Clinical Use
- Rapid Result Processing: Tests can produce results in a matter of hours, which is critical in emergency situations.
- Ease of Use: Less complicated compared to some other techniques, allowing broader use in clinical laboratories.
- Cost-Effectiveness: Compared to other diagnostic methods, ELISA tests are relatively inexpensive, making them accessible for widespread use.
Research Contexts
In research settings, ELISA methodology sees no shortage of utilization. Scientists employ these tests to understand diseases at a molecular level, facilitating groundbreaking advances in fields such as immunology, virology, and oncology. For example, researchers may use ELISAs to explore immune responses by detecting specific antibodies elicited by vaccines or infections.
Moreover, ELISA can serve as a platform for developing novel drugs by quantifying target antigens in various assays. This ability to gather quantitative data is instrumental in preclinical research, influencing hypotheses in experimental designs. Translational research, which bridges laboratory discoveries and clinical applications, also favors the use of ELISA for validating findings, ensuring that laboratory results hold true in clinical scenarios.
Food Safety and Quality Control
Beyond clinical and research uses, the significance of ELISA extends into the food industryāa sector where safety cannot be compromised. Testing food products for contamination by allergens or toxins can happen via ELISA methodologies. This application is crucial since even trace amounts of certain allergens can lead to serious health repercussions.
For instance, the testing of peanuts or gluten in various products is essential to safeguard consumers who may have allergies or intolerances. Moreover, employing ELISA in detecting pesticide residues is becoming a common practice in food laboratories. These tests help maintain high safety standards within food supply chains, ensuring products are safe for consumption.
Why This Matters
The implications of ELISA in food safety are profound, not just from a compliance perspective but also for consumer confidence. Ensuring that food products are tested correctly can help avoid catastrophic repercussionsālike food poisoning outbreaksāwhich could tarnish public perception of food brands.
Advantages of Using ELISA
When it comes to diagnostic assays, the ELISA tests stand head and shoulders above many alternatives. Their unique attributes make them a go-to method in both clinical and research settings. The advantages of ELISA lie not just in its broad application but also in its efficiency and reliability.
Sensitivity and Specificity
One of the most striking features of ELISA is its high sensitivity. To put it simply, this means it can detect even minute concentrations of biomolecules. This is especially crucial in clinical diagnostics. For instance, early detection of diseases like HIV or cancer can significantly impact treatment outcomes. The ability to spot low levels of antigens or antibodies empowers clinicians to make informed decisions fast.
Moreover, specificity is equally vital. ELISA minimizes cross-reactivity with other substances, ensuring that the results primarily reflect the target biomolecule. This accuracy is essential in research settings where precision is key to valid conclusions. In practical terms, if you were testing for a viral infection, the ELISA would help you avoid false positives that might arise from unrelated antibodies. Achieving a balance between sensitivity and specificity is often a tightrope walk, yet ELISA has perfected this.
Scalability and Adaptability
Another major boon of ELISA is its scalability. Whether you are running a single test or thousands, the methodology can easily adapt to your needs. In larger laboratories, for example, multiple wells on a plate can be used simultaneously, allowing a high-throughput screening of samples. This aspect is particularly advantageous during outbreaks or mass screening events, where efficiency is critical.
It is not just about the number of tests; adaptability is also key when discussing ELISA. The protocol can be tailored to accommodate various sample types, including serum, plasma, or even tissue extracts. This flexibility means that researchers can modify existing assays or develop new ones tailored to specific applications. For example, in food safety, ELISA can be customized to detect specific allergens in consumables, catering to regulatory standards.
"The beauty of ELISA lies in its simple yet effective method that can cater to varied scientific fields and practices," says a renowned bioanalytical researcher.
In summary, the advantages of using ELISA offer a compelling reason for its widespread adoption. Not only does it provide sensitive and specific results, but its scalability and adaptability ensure it remains relevant in the ever-evolving landscape of scientific research and diagnostics. Regardless of the domainābe it clinical diagnostics, food safety, or researchāthe benefits of ELISA are undeniably pronounced.
Limitations of ELISA
While the Enzyme-Linked Immunosorbent Assay (ELISA) is a prominent tool in various fields, it is not without its limitations. Understanding these shortcomings is crucial for anyone using the assay, whether in research or clinical settings. By recognizing these limitations, practitioners can make more informed decisions and implement additional measures to mitigate them.
Potential for False Positives
False positives in ELISA can present significant challenges. These are instances where the test indicates the presence of a biomolecule when it is not actually there. Several factors can contribute to this issue:
- Cross-reactivity: When antibodies used in the assay recognize similar but unrelated antigens, it can lead to incorrect conclusions.
- Sample contamination: A sample might get contaminated during the testing process, which can result in misleading results.
To minimize the chances of false positives, researchers often employ controls. For instance, including known negative samples in the assay allows for a better gauge of the test's specificity. However, even with stringent controls, the risk remains, which can complicate the interpretation of results.
Variability in Results
Variability is another critical limitation associated with the ELISA technique. Different batches of reagents, slight changes in procedural protocols, or even minor variations in laboratory conditions can affect results. This variability can be broken down further into two main aspects:
- Inter-assay variability: Differences observed when the same sample is tested using different ELISA runs. Factors influencing this can be calibration differences and variations in reagent quality.
- Intra-assay variability: Variability shown within a single run. This often stems from pipetting discrepancies or fluctuations in incubation times.
To tackle this variability, researchers may use standard curves and replicate samples in their assays. This helps in understanding the range of expected results and allows for a clearer analysis of data. Still, even carefully controlled experiments may experience variability, leading to questions about the reproducibility of results.
Understanding these limitations is equally as important as recognizing the applications of ELISA. It shapes how results are interpreted, and how further investigations are pursued.
In summary, while the ELISA test is an indispensable tool, acknowledging its limitations allows for better experimental designs and more precise outcomes. By being aware of issues like false positives and variability, professionals can enhance the reliability and validity of their findings.
Comparative Methods to ELISA
When it comes to biomolecular detection, various assays are employed alongside the ELISA test. Understanding these comparative methods allows researchers to choose the best approach for specific applications. Each method has unique strengths, applications, and concerns that can significantly influence results, making it essential to explore them holistically.
Western Blot
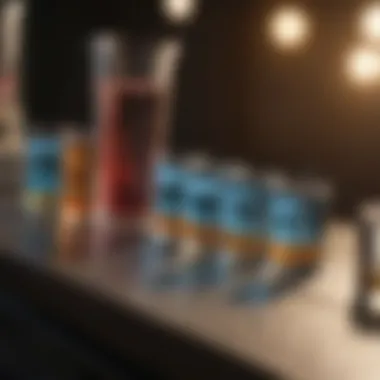
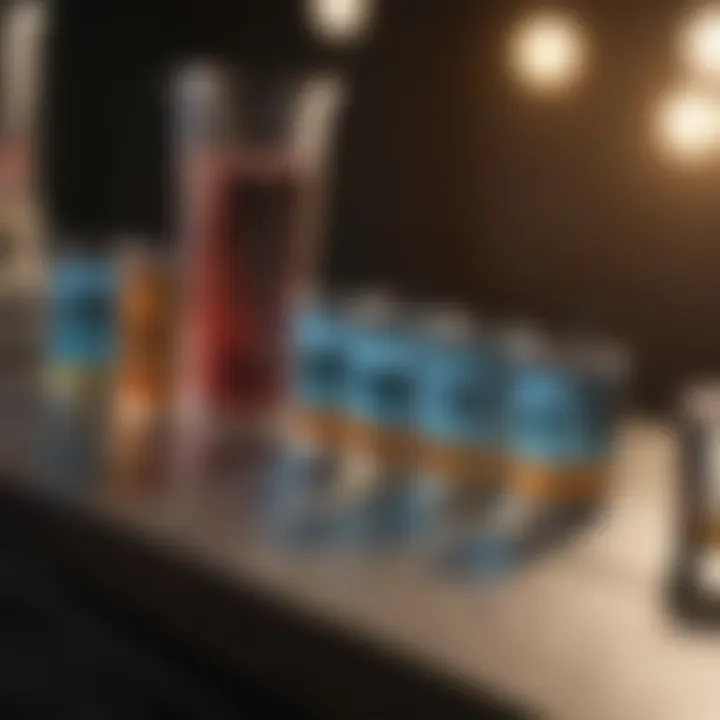
Western blotting has been a staple in the world of molecular biology since its introduction. This method primarily focuses on the detection of specific proteins in a sample. A typical Western blot involves gel electrophoresis, where proteins are separated based on their size. After the separation, they are transferred to a membrane and probed with antibodies specific to the target protein. This process offers high specificity and can be used to confirm the presence of a protein, providing both qualitative and quantitative results.
Key Points about Western Blot:
- Specificity: Antibodies used are highly specific, allowing researchers to confirm the presence of a protein with great confidence.
- Multiple Targets: It can differentiate between similar proteins, which is particularly useful in cases where protein isoforms are being studied.
- Quantification Challenges: While Western blots can indicate protein levels, quantification often requires careful calibration against standards, making it less straightforward than ELISA.
In the context of protein analysis, Western blotting serves as a powerful complementary tool to ELISA, particularly when further confirmation of antibody specificity is needed.
Radioimmunoassay (RIA)
Radioimmunoassay is another method that predates ELISA and was primarily developed for measuring hormone levels. RIA uses radioactively labeled antibodies or antigens, wherein the binding of the labeled molecule to its target quantifies the unlabeled ones in the sample. Despite the accuracy it can provide, the radioactivity raises safety concerns and regulatory issues, limiting its desirability in many labs today.
Considerations for RIA:
- Sensitivity: RIA is highly sensitive and can detect low levels of substances in a sample.
- Safety Risks: Handling radioactivity requires strict safety protocols, often discouraging its utilization in labs without extensive safety measures.
- Regulatory Hurdles: Disposal of radioactive materials brings regulatory attention, complicating the practicality of this method in many clinical settings.
Given these attributes, while RIA offers valuable insights, its practical drawbacks have led many researchers to opt for non-radioactive methods like ELISA, especially when safety and efficiency are paramount.
Lateral Flow Tests
Lateral flow tests, often seen in home pregnancy tests, represent a different genre of diagnostic assay. These tests offer an extremely user-friendly format for detecting specific antigens or antibodies. A liquid sample travels along a test strip, and positive results are signified by the appearance of lines.
Strengths of Lateral Flow Tests:
- Rapid Results: They provide results within minutes and do not require elaborate equipment or training.
- Field Usage: These tests are ideal for point-of-care diagnostics where laboratory infrastructure is absent.
- User-Friendly: The simplicity in operating these tests makes them accessible to the general public, reducing the need for professional handling.
While lateral flow tests are less quantitative than ELISA and might lack the sensitivity required for some applications, their speed and ease make them indispensable for rapid screening scenarios.
In summary, various methodologies like Western blot, RIA, and lateral flow tests offer distinct advantages and shortcomings. Recognizing when to utilize each method, based on factors such as specificity, ease of use, and quantitative needs, is crucial in the laboratory setting.
Future Directions in ELISA Research
The ELISA test has seen vast applications and improvements over the years, yet the horizon holds even greater promise. Advancements in technology and methodology are paving the way for enhanced performance and broader applications of the ELISA test in various domains. Understanding these future directions not only highlights the evolving landscape of biomedical research but also underscores the potential for developing more precise, quicker, and cost-effective diagnostic tools. As the scientific community continues to innovate, the incorporation of emerging technologies and integration with other assays is likely to redefine the ELISA testās role in diagnostics and research.
Emerging Technologies
In the landscape of lab diagnostics, emerging technologies such as microfluidics, nanotechnology, and advanced data analytics are on the rise. These innovations open new doors, allowing for more efficient and sensitive detection methods.
- Microfluidics: The poetry of small-scale fluid manipulation brings forth a new way of conducting assays. By using chips that handle minute quantities of liquids, microfluidics enables high-throughput screening and reduces reagent consumption, making ELISA tests more economical and environmentally friendly.
- Nanotechnology: Nanosized particles enhancing signal amplification can dramatically increase the sensitivity of ELISA tests. For example, gold nanoparticles, known for their optical properties, might be used as labels to boost the assay's detection limits significantly.
"The convergence of nanotechnology and ELISA can potentially lead to diagnostic tests that not only detect but also quantify analytes at the single-molecule level."
- Advanced Data Analytics: Modern data analytics tools, including machine learning and artificial intelligence, have excellent potential for simplifying interpretation processes. By integrating these technologies, the ELISA can transition to automated platforms that can provide analysis in real-time and with unprecedented accuracy.
Integration with Other Assays
Integration with other assays is a noteworthy trend shaping the future of ELISA research. Combining the strengths of ELISA with other testing methodologies can greatly enhance diagnostic capabilities. Consider the following approaches:
- Hybrid Assays: Combining ELISA with techniques like PCR (Polymerase Chain Reaction) can create hybrid assays that provide more comprehensive information about biomarkers. This integration offers both qualitative and quantitative data, invaluable for diagnostic precision, especially in infectious disease detection.
- Multiplexing Technologies: The ability to measure multiple biomarkers simultaneously is a great leap forward. By employing multiplexing technologies, ELISA can contribute to broader panels that assess various diseases from a single sample, reducing the workload and increasing the throughput of laboratory workflows.
- Point-of-Care Testing: Merging the ELISA format with rapid tests can facilitate point-of-care diagnostics. Devices that combine ELISA principles with lateral flow technology may offer quick, easy-to-use tests suitable for use outside lab settings, such as rural health clinics or emergency situations.
Culmination
In wrapping up this article on the ELISA test, it's crucial to underscore its paramount role in various scientific and clinical arenas. The significance of understanding this testing method cannot be overstated as it bridges a gap between laboratory capabilities and real-world applications. The ELISA test not only stands as a reliable tool for biomolecule detection but also exemplifies advancements in diagnostic methodologies.
Summarizing Key Points
An effective conclusion should encapsulate the core takeaways from our exploration:
- Fundamental Principles: The ELISA (Enzyme-Linked Immunosorbent Assay) operates on the principles of antigen-antibody interactions, serving as a robust method for quantifying specific substances in a sample.
- Diverse Applications: Its versatility shines through its use in clinical diagnostics, research contexts, and even in ensuring food safety.
- Advantages: Among its many advantages are its high sensitivity and specificity, which set it apart from other assays, making it invaluable in precise detection scenarios.
- Limitations: However, potential drawbacks like false positives and result variability do merit attention, emphasizing the importance of quality control.
- Future Directions: With emerging technologies and integration efforts alongside other methods, the ELISA test seems poised for further enhancement.
Final Thoughts on ELISA
In retrospect, the ELISA test is more than just a procedure; itās a critical element in the broader context of scientific discovery and clinical performance. By grasping its complexities and potential limitations, professionals can make informed decisions regarding its application, ensuring that the data collected is both reliable and actionable. As science continues to evolve, so too will the methodologies we use, and the ELISA test will undoubtedly remain a cornerstone of biomolecular detection.
Citing Key Research Articles
In the realm of scientific discourse, references hold a paramount importance. They not only lend credibility to the claims made within an article, but also act as a bridge for readers who wish to delve deeper. When it comes to understanding the ELISA test, citing key research articles forms the backbone of the discussion. These articles encompass a variety of studies and reviews that document the evolution, advancements, and applications of the ELISA methodology.
First and foremost, robust referencing allows for traceability. Readers can follow the trail of scientific reasoning and evidence back to its origins. This is particularly significant in the context of the ELISA test, where the methodologies and outcomes can vary widely. By consulting original studies, one can discern how different experiments have shaped the current understanding and utilization of ELISA in fields ranging from clinical diagnostics to environmental monitoring.
Moreover, high-quality references enrich the academic rigor of the article. They provide the necessary context and support for the claims made, enhancing the overall trustworthiness of the information. For instance, a groundbreaking paper on the sensitivity of ELISA tests in early disease detection not only informs the reader but also illustrates the ongoing relevance and evolution of the subject matter.
Additionally, references serve as a guide for further exploration. A well-researched article often points to seminal works or current innovations, allowing readersāwhether students, researchers, or educatorsāto pursue their own inquiries. This can lead to deeper insights into specific applications of the ELISA test, such as its role in monitoring therapeutic responses or its usage in food safety testing.
"References are not just a list of articles; they are the conversational threads that connect the tapestry of scientific knowledge."
Finally, itās crucial to consider the diversity of sources when citing references. An effective bibliography should include peer-reviewed research articles, authoritative reviews, and even healthcare practice guidelines. For instance, referencing a clinical guideline drawn up by a reputable organization can help in understanding the practical implications of ELISA tests in patient management scenarios.
In a nutshell, thorough referencing in articles concerning the ELISA test embraces not only the need for academic integrity but also facilitates a richer understanding of the topic. It helps to connect the dots for the audience and paves the way for future inquiries into this essential assay.