Understanding Electrodialysis: Principles and Uses
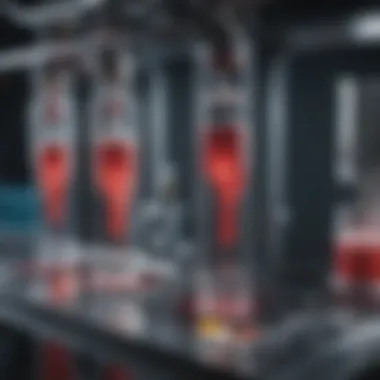
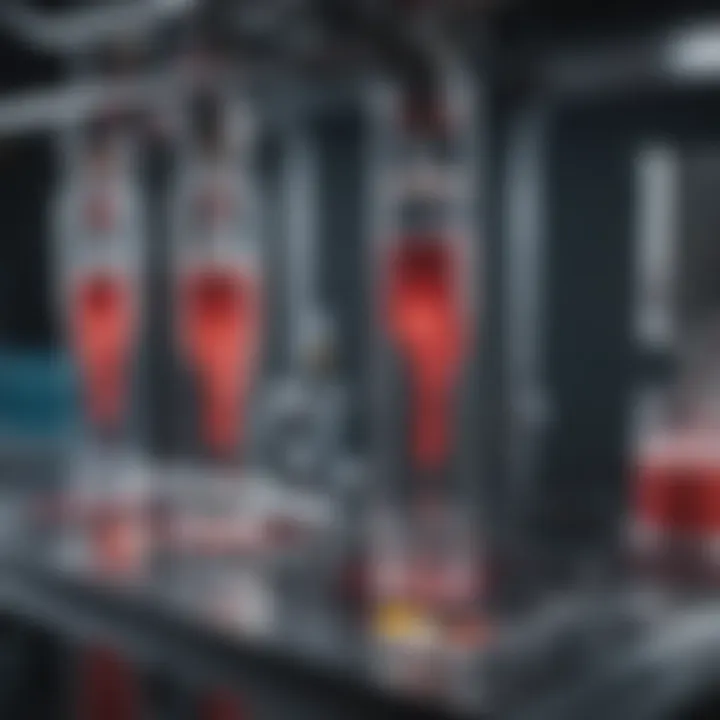
Intro
Electrodialysis stands as a cornerstone in the realm of electrochemical separation techniques. With the global demand for clean water on the rise, this method emerges as a beacon for addressing pressing issues in water desalination, chemical processing, and wastewater treatment. In simple terms, electrodialysis utilizes electric fields to move ions through selective membranes, thereby separating charged particles from a solution. This process has caught the attention of scientists, engineers, and industries alike, making it a hotbed of research and development.
As we delve into this topic, we will cover essential principles and operational mechanisms. We will explore various types of membranes and their crucial roles in the process. Additionally, the discussion will reflect on recent advances in technology that have notably improved the efficiency and effectiveness of electrodialysis systems.
Moreover, we’ll consider the obstacles this technology faces, such as scaling and fouling. Understanding these challenges is pivotal for paving the way forward. At the end of the journey, we aim to provide not only a thorough narrative but also insights into potential future applications in diverse fields.
Through this lens, let's transition into our key findings.
Key Findings
In reviewing the landscape of electrodialysis, several findings stand out.
Major Results
- Efficiency in Desalination: Electrodialysis achieves high water recovery rates, especially when contrasted with traditional methods like reverse osmosis. Studies indicate impressive recovery levels of around 75-90% in optimal conditions.
- Cost Effectiveness: With recent advances in materials and technology, the operating costs have dropped. For instance, utilizing energy-efficient ion-exchange membranes can cut energy expenses significantly.
- Environmental Benefits: By reducing chemical additives traditionally needed in water treatment processes, this technique showcases an eco-friendlier approach.
Discussion of Findings
The ability of electrodialysis to offer substantial water desalination and treatment capabilities cannot be overstated. The reduction in operational costs coupled with improved system efficiencies presents a compelling case for increased implementation in both industrial and municipal applications. Moreover, the lower carbon footprint associated with this method signals a shift toward more sustainable practices in processing.
However, while these findings are promising, there are hurdles that require attention. Fouling, for one, can lead to decreased performance over time, necessitating periodic maintenance or innovative solutions.
The advancement of materials science plays a pivotal role here. As new ion-selective membranes come into play, one can foresee even broader applications extending beyond water treatment. The potential adaptation in fields such as pharmaceuticals and food processing is ripe for exploration.
With this foundation, the next section will focus on the methodology underpinning current research in electrodialysis, linking practical applications to theoretical frameworks.
Prolusion to Electrodialysis
In a world where water scarcity and environmental concerns are rising to unsettling heights, understanding electrodialysis has become incredibly important. This technique is pivotal in various sectors, facilitating processes like water desalination and wastewater treatment. Its significance is not merely academic; it has real-world applications that can impact millions of lives. Without a doubt, navigating through the principles and operations of electrodialysis provides a clearer picture of how industrial and research communities work towards sustainable solutions for resource management.
Electrodialysis represents a fascinating confluence of chemistry and engineering, where ions dance across selective membranes under the influence of an electric field. This method has shown itself to be particularly advantageous in extracting salts and other contaminants from solutions, offering an efficient alternative to traditional filtration methods. Moreover, as technology advances, so do the possibilities for electrodialysis, making its study essential for future advancements.
Here’s a closer look at some elements worth considering when diving into electrodialysis:
- Efficiency: Electrodialysis operates with lower energy requirements than many other desalination techniques, making it an appealing choice for large-scale applications.
- Versatility: With a wide range of applications, from purifying drinking water to recovering valuable chemicals from industrial waste, its reach is extensive.
- Scalability: Whether large or small-scale, electrodialysis can be adapted to meet various production needs, allowing for its customization in diverse settings.
"The advancement in electrodialysis technology signifies not just a step forward for the chemical processes, but a leap towards sustainable environmental practices."
Definition and Scientific Background
Electrodialysis can be defined as an electrochemical separation process that utilizes selective ion exchange membranes to transport ions across the membranes driven by an electric potential. At its core, this process hinges on the principles of electrochemistry, where ions migrate toward electrodes. The result is a concentration gradient that facilitates the separation of ions from solutions.
Scientific studies explore the design and behavior of electrodialysis systems, focusing on how molecular charge plays a significant role in ion transport. Understanding these fundamentals is critical for engineers aiming to develop more efficient systems. The underlying science encompasses a blend of physics, chemistry, and fluid dynamics, providing a holistic view of how separation happens at a molecular level.
Historical Development
The history of electrodialysis traces back to the mid-20th century when research and experiments began to blossom. Early methods focused on simple setups that paved the way for more advanced designs. The concept emerged as scientists sought effective solutions to rising freshwater issues, leading to significant developments in membrane technology.
By the 1970s, electrodialysis grew into a commercial enterprise, with the development of specific membrane types that allowed for more efficient ion exchange. Over the decades, further refinements and innovations have vastly improved its applicability and performance, making it a valuable asset in addressing global challenges. Today, as we witness ongoing research and technological advancements, it's clear that electrodialysis has a bright future in remedying water scarcity and pollution.
In summary, understanding electrodialysis is essential not just for grasping a key electrochemical technique but also for appreciating its far-reaching impact on many industries. With the scientific backdrop and historical evolution laid out, we are better positioned to delve deeper into the principles, components, and future directions of this remarkable technology.
Basic Principles of Electrodialysis
Importance of Basic Principles of Electrodialysis
Understanding the fundamental principles of electrodialysis is vital for grasping how this separation technology operates effectively. It lays the groundwork for exploring its varied applications, from water desalination to wastewater treatment. This section addresses the intricate details of how electrodialysis capitalizes on electrochemical processes to achieve ion transport and separation. By looking closely at these principles, one can appreciate not only the technology’s mechanisms but also its practical implications in our increasingly industrialized world.
Electrochemical Fundamentals
Electrodialysis depends heavily on electrochemical principles. In its core, this process revolves around the movement of ions in response to an electric field. When a voltage is applied, cations are attracted to the negatively charged electrode (cathode), while anions move towards the positively charged electrode (anode). This selective ion movement occurs through ion exchange membranes, which only allow specific ions to pass through, effectively filtering them out.
To put it plainly, if you hook up a simple battery to two electrodes submerged in a saltwater solution, you’ll notice ions start to migrate. This migration is not just a random shuffle; it is guided by the electric potential created. Understanding this ion behavior is crucial, as it directly impacts the efficiency and effectiveness of electrodialysis systems.
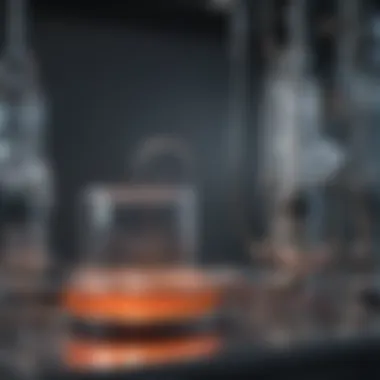
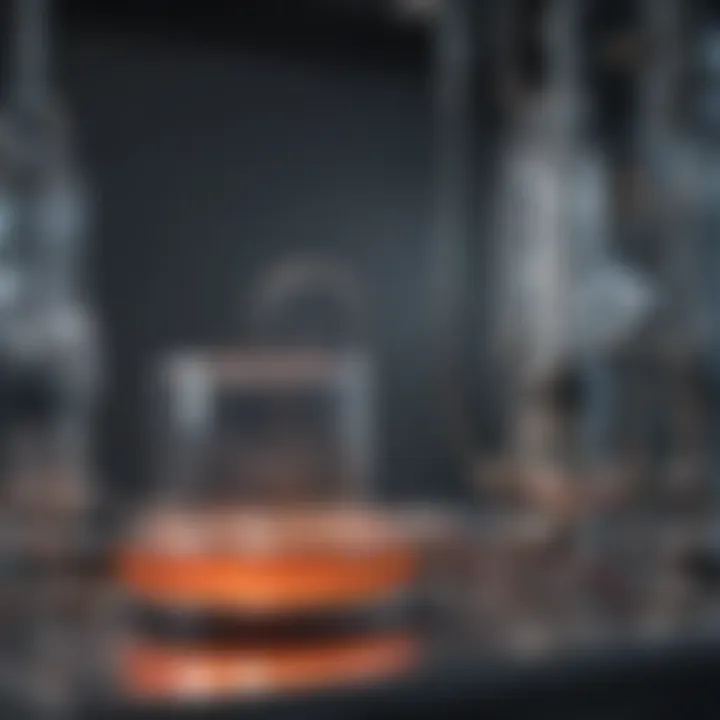
Ion Transport Mechanisms
Ion transport in electrodialysis is governed by a set of dynamic mechanisms. Two principal processes are often highlighted: diffusion and electrophoresis. Diffusion is the tendency of ions to move from a region of higher concentration to one of lower concentration, seeking equilibrium. On the other hand, electrophoresis is the motion of charged particles within a fluid in response to an electric field. It's this interplay—where diffusion battles against electrophoretic forces—that dictates the rate and efficiency of ion removal.
Additionally, the characteristics of the ion exchange membranes greatly influence these mechanisms. Membranes can be categorized into selective barrier types, ensuring that only ions of certain charges pass through while blocking others. This selectivity is critical for applications ranging from reclaiming valuable materials in chemical processes to purifying drinking water.
Role of Electric Field
The electric field is the driving force behind the phenomenon of electrodialysis. Its role is twofold: it not only initiates ion movement but also controls it. The strength of the electric field determines how quickly ions migrate and influences overall current efficiency.
When discussing the electric field, one must consider its uniformity within the electrodialysis cell. A consistent field can lead to a more predictable and efficient ion transport, enhancing the overall effectiveness of the process. Conversely, fluctuations can lead to uneven ion distribution and potential losses in efficiency. Thus, maintaining an optimal electric field is crucial for maximizing separation outcomes.
In summary, the basic principles underlying electrodialysis not only define its operational capacity but also spotlight areas for innovation and enhancement. Understanding these principles equips professionals with the knowledge to develop more efficient systems and strategies for a host of applications.
Components of Electrodialysis Systems
Electrodialysis systems are the backbone of this electrochemical technology, and understanding their components is vital for grasping its applications and efficiencies. These systems fundamentally consist of electrodialysis cells and various types of membranes, each playing a unique role in the separation processes. The performance, scalability, and effectiveness of electrodialysis technology largely hinge upon these components.
Electrodialysis Cells
Electrodialysis cells are where the magic happens. They are typically structured with alternating layers of anion and cation exchange membranes. When an electric current is applied, positively charged ions move towards the cathode, while negatively charged ions migrate towards the anode. This movement of ions creates a concentration gradient, facilitating the removal or recovery of ions from solutions. A crucial aspect of these cells is their design; they must ensure minimal resistance to ion flow, thereby maximizing efficiency. The arrangement of membranes within these cells can often determine the operational efficiency and the energy consumption required for the process.
Membrane Types Used
Meshing the right membranes into electrodialysis systems is not just a matter of choice; it is a fundamental decision that impacts the entire operation. Below are some key membrane types integral to these systems:
Anion Exchange Membranes
Anion exchange membranes play a pivotal role in the separation process, specifically allowing negatively charged ions to pass through while obstructing positive hordes. One of the defining characteristics of anion exchange membranes is their selectivity. This selectivity allows for targeted ion removal from a solution. They are often made from quaternary ammonium groups, which give them strong affinities for negatively charged ions. A significant advantage of using anion exchange membranes is their ability to operate effectively at lower pH levels, which can be a game changer in certain chemical environments. However, they can be susceptible to fouling by organic compounds in wastewater, which may necessitate additional maintenance.
Cation Exchange Membranes
On the flip side, cation exchange membranes are designed to allow positively charged ions to traverse while hindering negative ions. The key characteristic of cation exchange membranes is their positively charged functional groups, typically sulfonic acid (-SO3H). This specific property makes them suitable for scenarios requiring the removal of positive ions like sodium and calcium ions from saline solutions. A unique feature of these membranes is that they can tolerate a range of temperatures and pH levels, making them quite adaptable. However, they tend to be affected by high concentrations of multivalent cations, which could reduce their efficiency over time.
Composite Membranes
Composite membranes are a blend of both anion and cation exchange materials, engineered to enhance performance and provide versatility. Their construction often combines the strengths of both types, making them beneficial in more complex applications. The outstanding characteristic of composite membranes is their multilayer structure that helps optimize ion transport and reduce energy consumption. This structure allows them to withstand more extreme environmental conditions than their single-material counterparts. Still, they can be more expensive to produce and might face challenges related to membrane stability under certain operating conditions.
"The efficiency and adaptability of electrodialysis systems depend on the choice of membranes, where each type brings its own unique benefits and challenges to the electrochemical table."
In summary, the components of electrodialysis systems significantly dictate how effective and efficient the processes can be. From the construction and functionality of electrodialysis cells to the selection of appropriate membranes, every detail counts in optimizing this valuable technology.
Operational Modes of Electrodialysis
Understanding the different operational modes of electrodialysis is crucial for optimally applying this technology in various practical scenarios. Each mode has its unique benefits and considerations that can greatly influence outcomes in terms of efficiency, effectiveness, and overall sustainability. This section will explore the primary modes available, delving into continuous flow and batch processing, comparing their operational characteristics, and presenting their respective advantages.
Continuous Flow Electrodialysis
Continuous flow electrodialysis involves a constant movement of the feed solution through the electrodialysis cell, allowing a steady and uninterrupted process. This mode effectively separates ions while the solution continuously cycles through the system.
The continuous flow setup carries several advantages:
- Efficiency: Because the process operates without interruption, it can handle large volumes of water or solution, making it ideal for industrial applications.
- Real-Time Processing: You can monitor the separation in real-time, allowing for immediate adjustments to maintain optimal performance.
- Reduced Downtime: Continuous operations minimize the downtime usually required for cleaning and maintenance, resulting in higher productivity.
However, it’s not without challenges. Membrane fouling can occur more rapidly due to sustained flow, which might require more frequent cleaning cycles. Moreover, designs for effective flow and separation need to be well thought out to prevent short-circuiting, which would undermine the efficiency.
Batch Mode Electrodialysis
Batch mode electrodialysis, on the other hand, operates in distinct cycles. In this mode, a fixed volume of solution is processed at a time, which is particularly useful in situations where the quantities of the feed are smaller or irregular. Once a batch completes, another batch can be introduced.
The notable elements of batch mode include:
- Flexibility: Particularly suitable for variable feed quantities, it allows for adaptability depending on available resources. It’s very effective for research purposes and small-scale industrial applications.
- Control Over Parameters: Users can finely tune operational parameters like voltage and time to optimize the treatment for specific types of solutions.
- Lower Risk of Fouling: With intervals between batches, there’s less likelihood for membranes to foul during operation, which can be easier to manage and maintain.
However, batch processes may present limitations related to throughput. Users should be aware that this mode can be time-consuming, especially for larger treatment needs. The requirement for frequent switching between batches can also lead to higher energy consumption per unit volume processed.
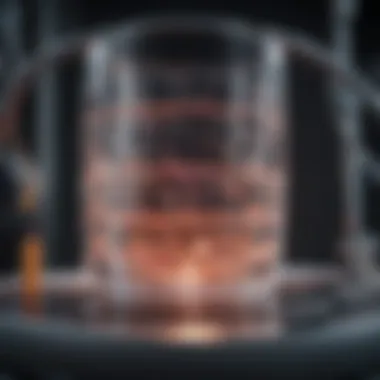
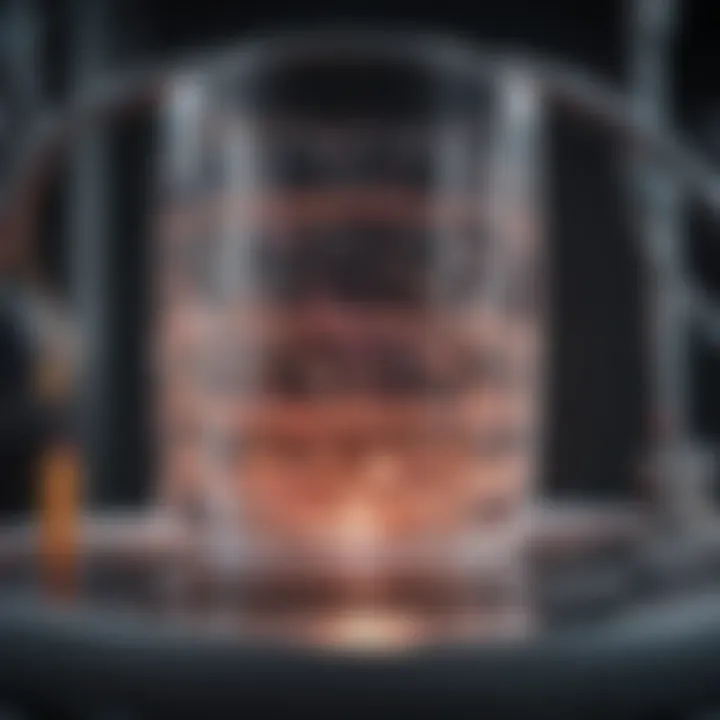
In summary, the choice between continuous flow and batch mode electrodialysis largely depends on the specific application’s demands and the nature of the feed solution.
Both modes offer distinct advantages and challenges, and understanding these operational nuances enables better integration of electrodialysis technologies in various fields.
Applications of Electrodialysis
Electrodialysis stands as a beacon of innovation in the landscape of separation technologies. Its applications in water desalination, wastewater treatment, and chemical industry processes demonstrate its pivotal role in addressing pressing environmental and industrial needs. By utilizing ion-selective membranes and electric fields, electrodialysis effectively separates ions from solutions in a more energy-efficient manner compared to traditional methods, such as reverse osmosis. As we explore these applications, several key facets will come to light, including sustainability, cost-effectiveness, and versatility.
Water Desalination
Water scarcity is a pressing issue that affects millions globally. Electrodialysis offers a viable solution for converting saline water into fresh, drinkable water. By selectively removing ions from seawater or brackish sources, electrodialysis significantly reduces the energy consumption associated with desalination processes. Traditional methods often rely on high pressure, which can be energy-intensive and costly.
In contrast, electrodialysis operates through an ionic movement facilitated by an electric field, thus lowering the energy footprint and operational costs. It proves particularly advantageous in regions where energy availability is limited, such as remote or developing areas. Using electrodialysis for desalination not only addresses water availability but also reduces the environmental impact typical of conventional desalination methods.
Wastewater Treatment
Wastewater treatment is another domain where electrodialysis shines. This method efficiently removes contaminants from industrial wastewater, making it a frontrunner in recovery processes. The technology enables the selective extraction of valuable ions, such as sodium and chloride, which can then be reused in different processes.
Moreover, electrodialysis helps in resource recovery, creating the opportunity to turn a liability into an asset. As industries face increasing regulations regarding wastewater discharge, adopting electrodialysis can lead to both compliance and cost-saving benefits. This leads to a more sustainable approach, enabling industries to reclaim water and minimize waste. The process not only cleans but also reintegrates into the water cycle, contributing positively to environmental stewardship.
Chemical Industry Processes
Within the chemical industry, electrodialysis performs critical roles in the recovery of acids and hydroxides, supporting the sustainable practices necessary for modern manufacturing.
Acid Recovery
The acid recovery process illustrates the advantages of electrodialysis vividly. By enabling the extraction of acids from waste streams, electrodialysis not only helps in reducing hazardous waste but also recycles valuable resources. This not only curtails waste disposal costs but also conserves natural resources. The key characteristic of acid recovery is its efficiency in operation; it handles acidic waste with less chemical intervention compared to traditional methods. The unique feature here is that it can achieve high recovery rates while maintaining the integrity of the chemicals involved, thus positioning itself as a popular choice for industries looking to improve sustainability and reduce operational costs.
Hydroxide Recovery
On the other end of the spectrum, hydroxide recovery also displays the potential benefits when leveraging electrodialysis. This process allows for the efficient recovery of hydroxides from dilute solutions, particularly in industries that produce large volumes of ethylene glycol or similar compounds. A primary advantage is the ability to reclaim hydroxides without the need for significant energy input or complex process setups. The key characteristic here involves the system's flexibility in adapting to different waste stream concentrations. This adaptability often makes hydroxide recovery economically attractive, presenting manufacturers with a dual opportunity to minimize waste and capitalize on valuable byproducts.
In summary, the applications of electrodialysis, particularly in water desalination, wastewater treatment, and chemical processes, highlight its essential nature and growing importance in a world confronting scarcity and environmental concerns. By fostering sustainability and promoting resource recovery, electrodialysis positions itself as a cornerstone of future technological advancements in separation and purification techniques.
Advantages and Limitations
Understanding the advantages and limitations of electrodialysis is essential for evaluating its effectiveness as an electrochemical separation technique. This exploration lays the groundwork for informed decision-making in various industries and applications. In this section, we will delve into the benefits that make electrodialysis a valuable method, as well as the challenges that can impact its efficiency and implementation.
Benefits of Electrodialysis
Electrodialysis presents several distinct benefits that make it a noteworthy choice for separation processes. Firstly, the efficiency of electrodialysis in removing ions from solutions stands out. Its operation can effectively separate salts from water, making it highly suitable for desalination purposes. Additionally, it is energy-efficient compared to other desalination methods like thermal distillation.
Another advantage is its modular design; systems can be scaled up or down based on specific needs. This flexibility fits well in various applications, from small-scale operations to large industrial processes. Also noteworthy is the minimal production of brine, which is a concern in other desalination methods.
Electrodialysis also supports the recovery of valuable ions from wastewater, which can be reused in various industries, promoting sustainability. The environmental impact is comparatively lower since it employs less harsh chemicals than traditional methods.
Challenges Facing Electrodialysis Technology
Despite its advantages, electrodialysis does have its share of challenges that must be navigated for optimal performance.
Membrane Fouling
Membrane fouling refers to the buildup of materials on the membrane surface, which hinders its ability to effectively separate ions. This can lead to reduced efficiency and increased operational costs due to frequent maintenance or replacement. The key characteristic of membrane fouling is its unpredictability. It can arise from various sources, including organic compounds, suspended solids, and smectic layers that accummulate during operation.
In the context of this article, discussing membrane fouling is beneficial because it underscores an important aspect of electrodialysis technology: maintaining membrane integrity is essential for achieving desired outcomes. While membrane fouling can be mitigated through regular cleaning and the use of anti-fouling membrane materials, it remains a significant impediment.
Energy Consumption
Energy consumption is another important aspect to consider when evaluating electrodialysis systems. While generally recognized for its energy efficiency, the actual consumption of electricity can vary substantially depending on the specific configuration and quality of the membranes being used. The key characteristic of energy consumption in electrodialysis is that it is often lower than traditional methods but can still represent a considerable operational cost.
From the perspective of this article, energy consumption is crucial as it ties directly into the overall efficiency and sustainability of the system. With advancements being made in optimizing energy usage through innovative technologies and better materials, the unique feature of energy consumption highlights both the potential for reducing costs and the challenges of keeping energy use at a minimal. Investing in more efficient systems or integrating renewable energy sources could further augment the sustainability of electrodialysis processes.
"Understanding both the benefits and limitations of electrodialysis gives us a clearer picture of its role in modern-day water treatment and chemical processing."
Innovations in Electrodialysis Technology
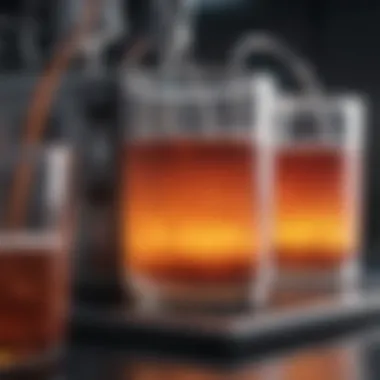
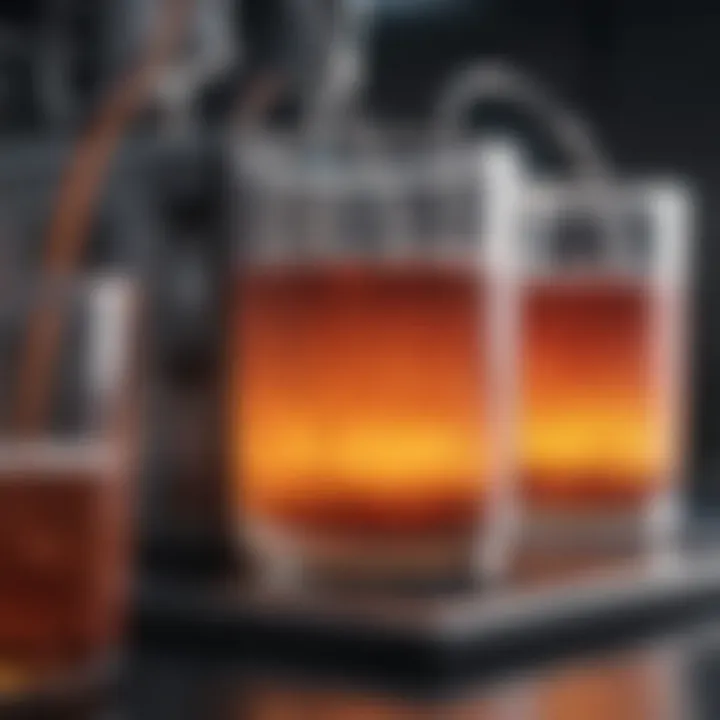
As the demand for sustainable water solutions and efficient waste management continues to grow, innovations in electrodialysis technology are shaping the landscape. These advancements not only improve the performance of existing systems but also open doors for new applications across various sectors. Understanding these innovations is crucial, as they can mean the difference between outdated systems and cutting-edge technology that meets today's needs.
Advancements in Membrane Materials
New materials for membranes are a game-changer in electrodialysis systems. Traditional membranes could be susceptible to fouling, which leads to decreased efficiency over time. Innovations in membranes, including the development of nanostructured and selectively permeable materials, have been pivotal. These advancements help mitigate fouling and increase the lifespan of membranes, leading to lower replacement costs.
Moreover, integrating high-performance polymer structures has shown to enhance ion selectivity. For example, membranes made from graphene oxide display significant potential in improving the efficiency of ion transport. This not only speeds up the desalination process but also can potentially reduce the energy consumption associated with electrodialysis.
Integration with Other Technologies
Integrating electrodialysis with other technologies amplifies its capabilities. This can lead to more resource-efficient and less energy-intensive processes.
Reverse Osmosis
Reverse osmosis (RO) is often reflected upon as an effective method for desalination and water purification. Its key characteristic is the ability to remove a wide range of contaminants from water, making it a popular choice in industries where water quality is paramount. However, the energy consumption associated with RO can be significant, which presents an opportunity for enhanced systems through the integration of electrodialysis.
By combining reverse osmosis with electrodialysis, users can benefit from the removal of specific ions, reducing the overall burden on the RO system. This synergy allows for lower energy use while sustaining high-quality output. While RO excels at filtering out larger contaminants, electrodialysis effectively targets ions. This complementary nature enhances overall process efficiency.
Electrodialysis Reversal
Electrodialysis reversal (EDR) is another notable integration with electrodialysis systems. EDR’s core characteristic, which is the ability to periodically reverse polarity, allows for easier management of fouling and scaling. This feature is particularly advantageous in brackish water treatment where fouling is a massive concern.
The decision to utilize EDR in certain applications can yield numerous benefits, such as increased operational stability and extended membrane life. However, it's not without its challenges; the complexity it introduces to system operations might involve higher initial costs and the need for skilled personnel to manage and maintain such systems. Despite this, the long-term operational savings tend to outweigh these challenges, making it an attractive option for many.
"The integration of innovative technologies in electrodialysis not only enhances performance but also contributes to long-term sustainability goals across various industries."
In summary, the advancements in membrane materials combined with the strategic integration of electrodialysis with technologies like reverse osmosis and electrodialysis reversal not only improves efficiency but also sets the stage for sustainable practices in water treatment. The ongoing research in these areas holds promise for even greater innovations, benefitting both the environmental and economic aspects of water management.
Whether you are a researcher, an educator, or an industry professional, staying abreast of these developments is essential for harnessing the full potential of electrodialysis in future applications.
Future Directions for Electrodialysis
As we move forward, the potential of electrodialysis emerges not only as a cornerstone technology, but also as a dynamic force geared towards solving pressing global issues. With climate change, water scarcity, and industrial sustainability at the forefront, the future directions for electrodialysis promise not only technological advancements but also significant environmental benefits. This segment sheds light on evolving research trends that could revolutionize the field while exploring new applications that could broaden the scope of electrodialysis beyond traditional uses.
Research Trends
In recent years, research efforts have surged in various facets of electrodialysis. Noteworthy trends include:
- Membrane Development: Innovators are examining new materials and configurations for membranes that can enhance ion selectivity and reduce fouling, thus increasing overall efficiency in separation processes.
- Hybrid Systems: Integrating electrodialysis with other technologies, like bioreactors and solar energy systems, is gaining traction. This hybrid approach can maximize resource recovery and minimize environmental impacts, addressing multiple issues at once.
- Computational Modeling: Advances in computational techniques are being used to develop predictive models. These models help optimize system designs and operational parameters, ultimately leading to better performance across various applications.
- Sustainability Metrics: Measuring the sustainability of electrodialysis processes has become critical. Researchers are delving into life cycle assessments, focusing on energy consumption, environmental footprints, and resource use to ensure that electrodialysis remains a green technology.
With these overarching research trends, we can expect significant strides in efficiency, performance, and sustainability of electrodialysis systems in the coming years.
Potential New Applications
As the landscape of electrodialysis continues to evolve, several potential new applications are surfacing that could extend its reach beyond conventional uses. These applications could pave the way for groundbreaking advancements across numerous industries:
- Mineral Recovery: Besides wastewater treatment and desalination, electrodialysis could play a crucial role in the selective recovery of valuable minerals from mining effluents, reducing the environmental impact of mining activities.
- Agricultural Benefits: There's potential for using electrodialysis in agriculture, particularly in soil conditioning. Reclaiming nutrients and detoxifying saline water can improve soil health and yield.
- Food and Beverage Industry: In the food industry, electrodialysis has possibilities for processes like flavor extraction and salt reduction in products such as cheese or sauces. This could lead to healthier options while maintaining product quality.
- Pharmaceuticals: Lastly, the pharmaceutical sector could see innovations in drug formulation and purification processes through the integration of electrodialysis, allowing for more efficient separation methods in drug manufacturing.
As we explore these new possibilities, electrodialysis is positioned not just as a utility, but as a versatile solution championing sustainable practices in various fields.
In summary, the future of electrodialysis is a tapestry of potential. Ongoing research is not only focused on optimization but also on unlocking new avenues that can make a significant impact. This forward-thinking approach confirms that electrodialysis technology can adapt and thrive amidst evolving demands and challenges.
Finales
In the realm of electrochemical processes, the significance of electrodialysis cannot be overstated. This technique serves as a bedrock for addressing some of the pressing challenges in water purification, chemical separation, and many other industrial applications. Understanding the fundamentals laid out in this article offers invaluable insights into how electrodialysis operates and its broader implications.
One of the key benefits of electrodialysis is its ability to efficiently separate ions, which greatly enhances desalination efforts and wastewater treatment. The technology holds promise for industrial sectors aiming to optimize resource recovery while minimizing environmental impact. Furthermore, as highlighted through each section, advancements in materials and integration with complementary technologies can amplify electrodialysis's overall efficacy.
"Electrodialysis stands at the intersection of innovation and necessity. Its evolving capabilities could make it pivotal in sustainable resource management."
Recognizing the importance of ongoing research into this technology cannot be sidelined. With various challenges such as membrane fouling and energy use being persistent issues, continuous exploration is fundamental to developing more robust systems. Moreover, understanding the new applications of electrodialysis allows researchers and practitioners to transcend traditional boundaries, potentially unlocking solutions to emerging global challenges.
Recap of Key Points
- Electrodialysis is an essential electrochemical technique used extensively for desalination, wastewater treatment, and chemical processing.
- Basic principles include ion transport mechanisms and the influence of an electric field, which are crucial for operational efficacy.
- Components of the system such as different types of membranes (anion, cation, and composite) are fundamental to achieving desired outcomes.
- Applications span across numerous industries, emphasizing the versatility of electrodialysis in diverse contexts.
- Innovations in membrane technologies and integration with systems like reverse osmosis point to an exciting future for electrodialysis.
- Research and development efforts are critical to overcoming existing challenges and enhancing the potential of this technology.
Importance of Ongoing Research
Ongoing research in electrodialysis is vital for several reasons:
- Enhancing Efficiency: Continuous study leads to the identification of more efficient membrane materials and operational modes.
- Addressing Challenges: As the technology matures, understanding and mitigating challenges such as energy consumption and fouling is essential.
- Expanding Applications: Research unveils new applications, such as in resource recovery from industrial waste streams, which could transform sectors heavily reliant on water.
- Sustainability Goals: In an era increasingly focused on sustainability, advancements in electrodialysis can contribute to meeting global environmental targets by providing innovative solutions to water scarcity and pollution.
Keeping abreast of these developments will enable a more comprehensive grasp of electrodialysis and its pivotal role in the future landscape of electrochemical technologies.