Neuronal Regeneration: Mechanisms and Applications

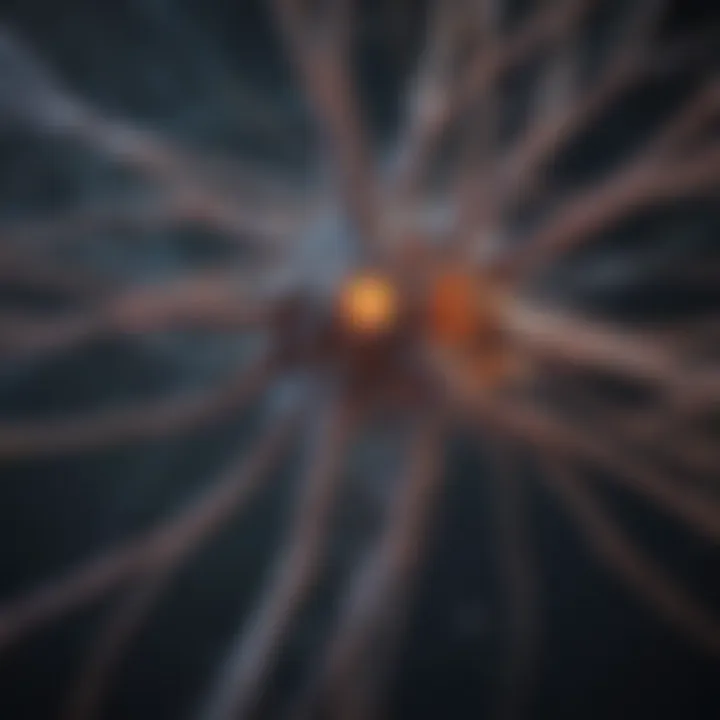
Intro
Neuronal regeneration has become a focal point in the fields of neurobiology and medicine. The ability of neurons to repair and grow after being damaged can make a significant difference in the lives of individuals who suffer from neurodegenerative diseases or injuries. This intricate process hinges on various cellular mechanisms that are not only fascinating but hold a promise for therapeutic advancements as well. By deciphering the biological pathways that facilitate neuronal repair, researchers gain insights that could lead to groundbreaking treatment options, reshaping our understanding of brain injuries and their recovery.
In today's discussion, we dive deep into the fundamental aspects of neuronal regeneration, exploring its key components and mechanisms, alongside the implications it has on rehabilitation and medicine. Through this exploration, we aim to illuminate why understanding neuronal regeneration is crucial for students, researchers, educators, and professionals who are vested in the nuances of neurobiology.
Key Findings
Major Results
Recent studies in neuronal regeneration highlight several significant findings. Some of the most compelling include:
- Neuroplasticity: One essential finding is the role of neuroplasticity in recovery. When a neuron is damaged, the surrounding environment can influence its ability to regenerate. Neural circuits can adapt over time, creating new pathways that bypass damaged ones.
- Stem Cells: Research indicates that stem cell therapy may enhance the regenerative capacity of neurons. These cells can differentiate into various types, offering potential for repairing injured neuronal networks.
- Molecular Signals: Factors such as neurotrophic factors, particularly brain-derived neurotrophic factor (BDNF), have shown to promote neuronal survival, differentiation, and synaptic plasticity. Understanding these molecular signals can open the door for therapeutic applications.
Discussion of Findings
The implications of these findings are vast. The interplay between neuroplasticity and molecular signaling suggests that creating an optimized environment for neuronal growth can substantially influence recovery outcomes. By leveraging stem cell therapy, clinicians can aim to enhance the natural repair mechanisms of neurons. However, the effectiveness of such approaches still requires extensive investigation. Additional studies are needed to clarify the intricate balance between promoting regeneration and preventing the formation of non-functional or scarring tissue.
"The journey of understanding neuronal regeneration highlights the complexity of the human nervous system, reflecting its remarkable capacity for resilience as well as the challenges we confront in therapeutic contexts."
Methodology
Research Design
The research into neuronal regeneration employs a variety of methodologies, combining both experimental studies and clinical trials. The design is often centered around longitudinal studies that monitor patients over extended periods, assessing their recovery after interventions targeting neuronal growth and repair.
Data Collection Methods
Data collection in this domain typically involves:
- Clinical assessments: Neurological evaluations to measure functional recovery in patients undergoing treatment.
- Imaging Techniques: Techniques such as MRI and PET scans can visualize brain activity and structural changes over time due to regeneration.
- Molecular Analysis: Gathering samples to analyze the expression of growth factors and other relevant biomarkers.
These methods collectively provide a rounded view of neuronal regeneration processes, offering crucial insights into how interventions may optimize recovery after nerve injuries or neurodegeneration.
Prelude to Neuronal Regeneration
Neuronal regeneration is not just a fascinating biological process; it’s a vital area of study that holds significant implications for neuroscience, medicine, and even technology. Understanding how neurons can repair and grow after an injury is crucial for developing treatments for various neurological disorders and injuries, including spinal cord injuries and degenerative diseases like Alzheimer's. This section explores the importance of neuronal regeneration, focusing on its specific elements and benefits for various audiences.
The study of neuronal regeneration informs us about potential therapies that could vastly improve patient outcomes. Research in this area offers hope for restoring lost functionalities and enhancing quality of life for individuals suffering from debilitating conditions. For students and educators, this topic presents an opportunity to engage with a dynamic field that is constantly evolving. Moreover, for practitioners, it emphasizes the significance of staying updated on the therapeutic advances that could shape clinical practices in the future.
Defining Neuronal Regeneration
Neuronal regeneration refers to the process by which neurons repair themselves and regrow following damage. This may include the regeneration of axons, the long projections that transmit signals between neurons, as well as the restoration of connections between neurons, known as synapses. Unlike most cells in the body, the ability of neurons to regenerate is limited and varies greatly across species.
In higher mammals, such as humans, neuronal regeneration occurs primarily in the peripheral nervous system, not the central nervous system. In contrast, some lower vertebrates, like zebrafish, possess remarkable regenerative capabilities, which serve as a model for understanding the underlying mechanisms. The interplay between intrinsic repair mechanisms and external influences is key in the regeneration process, determining the fate of the injured neuron and its capacity for recovery.
Historical Context and Evolution of Research
The historical context surrounding neuronal regeneration is both rich and complex. Early research in this field can be traced back to the late 19th century when scientists like Santiago Ramón y Cajal began elucidating the structure of neurons. His groundbreaking work laid the foundation for modern neuroscience by advocating that neurons are discrete entities that communicate through specialized connections.
In the decades that followed, the prevailing view was pessimistic; it was commonly believed that once neurons were damaged, they could not regenerate. However, the latter half of the 20th century witnessed significant breakthroughs, challenging this dogma. Notably, studies on mammalian spinal cord injuries indicated some degree of plasticity and adaptability.
In recent years, advancements in molecular biology, genetics, and imaging technologies have propelled our understanding further. Researchers now explore how cells respond to injury, identify signaling pathways involved in regeneration, and investigate potential therapeutic interventions to enhance these processes. As collaborative efforts between neuroscience, biotechnology, and robotics intensify, fresh avenues for research are continually emerging, opening the door to potential treatments that were previously thought impossible.
"The journey of understanding neuronal regeneration illustrates how far we have come, but it also highlights the significant challenges that remain in harnessing this knowledge for therapeutic use." - Anonymous
Through this historical lens, it becomes clear that while the field has deep roots, we are only scratching the surface of what might be achieved through neuronal regeneration research.
Understanding Neurons and Nervous System
The exploration of the neuronal regeneration process necessitates a comprehensive understanding of neurons and the complex nervous system in which they operate. This framework aids in appreciating the intricate mechanisms behind neuronal growth and repair. Recognizing how neurons function, their various types, and the pathways they form is crucial for anyone keen on embracing the depths of neuronal regeneration.
Anatomy of Neurons
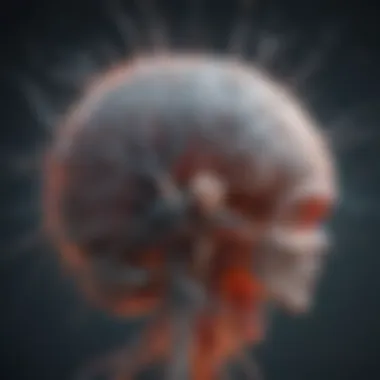
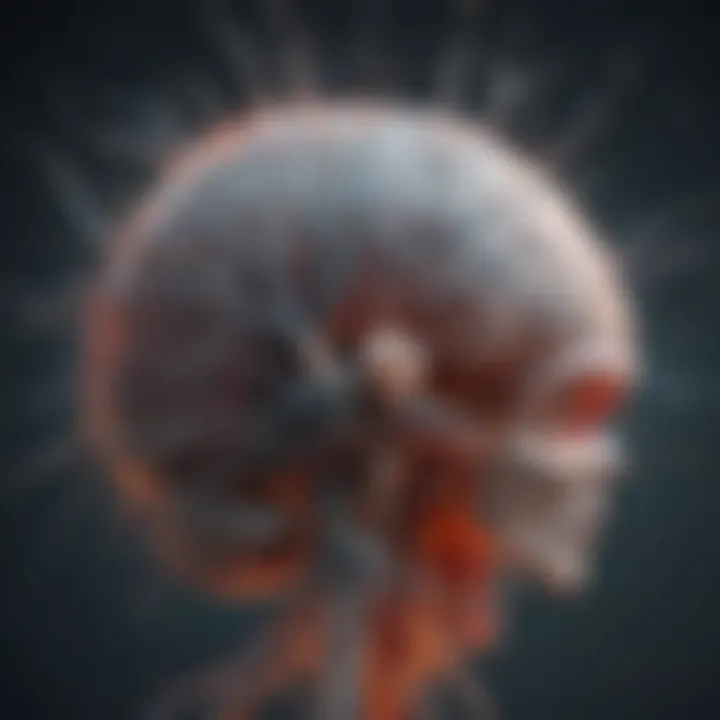
Neurons, often referred to as the fundamental units of the brain and nervous system, exhibit a distinct structure suited for their roles in communication. A typical neuron comprises several parts:
- Cell Body (Soma): This is the neuron's core, housing the nucleus and vital organelles responsible for sustaining cellular functions.
- Dendrites: These tree-like extensions receive signals from other neurons. The greater the dendrite network, the more information a neuron can gather.
- Axon: This is a long, slender projection that transmits electrical impulses away from the cell body towards other neurons or muscles. Some axons are sheathed in a fatty layer called myelin, which enhances signal transmission speed.
- Synapse: The gap between two neurons where communication occurs through the release of neurotransmitters.
Understanding this anatomy is key, as injuries or degeneration affecting any part can impede neuronal regeneration, directly impacting a person’s neurological functions.
Types of Neurons
Neurons can be classified into three primary types based on their functionality:
- Sensory Neurons: These neurons convey information from sensory receptors to the central nervous system (CNS). They play a vital role in processing stimuli from the environment, such as light, sound, touch, and pain.
- Motor Neurons: These neurons transmit signals from the CNS to muscles or glands, initiating movement or secretion. Understanding motor neuron function is pivotal, especially in rehabilitation contexts where motor control recovery is essential.
- Interneurons: Acting as connectors, they facilitate communication between sensory and motor neurons within the CNS. Interneurons are fundamental in reflexes and regulating the flow of information in the nervous system.
By grasping the differences between these neuron types, one can better appreciate how damage to specific types impacts overall health and recovery processes in neuronal regeneration.
Neural Pathways and Functional Networks
Neurons do not operate in isolation; they form extensive networks that relay signals throughout the nervous system.
- Neural Pathways: These defined routes allow for efficient signal transmission, as neurons transmit information along established connections. For instance, pathways involved in pain transmission enable immediate reflex responses, which are crucial for survival.
- Functional Networks: Different groups of neurons collaborate for intricate processes like movement, thought, and sensation. Understanding these networks sheds light on how neuronal regeneration affects various functions.
This understanding creates a backdrop for the potential interventions discussed later in this article, emphasizing the importance of optimizing the conditions under which neurons can regenerate effectively.
"To understand the body’s healing processes, one must first comprehend how its parts interact intricately; this is especially true for the nervous system."
By illustrating the anatomy of neurons, classifying their types, and mapping out functional networks, this section lays the groundwork necessary to delve into the mechanisms of neuronal regeneration. Understanding these components is not merely academic; it offers insights that can aid in the development of more effective therapeutic methods aimed at repairing and regenerating neuronal tissues.
Mechanisms of Neuronal Regeneration
Understanding the mechanisms of neuronal regeneration is crucial for several reasons. By comprehending how neurons repair and regrow, researchers can develop effective treatments for various neurological conditions. This section dives into key elements of these mechanisms, emphasizing their benefits and considerations. The intricate dance between intrinsic and extrinsic factors ultimately dictates the success of regeneration in neurons, shaping both clinical and research landscapes.
Intrinsic Repair Mechanisms
Intrinsic repair mechanisms are the biological processes within the neurons themselves that enable them to heal and regenerate. These mechanisms can be likened to a toolbox that neurons use for self-repair, highlighting their inherent resilience. Here are a few standout components:
- Axonal Regeneration: After injury, axons have the capability to regrow. This process can be seen in sensory neurons, which often regenerate more effectively than central nervous system neurons. Factors like age and injury severity significantly affect this capability.
- Neurite Outgrowth: Crucial to regeneration, neurite outgrowth involves the sprouting of new branches from the neuron. Growth cones—dynamic tips of the neurites—play a pivotal role as they navigate the environment to find targets.
- Gene Expression: Healing requires changes at the genetic level. After an injury, specific genes responsible for repair mechanisms are upregulated. For example, the upregulation of growth-associated protein 43 (GAP-43) is vital for neuronal regeneration, acting as a signal for the repair processes.
The regenerative capacity of neurons, however, varies enormously based on factors such as location and type of neurons. While some neurons manage to regenerate spontaneously, others struggle or fail entirely, underscoring the mixed successes of intrinsic mechanisms.
Extrinsic Factors Influencing Regeneration
Extrinsic factors significantly influence the capacity of neurons to regenerate. These elements, arising from the surrounding environment, can either facilitate or hinder the regeneration process. Their role cannot be overstated:
- Cellular Environment: The presence of supportive cells, such as Schwann cells in the peripheral nervous system, enhances regeneration. These cells provide structural support and secrete growth factors. Conversely, the glial scar formed after central nervous system injuries can obstruct regeneration, creating a hostile environment.
- Chemical Signals: Neurotrophic factors, such as brain-derived neurotrophic factor (BDNF), are essential. They not only promote survival but also enhance growth. The balance of these chemical signals can tilt regeneration in favor or against recovery.
- Physical Factors: The structure of the injury site can influence outcomes. For instance, aligning the extracellular matrix can guide regenerating axons, improving chances for successful reconnection.
Extrinsic influences can create a double-edged sword; while they foster healing in some contexts, they can also impose barriers that neurons must overcome.
Cell Signaling Pathways
Understanding cell signaling pathways is pivotal to decode how neurons communicate and react during the regeneration process. These pathways relay important information within and outside the cell, driving various regeneration steps:
- MAPK/ERK Pathway: This pathway is well known for its role in cell proliferation and differentiation. In the context of neuronal regeneration, it is critical. Activation of this pathway promotes neurite outgrowth, and various neurotrophic factors operate through this route.
- PI3K/Akt Pathway: This pathway is fundamental in promoting cell survival and can prevent apoptotic cell death. Inhibition of apoptosis post-injury is vital for allowing neurons time and resources to enact repair and regeneration processes.
- Wnt Signaling: Recently, Wnt signaling has garnered attention for its role in both development and regeneration. This pathway appears to be intricately involved in guiding axonal regrowth.
These signaling cascades offer potential intervention points for therapeutic strategies. By modulating these pathways, it may be possible to boost the chances of successful neuron regeneration in various clinical scenarios.
"The interplay between intrinsic and extrinsic factors is a dance of resilience and opportunity that defines the prospects of neuronal regeneration."
In summary, mechanisms of neuronal regeneration involve a complex interplay of intrinsic repair capabilities, extrinsic environmental factors, and sophisticated cell signaling pathways. Understanding each component's nuances leads not only to better treatments but also to a deeper appreciation of neural resilience.
Neuronal Plasticity and Its Role in Regeneration
Neuronal plasticity is like the secret sauce that adds flavor to the process of neuronal regeneration. It's not nearly enough to just have the ability to repair — how those neurons connect, adapt, and learn after injury can make all the difference in recovery. This section digs into the importance of neuronal plasticity, focusing on its various elements and the multiple benefits it posits for regeneration after neural damage.
Plasticity can be thought of as a two-sided coin, showcasing both synaptic and structural aspects. The former primarily pertains to how pathways and connections between neurons can strengthen or weaken over time, shaped by experience, environment, and, crucially, injury. The latter refers to changes in the physical structure of neurons, which can occur when existing connections are lost, and new ones are formed, optimizing the nervous system’s response to damage.
Two key points are essential in recognizing its relevance:
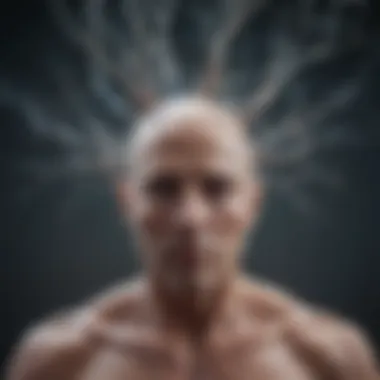
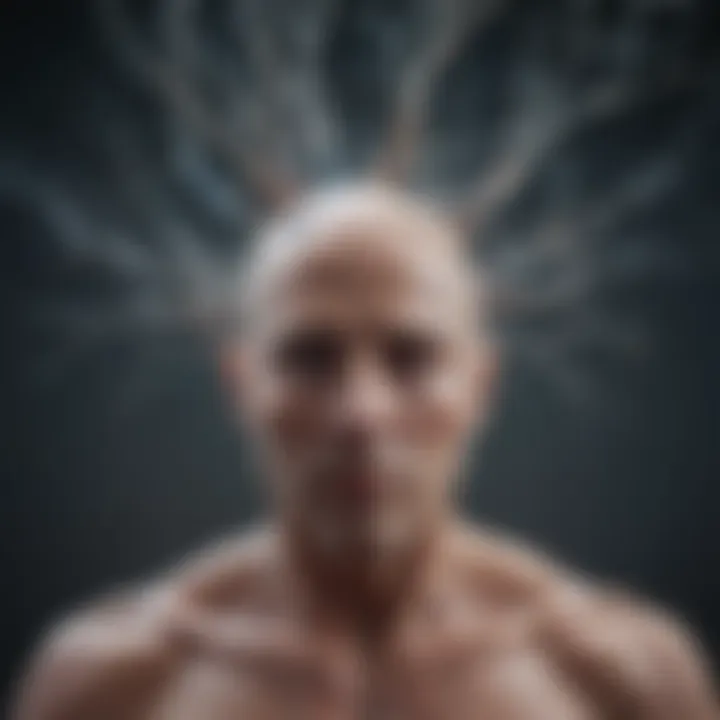
- Adaptability: The brain's ability to rewire itself, allowing for alternate pathways when traditional routes are obstructed.
- Learning: Plasticity isn't just about repair; it also extends to the ability to learn from the injury itself and adjust accordingly.
The interconnection between neuronal plasticity and regeneration extends beyond merely understanding the biological mechanisms; it poses significant implications for how we approach rehabilitation therapies and treatments after neurological damage.
"The brain doesn't just heal; it reinvents itself."
Synaptic Plasticity
Synaptic plasticity refers to the processes that enable synapses, or the junctions between neurons, to change their strength based on activity. Consider it a form of communication that can become more profound or fragile depending on the experiences or injuries. After a neuronal injury, if the synapses can adapt and modify their effectiveness, the chances of functional recovery increase.
Some features worth emphasizing include:
- Long-term potentiation (LTP): The phenomenon where repeated stimulation of synapses leads to more robust synaptic strength. This is crucial in learning and memory and also plays a role in how the brain adapts post-injury.
- Long-term depression (LTD): A process where synaptic strength decreases, essential for distinguishing between beneficial and harmful signals — it aids in the adjustment of neuronal networks.
In rehabilitation, enhancing synaptic plasticity might entail targeted therapies or exercises designed to repeatedly activate certain pathways, fostering stronger connections while retraining the brain.
Structural Plasticity
Structural plasticity goes beyond transient changes in synaptic strength. It involves long-lasting modifications in the structure of neurons. After an injury, neurons might sprout new dendrites and axons to reconnect with other neurons, something that can be visualized as a road being rebuilt after a flood.
Points of interest with structural plasticity include:
- Neurogenesis: The possibility of generating new neurons from neural stem cells, contributing to the recovery process across several brain regions.
- Dendritic remodeling: Changes can occur in the shape and size of dendritic trees which can enhance or inhibit connectivity with other neurons. Renovating the dendritic landscape gives neurons the ability to form new relationships post-injury.
Understanding structural plasticity means not just treating the symptoms of neuronal damage, but also fostering an environment conducive to growth and rebuilding. It underlines the necessity of integrating strategies that support both synaptic and structural plasticity in therapeutic practices moving forward.
Through an intricate dance of building new pathways and adjusting connections, neuronal plasticity shapes the journey of regeneration, influencing not only physiological recovery but also cognitive and functional outcomes.
In essence, by effectively engaging with these two forms of plasticity, we can leverage an individual’s innate capacity to heal and adapt, leading to greater horizons in the landscape of neuronal regeneration.
Challenges in Neuronal Regeneration
Understanding the challenges in neuronal regeneration is crucial for anyone diving into the fields of neurobiology and rehabilitation. This mosaic of difficulties not only reflects the biological complexities inherent to the nervous system but also shines a light on potential roadblocks in clinical applications aimed at enhancing recovery after neuronal injuries. Analysis of these challenges reveals not just concerns but also hints at avenues for future research and improvement.
Scar Formation and Its Impediment
A significant hurdle in neuronal regeneration arises from scar formation. When neurons are damaged, the body initiates a healing response, leading to the creation of a scar tissue. This tissue, often filled with reactive glial cells, serves a protective function but inadvertently hinders neuronal reconnections. The scar is like a barricade, making it difficult for neurons to extend their axons and form new synapses.
In simpler terms, if you've ever built a fence around your garden to keep out pests, you can imagine how it feels for injured neurons. The fence does its job of protection but also limits the freedom and growth of the garden within. Research suggests that targeting processes that promote scar tissue formation may bolster regeneration. Inhibiting factors like chondroitin sulfate proteoglycans could make way for better neuronal recovery.
Aging and Degenerative Diseases
Aging represents another layer of complexity. Neuronal regeneration becomes increasingly challenging as we age. The presence of neurodegenerative diseases, such as Alzheimer's or Parkinson’s, exacerbates these challenges. Aging neurons exhibit reduced ability to regenerate, partly due to diminished intrinsic repair mechanisms. It is as if the neurons slow down as if they're not in a race anymore, retreating into a more static phase of life. This diminishment is compounded further by the progressive nature of degenerative conditions, which can generate a relentless cycle of damage.
Moreover, the brain’s microenvironment undergoes changes as age creeps in. The supporting cast of cells—the glia—begin to function less effectively. To tackle these issues, researchers are exploring potential interventions ranging from lifestyle changes like exercising to technological advancements such as gene therapies aimed at rejuvenating neuronal function. In seeking solutions, researchers often look to models that simulate earlier stages of life, providing insight into what goes wrong in aging neurons.
Environmental and Genetic Factors
Environmental influences and genetic predispositions play interconnected roles in neuronal regeneration. Factors such as exposure to toxins or prolonged stress create unfavorable conditions for healing. It's not just a matter of bad luck; these external elements seem to throw additional sand in the gears of recovery. For example, studies have shown that individuals living in polluted areas may experience higher incidences of neurodegeneration.
On the flip side, genetic factors can also dictate the efficiency of neuronal repair. Specific genotypes may possess inherent advantages or disadvantages in the face of neural injury. If we were to think of the nervous system as a garden that needs tending, then these genetic factors act as the seeds that either flourish or wither under varying environmental conditions. Understanding these influences can guide therapeutic strategies tailored to individual needs, offering hope for more personalized treatment plans.
"Neuronal regeneration is not merely a biological process; it is influenced by a symphony of genetic and environmental factors. Understanding this interplay is vital for advancements in regenerative medicine."
Both environmental and genetic factors underscore the need for holistic approaches in addressing challenges to neuronal regeneration. As researchers untangle these webs of influence, there's potential for innovations that could drastically improve outcomes for individuals facing neuronal injuries.
Therapeutic Approaches for Enhancing Neuronal Regeneration
Understanding how to effectively repair and regenerate neurons following injury is of vital importance in the field of neurobiology. Therapeutic strategies can offer tangible benefits, not just for those recovering from severe brain or spinal cord injuries, but also for individuals coping with neurodegenerative diseases. Here, we explore the significance of various therapeutic approaches that can enhance neuronal regeneration and their multifaceted implications in clinical settings.
Pharmacological Interventions
Pharmacological interventions play a pivotal role in facilitating neuronal regeneration. These include a range of drugs designed to either promote the intrinsic repair mechanisms of neurons or alter the microenvironment to support regeneration.
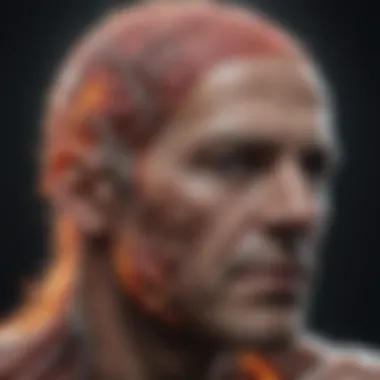
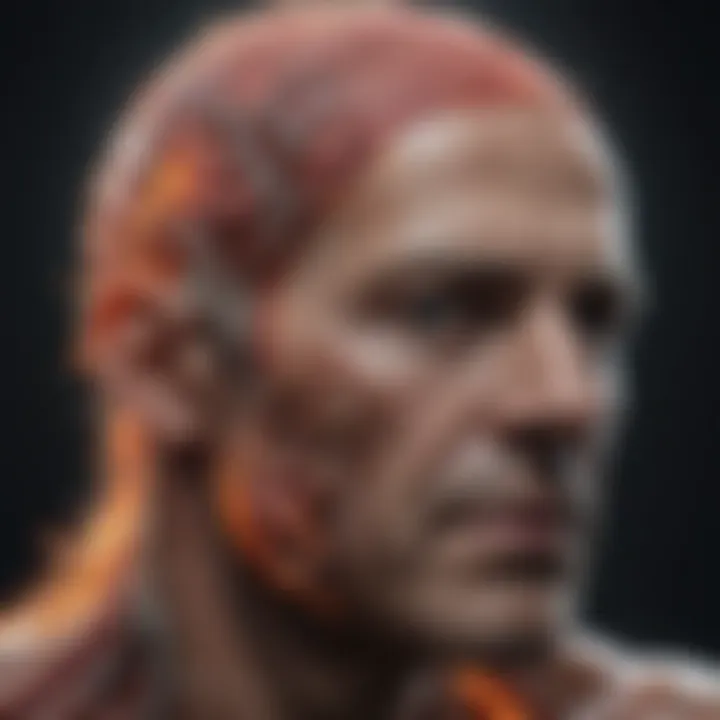
- Neuroprotective Agents: Certain medications have been identified to protect neurons from damage. Drugs like Riluzole, often used in Amyotrophic Lateral Sclerosis (ALS), show potential in limiting neuronal death.
- Growth Factors: Factors such as Brain-Derived Neurotrophic Factor (BDNF) have gained attention for their role in promoting neuron survival and growth. This factor can encourage existing neurons to sprout new connections, helping reestablish lost circuits.
- Anti-inflammatory Agents: Inflammation can significantly impair recovery after nerve injury. By utilizing drugs that address neuroinflammation, researchers aim to create a more conducive environment for regeneration.
These interventions must be approached with care, as the balance between stimulating growth and inducing potential side effects requires thorough investigation.
Stem Cell Therapy
Stem cell therapy represents one of the more promising approaches in enhancing neuronal regeneration. Stem cells offer incredible potential due to their ability to differentiate into various cell types, including neurons, and can be used to replace lost or damaged cells. Here are some key points:
- Types of Stem Cells:
- Clinical Trials: There are ongoing clinical trials exploring the efficacy of stem cell treatments in conditions such as spinal cord injury and stroke. While results are promising, long-term effects and safety need to be more thoroughly examined.
- Challenges: Despite the promise, challenges remain, including tumorigenesis, rejection by the immune system, and ensuring that the stem cells precisely differentiate into the appropriate neuronal types.
- Embryonic stem cells are pluripotent and can become any type of cell, making them a powerful tool for regeneration. However, ethical considerations surround their use.
- Adult stem cells, such as neural stem cells found in the brain, are less controversial and have shown efficacy in preclinical models for promoting neuronal recovery.
Neuroprosthetics and Rehabilitation Technologies
Combining technology with biology, neuroprosthetics and rehabilitation technologies aim to enhance capabilities for individuals with neurological impairments. These tools not only support functional recovery but also actively contribute to the regeneration process.
- Neuroprosthetics: Devices that interface with the nervous system can assist in restoring functions lost due to injury. For example, brain-computer interfaces (BCIs) enable individuals to control devices purely using neural signals, showcasing neuroplasticity in action.
- Rehabilitation Robotics: These are designed to provide guided rehabilitation exercises post-injury. By mechanically facilitating movement, they encourage neural pathways to adapt and strengthen. They can also offer valuable feedback that aids in refining motor skills.
- Virtual Reality and Biofeedback: Emerging technologies using virtual reality applications and biofeedback systems enhance engagement in rehabilitation, facilitating better recovery outcomes by providing an immersive environment for therapy.
The integration of neuroprosthetics reflects a fusion of biology and technology, showcasing human ingenuity in tackling impairments that arise from neuronal damage.
Through these therapeutic approaches, the landscape of neuronal regeneration is increasingly becoming more hopeful. As we dig deeper into these areas, researchers are striving toward ending the stagnation in recovery processes that often follow neurological injuries. By continuously pushing the boundaries, future innovations may hold the key to reclaiming lost functionalities, therefore enhancing the quality of life for many.
Future Directions in Neuronal Regeneration Research
Neuronal regeneration is a field still rife with uncharted territories. Understanding its future directions stemming from recent advancements in research can lead to groundbreaking treatments. Discovering more about this realm can change the landscape of neurobiology and rehabilitation practices. The research in this area holds feasible benefits for developing therapies that repair neuronal damage, enhancing quality of life for individuals suffering from neurodegenerative diseases.
The importance of focusing on future directions in neuronal regeneration research extends to its impact on both basic science and clinical applications. New insights into the cellular and molecular mechanisms responsible for neuronal repair can pave the way towards
- Novel Therapies: Developing targeted treatments can address specific types of injuries or degenerative conditions, potentially revolutionizing patient care.
- Understanding Limitations: By identifying factors that limit regeneration, researchers can tailor interventions more effectively.
- Interdisciplinary Collaborations: Collaboration across various scientific domains can yield a comprehensive understanding of regeneration processes.
Emerging Technologies and Innovations
When we open the door to emerging technologies, we see a whole new toolbox for neuronal regeneration. One of the most notable innovations is the use of biomaterials. For instance, hydrogels can be designed to provide scaffolding that not only supports neuronal growth but also delivers bioactive molecules that can encourage healing. This dual-action approach shows promise in promoting better outcomes after neuronal injury.
Moreover, consider bioelectronics. Recent advances in neurotechnology allow us to interface devices with neural tissues, facilitating communication that can enhance recovery. Devices such as neuroprosthetics can be integrated to enable motor functions lost due to injury. Hence, reconsider how we can utilize these tools in rehabilitation protocols.
Additionally, gene editing tools like CRISPR-Cas9 stand to play a significant role. By selectively correcting mutations at a genetic level within neurons, scientists can potentially reinstate normal function. It's both a daring and exciting pathway that opens doors to entirely new preventive and corrective measures in neurodegenerative disorders.
Interdisciplinary Approaches
Interdisciplinary collaboration is crucial for bolstering research in neuronal regeneration. At its core, this entails bringing together diverse fields such as biology, engineering, psychology, and even social work to enrich the study of neuron repair and recovery. For example, engineers working alongside neuroscientists can develop effective neural interfaces that enhance prosthetic control.
Moreover, integrating knowledge from disciplines like developmental biology might provide insights into how natural regenerative processes occur in other organisms. Studies on organisms such as the axolotl, which can regenerate limbs, could inform strategies for human neuronal regeneration.
Collaboration isn't just beneficial but necessary, as it creates a multidimensional framework to approach complexities in neuronal regeneration. It serves as a bridge, linking research initiatives with practical applications that may lead to effective treatments and improved patient outcomes. As we steer towards the future, the ultimate goal remains the same: enhancing the functionality and quality of life for those affected by neurological impairments.
"The future of neuronal regeneration will likely rely on the convergence of various scientific disciplines, ensuring a holistic understanding of the regeneration process that could inform more effective therapies."
Culmination and Implications for Practice
The area of neuronal regeneration is not just fundamental; it’s practically pivotal in the realm of neurobiology, rehabilitation, and clinical medicine. Understanding how neurons can repair and regrow opens doors to innovative treatments and therapies that could drastically improve the quality of life for individuals affected by neurological injuries or degenerative diseases. Within this article, we've traversed varied landscapes—from the complex mechanisms that underpin neuronal regeneration to the therapeutic interventions that promise hope for many. This concluding segment synthesizes the key takeaways from our exploration and highlights the implications these findings have for both clinical practice and research.
Summary of Key Findings
From the discussions presented in prior sections, several crucial points stand out:
- Neurons possess intrinsic repair mechanisms that enable them to regenerate under specific conditions. This intrinsic capacity can be modulated by various extrinsic factors, including the environment and biochemical cues.
- Neuronal plasticity plays an essential role in recovery, indicating that the nervous system can adapt itself structurally and functionally in response to injury. Understanding plasticity can profoundly impact rehabilitation strategies.
- Therapeutic approaches, such as stem cell therapy and neuroprosthetics, have emerged as promising avenues to bolster the process of regeneration, providing alternate methods for treatment where traditional approaches may falter.
- Challenges persistence—such as scar formation and the effects of aging—that continue to thwart effective regeneration solutions warrant further investigation.
These findings not only underline the intricacies of neuronal repair mechanisms but also signify how varied applications can be considered in practice. Each insight creates a roadmap for future research, laying foundational knowledge that practitioners and researchers can build upon.
Clinical and Research Implications
The implications of these insights extend far beyond theoretical understanding; they hold real-world significance in both clinical settings and research circles.
- In clinical settings, knowledge of neuronal regeneration can lead to improved therapeutic strategies. For instance, clinicians could leverage treatment protocols that enhance plasticity to optimize recovery outcomes in patients with spinal cord injuries or those recovering from strokes.
- Researchers can harness the critical insights presented in this article to innovate and refine approaches. For example, by investigating the pathways that facilitate intrinsic repair, new drug targets can be explored to enhance neuronal regeneration post-injury.
A more robust understanding of these mechanisms not only aids clinical interventions but also shapes research agendas in neurobiology and related fields.
Additionally, interdisciplinary collaborations may arise from the blending of insights from neurobiology, engineering, and pharmacology, reflecting the multi-faceted nature of this research area. Future avenues may also delve deeper into personalized medicine, tailoring treatments based on individual regeneration capacity. This targeted approach provides resilience against inefficacious blanket strategies, maximizing potential positive outcomes for patients.
In summary, the collective insights from neuronal regeneration research present a compelling narrative that underscores the need for ongoing exploration and collaboration in both the clinical and research spheres. Only by deepening our understanding of these processes can we aspire to truly make a difference in the lives of those affected by neurological conditions.