Innovations and Uses of Modern Ceramic Technology
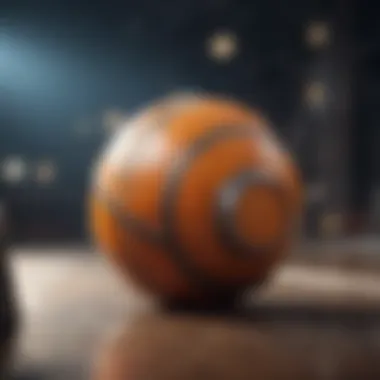
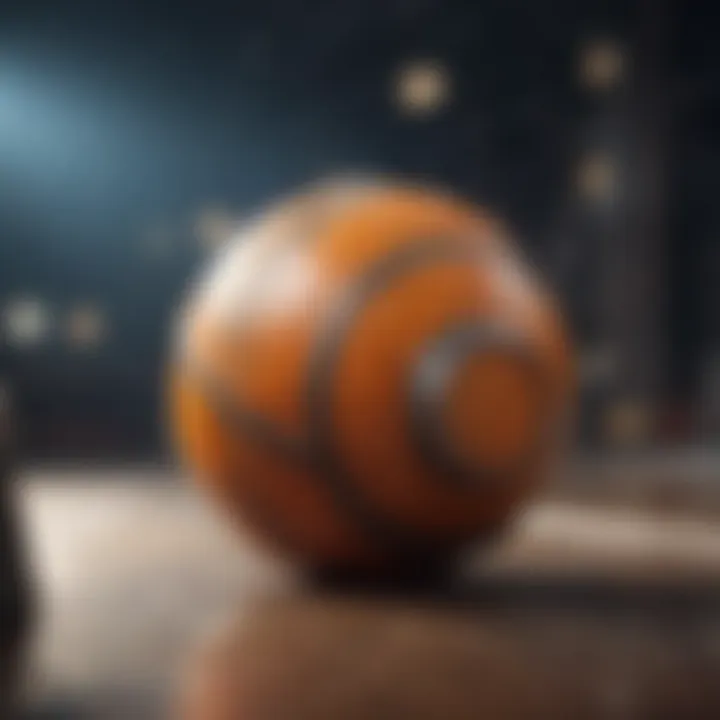
Intro
Ceramics have long been part of human history, but recent decades have seen a remarkable evolution in ceramic technology. Their role spans various fields, encompassing everything from the practical applications in everyday products to cutting-edge uses in high-tech industries. This section delves into how ceramics are transforming sectors like electronics and medicine, marking their significance in the modern age.
For instance, while traditional ceramics were primarily used in pottery, advanced ceramics now find applications in rigid components, biomedical implants, and even electronic devices. This shift to advanced ceramics highlights both innovation and adaptability, painting a picture of a material that is not only durable but also versatile.
Ceramics exhibit unique properties that make them suitable for various uses. Their high-temperature stability, wear resistance, and lightweight characteristics have allowed them to break into territory that was once dominated by metals and plastics. Moreover, with the advent of manufacturing techniques like additive manufacturing (3D printing) and advancements in raw material properties, ceramic technology is reaching new heights.
Several research efforts and technological innovations have provided fertile ground for ceramics to thrive. For instance, the introduction of nano-ceramics has paved the way for significant advancements in strength and thermal resistance. With these developments, the potential applications seem almost limitless, expanding into realms like aerospace technology and renewable energy solutions.
This exploration will further highlight key findings in material science and innovative applications made possible by advancements in ceramics. By delving into detailed methodologies and the incredible advancements in material design and synthesis, we present a comprehensive overview of the vibrant landscape of ceramic technology today.
Preface to Ceramic Technology
Ceramic technology stands at the crossroads of numerous fields, serving each with precision and reliability. From the electric circuits in smartphones to the biocompatible implants that heal bones, ceramics have woven themselves into the fabric of modern society. The significance of ceramic technology can not be overstated; as industries evolve, the demand for advanced materials continues to surge, leading to exciting advancements that push boundaries.
Definition and Scope
Ceramics are often misconceived as delicate materials like china dishes, but their scope extends far beyond everyday items. Essentially, ceramics are inorganic and non-metallic substances, formed through the combination of metallic and non-metallic elements. This results in a diverse range of materials which exhibit remarkable properties, such as heat resistance, durability, and electrical insulating capabilities.
The scope of ceramic technology encompasses both traditional ceramics, such as pottery and tiles, and advanced ceramics, which include materials engineered for specific applications like bioceramics or superconductors. These innovations capture the imagination and serve vital roles in cutting-edge fields including electronics, aerospace, and healthcare.
Historical Context
To truly appreciate ceramic technology, it's crucial to grasp its historical roots. Humans have been crafting ceramics for thousands of years, with archaeological evidence tracing back to 29,000 BC when early peoples fashioned tools and ornaments from this versatile material. From ancient pottery used for storage to the elaborate porcelain developed in China during the Tang dynasty, ceramics underwent continuous evolution shaped by societal needs and available technologies.
The invention of the kiln drastically changed the landscape of ceramic production. This allowed for improved strength and heat resistance, paving the way for innovations in building materials and even art. The 20th century ushered in an era of engineering, leading to modern advanced ceramics that set the stage for the applications we see today.
"Ceramics are a testament to human ingenuity, evolving from basic artifacts to sophisticated materials that enhance various aspects of technology and daily life."
In summation, understanding ceramic technology provides insight into how a seemingly simple material can underlie complex systems at work in today’s world. The blend of historical evolution with contemporary advancements forms a rich tapestry of knowledge, setting the stage for future innovations. Crafting a deeper understanding of this field equips researchers, students, and professionals with the tools to explore and maximize the countless possibilities that lie ahead.
Fundamental Properties of Ceramics
Understanding the fundamental properties of ceramics is crucial for appreciating their roles across various industries. The insights gleaned from these properties allow researchers and engineers to harness ceramics in tailored applications, making them indispensable in fields like electronics, medicine, and aerospace. These properties can be broadly classified into mechanical, thermal, and electrical attributes, each impacting the performance and reliability of ceramic materials in practical scenarios.
Mechanical Properties
Tensile Strength
Tensile strength measures a material's ability to withstand being pulled apart without breaking. For ceramics, this property can be both influential and arguably limiting depending on the context. Ceramics often exhibit impressive compressive strength, but their tensile strength can leave something to be desired, as they typically may fracture under tension.
A key characteristic of tensile strength is its relevance in structural applications. For instance, while using ceramics in load-bearing components, it becomes essential to consider their tensile limitations. The uniqueness of ceramics lies in their ability to maintain structural integrity in high-pressure environments, yet they tend to struggle with tensile stresses.
Pros: Great for high compressive loads.
Cons: Prone to brittle failure under tension.
Hardness
Hardness refers to a material's resistance to deformation or abrasion. Ceramics stand out for their exceptional hardness, making them highly desirable for applications requiring wear resistance, like cutting tools and protective coatings. The hardness of a ceramic can be a result of its crystal structure and bonding, often providing benefits in performance.
This property is notably beneficial in scenarios where longevity and durability are non-negotiable. It allows manufacturers to use ceramics in environments that would quickly erode other materials.
Pros: Long-lasting performance in harsh conditions.
Cons: Can be difficult to work with due to brittleness.
Elastic Modulus
Elastic modulus gauges how much a material deforms under stress. For ceramics, this property often indicates a high resistance to deformation, which can be advantageous in applications where precision is required. The considerable elastic modulus of ceramics allows them to maintain their shape even under significant loads, offering reliability in engineering contexts.
Yet, high rigidity also means limited flexibility. This characteristic must be balanced against the demands of specific applications, like those in neural implants where slight flex is often needed to match different materials.
Pros: Maintains shape under load.
Cons: Limited flexibility may not suit all applications.
Thermal Properties
Thermal Conductivity
Thermal conductivity is the measure of a material's ability to conduct heat. In ceramic technology, this property varies widely among different types of ceramics. Generally, ceramics are known for their low thermal conductivity, which can be a strategic advantage in applications like thermal insulators or heat shields.
This feature becomes particularly beneficial in industries requiring temperature control. The ability to resist heat flow leads to energy savings and improved efficiency, making ceramics an appealing choice for building materials.
Pros: Effective thermal insulators.
Cons: May require additional materials for certain high-load applications.
Thermal Expansion
Thermal expansion describes how materials change in volume with changes in temperature. The controlled thermal expansion of ceramics can be advantageous in high-temperature applications, preventing catastrophic failures that other materials could suffer from thermal stress.
The distinctive quality of ceramics often showcases minimal thermal expansion, which can safeguard precision in electronic components. However, in certain applications, the mismatch in thermal expansion coefficients with adjoining materials can lead to cracking or delamination, which should be diligently considered.
Pros: Stability in thermal environments.
Cons: Risk of failure in multi-material assemblies.
Heat Resistance
Heat resistance is vital for materials exposed to extreme temperatures. Ceramics are frequently used because they can sustain higher temperatures without losing structural integrity. This property stems from their composition, allowing these materials to serve in hostile environments like jet engines or industrial furnaces.
The standout feature here is the ceramic's resilience, making it a popular choice in aerospace and automotive applications where failure is not an option. However, the very characteristics that contribute to their heat resistance can render them brittle, a hurdle that engineers must often navigate.
Pros: High performance in extreme conditions.
Cons: Brittleness can lead to failure under impact.
Electrical Properties
Dielectric Constant
The dielectric constant indicates a material's ability to store electrical energy in an electric field. High dielectric constants are often featured in capacitors, where ceramics play a critical role in enhancing performance. Their ability to exhibit controlled dielectric properties opens doors to various applications in electronics.
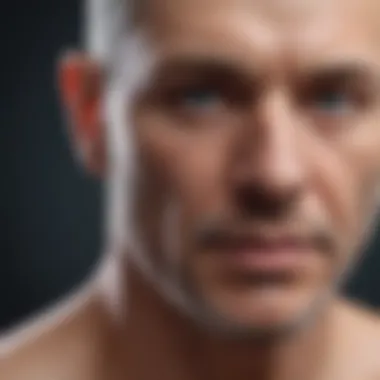
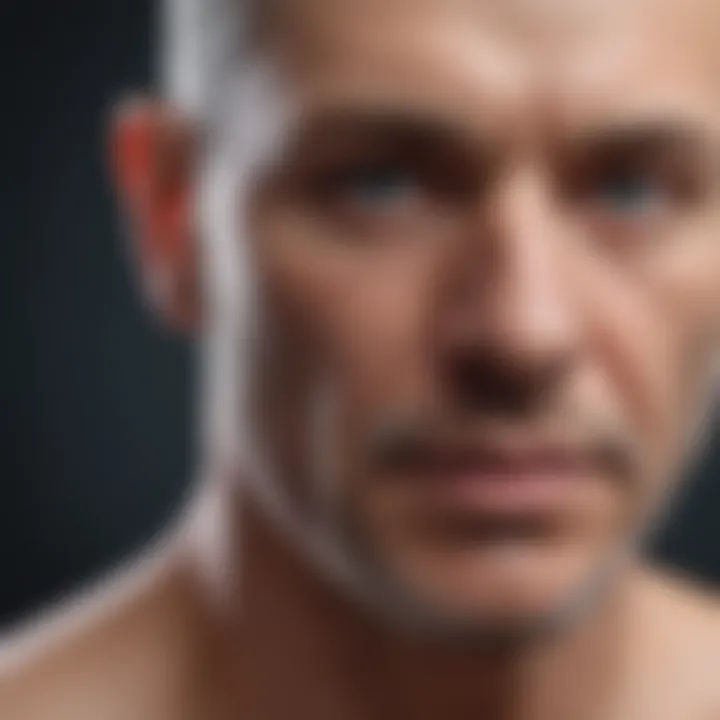
A unique feature of ceramics is that their dielectric constant can be fine-tuned from low to high, depending on the composition and structure. This makes ceramics a versatile option for devices needing efficient energy storage and transmission.
Pros: Versatile for different electronic applications.
Cons: The manufacturing process can influence dielectric properties more than expected.
Insulative Capabilities
Ceramics possess impressive insulative capabilities, making them preferred in electrical applications for preventing unwanted flow of electricity. This feature is critically important for ensuring the reliability and safety of electronic devices, especially in power applications.
The prominent characteristic of ceramics here is their ability to withstand high voltages without breaking down. However, ensuring a uniform dielectric layer is crucial in practical implementations, as inconsistencies could lead to failures or reduced performance.
Pros: Ensures safety in electrical applications.
Cons: Vulnerable to environmental degradation over time.
Piezoelectric Effects
Lastly, the piezoelectric effect refers to the electric charge generated by applying mechanical stress to certain materials, including ceramics. This phenomenon makes piezoelectric ceramics invaluable in sensors, actuators, and various consumer electronics.
This characteristic of ceramics allows for the conversion of mechanical energy to electrical energy, enhancing the efficiency of devices like microphones and pressure sensors. However, the specific properties of piezoelectric ceramics can be sensitive to environmental factors, which could affect their reliability.
Pros: Effective for innovative sensor applications.
Cons: Environmental sensitivity could impact long-term reliability.
In summary, the fundamental properties of ceramics shape their applicability and performance in numerous industries. Understanding these attributes—from mechanical traits like tensile strength and hardness to crucial thermal and electrical properties—helps drive innovation in ceramic technology.
Types of Ceramic Materials
Ceramic materials play a pivotal role in a spectrum of industries, owing to their unique properties and capabilities. Understanding the various types, including traditional and advanced ceramics, provides insights into how these materials can be optimized for specific applications. They are not just a collection of materials; each type comes with its own set of benefits and considerations that address a wide array of functional needs. In this section, we will dive into the replenished world of ceramic materials, exploring their distinction and relevance in modern technology.
Traditional Ceramics
Traditional ceramics, often termed as the foundation of ceramic technology, have been employed for centuries. Their time-tested characteristics lend them to diverse practical applications that span both daily utility and artistic expression.
Earthenware
Earthenware, a staple in traditional ceramics, is particularly notable for its versatility and ease of production. This type of ceramic is characterized by its porous body and is typically fired at lower temperatures.
Key characteristic: It can be easily molded and shaped, making it popular among artisans. Its ability to retain heat also contributes to its ongoing use in cooking and dining products, like rustic pottery and terracotta pots.
One unique feature of earthenware lies in its ability to absorb and retain moisture. While this can be seen as an advantage, particularly in maintaining the freshness of certain foods, it also limits its use in applications where impermeability is essential. Consequently, earthenware serves its purpose well in decorative and functional contexts but may not be suitable for more demanding applications like high-temperature cooking or liquid storage.
Stoneware
Stoneware offers another layer of complexity, striking a balance between durability and aesthetic appeal. This ceramic type is fired at higher temperatures than earthenware, resulting in a dense and non-porous material.
Key characteristic: Its robust nature makes it resistant to chipping and scratching, which is why it's favored for dinnerware and baking dishes.
A distinguishing feature of stoneware is its unique glazing capabilities. The glaze not only enhances visual appeal but also provides a non-stick surface and prevents absorption of liquids. This means that stoneware is often favored for both culinary and artistic purposes but can come at a higher cost and requires careful handling to avoid thermal shock.
Porcelain
Porcelain is often celebrated as the pinnacle of traditional ceramics, prized for its strength, translucence, and whiteness. Its production involves a combination of kaolin, feldspar, and quartz, fired at extremely high temperatures.
Key characteristic: The fine texture and aesthetic qualities make it appealing for luxury dinnerware and art pieces. Porcelain’s unique feature is its ability to maintain structural integrity even at elevated temperatures, making it suitable for various heating processes as, for instance, in laboratory glassware.
Despite its sophistication, porcelain requires delicate handling. It is prone to chipping if not treated with care, and it often comes at a considerably higher price point. In this way, while porcelain serves well in both artistic and functional roles, its temperatures and fragility can be daunting for some consumers.
Advanced Ceramics
Advanced ceramics, while emerging from the same family as traditional types, exhibit properties that lend them to technological advancements and specialized applications. Their unique structures and modifications make them significant in high-performance environments.
Bioceramics
Bioceramics refer to ceramics specifically designed for use in medical applications, such as bone implants and dental restorations. Their compatibility with biological tissues is a primary aspect of their design.
Key characteristic: They promote cell growth and can integrate with the human body, facilitating healing and improving quality of life for patients.
A notable feature is the ability to be engineered for specific osseointegration requirements, providing strength and longevity in demanding biological environments. However, production can be costly and requires meticulous regulation to ensure safety and efficacy.
Functional Ceramics
Functional ceramics represent a variety of materials that possess specific electrical, magnetic, or optical properties, allowing them to be used in advanced technological applications. They can be found in sensors, actuators, and capacitors.
Key characteristic: Their ability to withstand extreme conditions makes them indispensable in fields like electronics and aerospace.
One of the intriguing aspects of functional ceramics is the diversity of compositions that can lead to different functionalities, but managing the production processes to ensure uniformity can be challenging. Balancing performance and cost is crucial in maintaining their market relevance.
Nanoceramics
Nanoceramics are a cutting-edge advancement in ceramic technology, utilizing nanometer-sized materials to achieve superior performance characteristics. These materials often demonstrate enhanced strength and thermal stability.
Key characteristic: Their small size allows for unique properties, enabling applications in nanoelectronics and cutting-edge materials science.
A decisive advantage of nanoceramics comes from their potential to improve performance while reducing weight. Nevertheless, the production process can be complex and requires specialized technology. This complexity may lead to higher costs, posing a barrier for widespread adoption, yet the promise of transformative capabilities remains an area of active exploration.
Ceramic Processing Techniques
Ceramic processing techniques are the backbone of the entire ceramic manufacturing process. Through these techniques, raw materials are turned into finished products, catering to a multitude of applications from household items to high-tech components in electronics. Understanding the nuances of ceramic processing enables manufacturers to capitalize on various benefits while overcoming challenges related to quality and cost. The choice of processing technique directly impacts material properties, performance, and, subsequently, the application of the ceramics produced.
Powder Preparation
Milling
Milling is one critical step in the powder preparation phase of ceramic processing. This method reduces raw materials into fine particles, enabling a more homogeneous mixture that is easier to shape and sinter. The main characteristic that makes milling widely embraced is its ability to achieve precise particle sizes, enhancing the uniform distribution of powder in subsequent processes. Unlike other methods, milling permits the production of powders with different morphologies, thus giving manufacturers the flexibility to tailor the powder for specific applications.
However, milling does come with certain disadvantages. The energy input required can be significant, adding to operational costs. Additionally, the risk of contamination increases, especially if milling tools are not properly maintained.
Classification
Classification involves sorting the milled powders based on size and other characteristics. This step is crucial as it ensures that the particle size distribution is suitable for the subsequent processing stages. A key feature of classification is its focus on removing oversized or undersized particles that could compromise the integrity of the final product. The benefit of employing classification techniques is the enhancement in the uniformity of the ceramic body, leading to better mechanical properties. Nevertheless, classification can be a double-edged sword. The process can introduce additional costs and time delays, and if not accurately done, it may lead to an undesired particle size that defeats the purpose of milling.
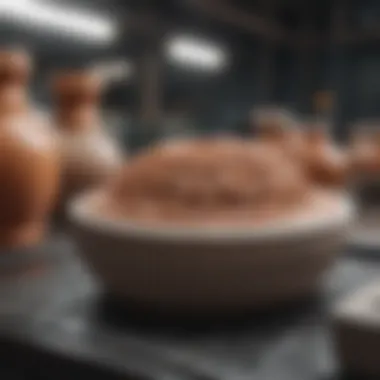
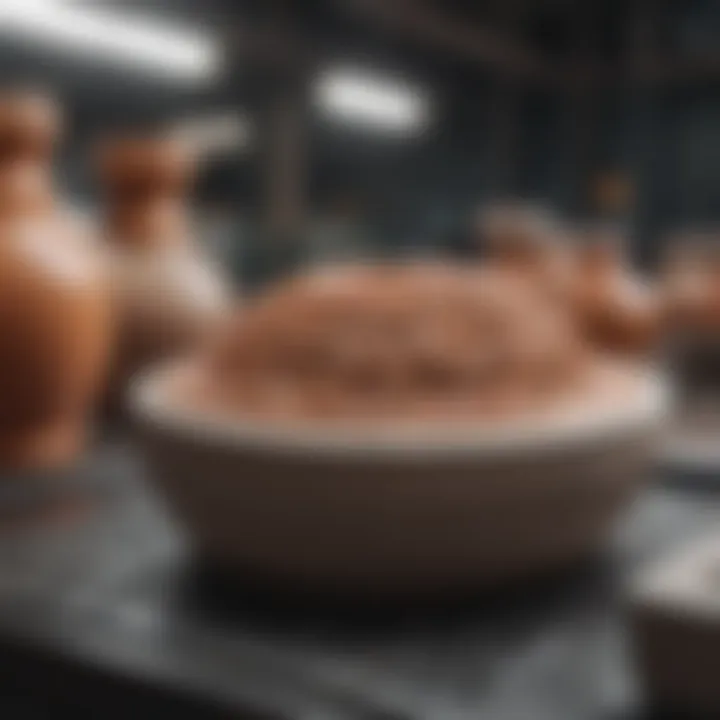
Granulation
Granulation is a technique employed to form granules or agglomerates from fine powders. This step has immense significance as it improves the flowability of the powder, making it easier to handle during subsequent forming processes. The characteristic that sets granulation apart is its ability to increase the density of powders, which in turn enhances compaction and reduces defects in the final ceramic body.
However, granulation may affect the final product's properties if not conducted carefully. Excessive moisture content can lead to clumping or undesired changes in particle shape, ultimately influencing sintering behavior.
Forming Processes
Pressing
Pressing, a common forming technique, involves compacting the granulated powders into desired shapes. The fundamental aspect of pressing centers on applying pressure to create denser and stronger compacts. It's often seen as a beneficial choice due to its effectiveness in producing consistent shapes efficiently. One unique advantage of pressing is its adaptability, as it can cater to a range of complex shapes while maintaining low material waste.
Yet, one of the challenges with pressing is ensuring uniform density throughout the compact. Variability can lead to structural weaknesses, making careful control of the pressing parameters essential.
Extrusion
Extrusion is another vital method used for creating ceramic products, especially for long shapes like tiles or pipes. The key characteristic of extrusion is the continuous flow of material through a die, enabling the production of complex geometries with precision. This technique is beneficial because it minimizes the number of defects in the resulting products and allows for higher production rates.
Yet, the downside of extrusion is its limited applicability on certain types of ceramic powders that might not flow well. Thus, selecting the right materials to extrude can sometimes prove tricky.
Casting
Casting involves pouring slurry into a mold, allowing the ceramic to take on the desired shape. This technique stands out due to its capability to produce intricate designs and complex geometries that may be challenging with other methods. It's particularly advantageous for creating larger ceramic parts with high dimensional accuracy.
However, casting also carries a risk. The drying process can lead to warping or cracking if not controlled properly. Also, the initial setup costs for molds can be significant, making it less attractive for small production runs.
Sintering
Conventional Sintering
Conventional sintering is a widely utilized method to densify ceramic powders into solid materials by applying heat without melting the powders. The primary advantage of this approach lies in its effectiveness in enhancing strength and lower porosity in ceramics. With its simplicity and relative cost-effectiveness, conventional sintering remains a popular choice in various manufacturing scenarios.
However, attaining uniformity in sintering can be challenging, as variations in temperature and atmosphere can lead to inconsistent properties in the final product.
Spark Plasma Sintering
Spark plasma sintering stands apart by using electric pulses to generate rapid heating, which can bring about significant improvements in mechanical properties and decrease processing times. This method's key feature is the ability to fabricate dense ceramics at lower temperatures compared to conventional methods. Consequently, this can lead to better preservation of microstructure and superior performance in the finished product.
Despite its advantages, spark plasma sintering is sometimes limited by the overhead costs and technical expertise required for operation.
Hot Isostatic Pressing
Hot isostatic pressing combines high temperature and pressure, providing an effective means to eliminate porosity in ceramics and enhance their structural integrity. The unique feature of this method is its isotropic properties, meaning it promotes uniformity across all directions, ultimately leading to stronger materials.
The challenge lies in the costs associated with implementing this technique, as it requires specialized equipment and conditions. Nonetheless, its ability to deliver high-performance ceramics makes it an attractive choice for industries demanding reliability and durability.
In summary, understanding ceramic processing techniques is paramount to unlocking the full potential of ceramic materials in various applications.
By mastering these technqiues, manufacturers can navigate challenges, create innovative products, and ensure that the various needs of diverse industries are met.
Innovations in Ceramic Technology
Innovations in ceramic technology have marked a significant evolution in material science, profoundly impacting various industries. The emphasis on this topic underscores how recent advancements push the boundaries of what ceramics can achieve. From enhancing performance in electronics to introducing smart functionalities in everyday applications, these innovations are not just improvements but transformation of standards and expectations. Understanding these developments allows for a deeper appreciation of ceramics' relevance in modern technological landscapes and hints at future possibilities.
3D Printing of Ceramics
Processes Involved
The processes involved in 3D printing ceramics signify a revolutionary step in manufacturing methods. This technique, often termed additive manufacturing, allows for the layer-by-layer creation of intricate structures that traditional methods cannot easily achieve. One of its standout features is the precision it offers, enabling designers to create complex geometries tailored for specific applications. This specific aspect of 3D printing plays a pivotal role in accelerating design cycles, allowing prototypes to be produced rapidly, which paves the way for innovative applications in industries from aerospace to biomedical fields.
This method isn't without its disadvantages, though. Managing the delicate balance between printing speed and quality can be tricky. Often, print resolutions can vary during the process, introducing imperfections that might be detrimental in highly sensitive applications. Regardless, its capacity for customization remains one of its most advantageous characteristics.
Advantages and Challenges
In exploring the advantages and challenges of 3D printing in ceramics, one must consider its potential to create low-volume, high-complexity part production. This represents a drastic shift from the economic viability of traditional mass production methods. Not only does it cater to bespoke designs, but it also aligns tightly with sustainable practices as it often produces less waste.
However, there are also challenges that cannot be overlooked. The cost of high-quality raw materials for 3D printing can be prohibitive, and not every ceramic material is suitable for this process. Moreover, the post-processing stage often demands intensive labor and fine-tuning, which may add to overall production time and costs.
Smart Ceramics
Functional Capacity
Smart ceramics embody the fusion of traditional ceramic materials with advanced technologies. These materials respond to external stimuli, enabling functionalities such as self-healing, shape memory, and even thermal regulation. A major point of interest in studying smart ceramics is their ability to adapt and interact with their environment, fostering innovations like impact-resistant structures in buildings or responsive medical implants.
This remarkable functional capacity positions smart ceramics as a game-changer in various fields. The unique feature of this technology lies in its adaptability—materials can offer specific responses to changes in temperature, pressure, or electrical fields, providing a valuable asset in multiple applications. Yet, the challenges persist; these advanced materials often require sophisticated manufacturing techniques and quality control processes that complicate scalability.
Applications in Industry
The applications of smart ceramics in industry are vast and varied. They include their use in sensors, actuators, and energy conversion devices, making them critical for fields such as telecommunications, aerospace, and healthcare. One key characteristic that highlights their importance is their lightweight nature combined with superior strength, making them ideal for reducing energy consumption in transportation while maintaining structural integrity.
Despite the impressive nature of these applications, they come with costs and complexity that can limit wider adoption. The initial investment in research and development can be high, and the integration of smart ceramics into existing systems requires comprehensive training for technicians and engineers. However, the ongoing efforts to reconcile these issues foreshadow even more innovative uses in the near future.
"The adaptability and advanced functionalities of ceramics only scratch the surface of their potential in modern tech."
These advancements represent not just changes in material characteristics, but a whole new arena of possibilities that enhance the performance and functionality of ceramics across sectors.
Applications of Ceramic Technology
Ceramic technology has showcased a myriad of applications, reflecting its versatility and capacity to address specific needs across various sectors. The importance of this topic lies in how ceramics enhance performance, contribute to sustainability, and drive innovation in design and functionality. Not only do ceramics serve practical purposes, but they also redefine the boundaries of what is achievable in material science today. This exploration of applications reveals significant benefits, such as their unique properties that are leveraged in diverse fields.
Ceramics in Electronics
Capacitors
Capacitors are essential components in electronic circuits, serving as energy storage devices that maintain electrical charge. One of the key characteristics of capacitors is their ability to discharge energy quickly when needed, making them invaluable for smoothing out voltage fluctuations in power supplies. Their reliable performance and compact size make them a common choice in various electronic applications, including consumer electronics and telecommunications.
A unique feature of these ceramic capacitors is their high dielectric strength, allowing them to handle high voltages without breaking down. However, the drawbacks come with the size and form factor; as capacitance values increase, capacitors can become noticeably larger or more costly. Nevertheless, their broad application makes them indispensable in modern electronics.
Dielectric Resonators
Dielectric resonators serve as miniaturized oscillators in electronic applications, particularly in radio frequency circuits. Their pivotal role lies in maintaining stable resonance frequencies, which is crucial for precise signal transmission. A standout property of dielectric resonators is their compactness paired with effective performance, fostering advancements in communication technology.
What makes these resonators particularly beneficial is their lower losses compared to traditional metallic resonators. However, they require careful handling due to their brittle nature. In essence, dielectric resonators exemplify how ceramics can elevate performance standards within the electronics sector.
Biomedical Applications
Bone Implants
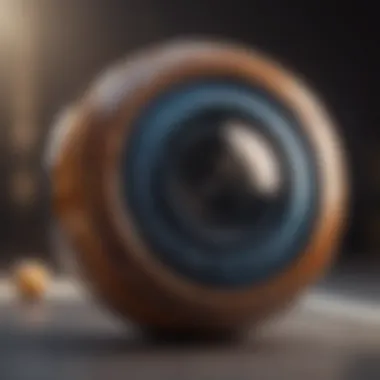
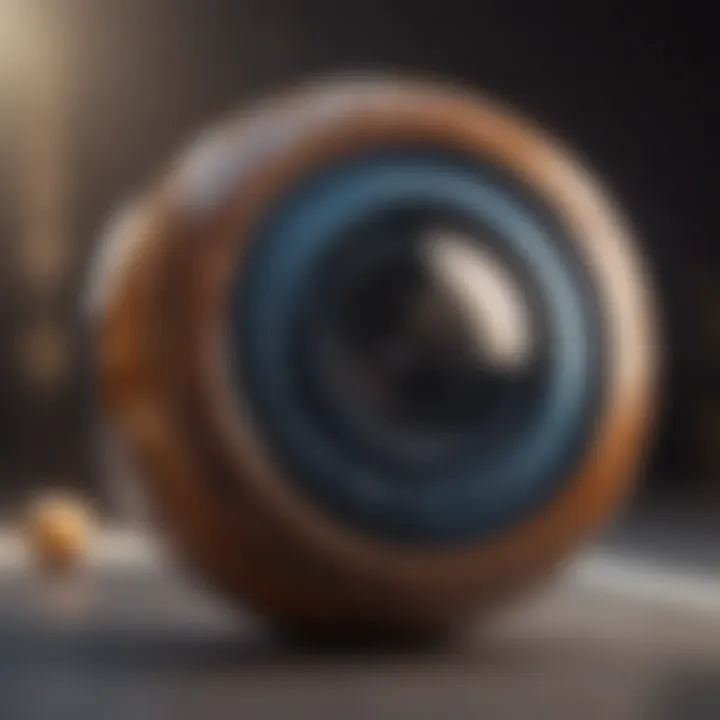
Ceramics have garnered significant attention in the biomedical field, especially in the context of bone implants. These implants replicate the natural properties of bone tissue, allowing for better integration with the human body. The primary characteristic of these bioceramics is their biocompatibility, which minimizes adverse reactions in patients.
A noteworthy feature of bioceramic materials is their ability to promote bone growth around the implant. This unique trait effectively addresses challenges faced with traditional metal implants, such as rejection or lack of integration. Despite their advantages, further research is needed to enhance the long-term durability of these implants in high-stress environments.
Dental Ceramics
Dental ceramics are another critical aspect of ceramic applications in healthcare, particularly for restorative work such as crowns and bridges. Their strong aesthetic appeal comes from their translucency and ability to mimic natural teeth. What sets dental ceramics apart is their toughness and resistance to wear, making them a popular choice for dental restorations.
However, while they are incredibly attractive and functional, they can be more brittle than other restoration materials. Balancing aesthetics and durability remains a crucial focus in the development of dental ceramics.
Aerospace and Automotive Uses
Heat Shields
Ceramic heat shields are vital components in aerospace engineering, designed to protect spacecraft during re-entry into the Earth’s atmosphere. Their standout feature is their exceptional heat resistance, allowing them to withstand extreme temperatures while remaining lightweight. This characteristic is crucial, as any additional weight can significantly affect the performance and efficiency of a spacecraft.
On the downside, the rigidity of ceramics can make them susceptible to cracking under mechanical stress. Thus, careful design and material selection are essential to ensure their effectiveness in these high-stakes situations.
Ceramic Matrix Composites
In the quest for superior materials in aerospace and automotive applications, ceramic matrix composites have emerged as a robust solution. These materials combine ceramics with matrix materials such as carbon, resulting in high-performance solutions that boast superior strength and thermal resistance.
The unique advantage of ceramic matrix composites is their ability to maintain structural integrity at elevated temperatures, which is a necessity in aerospace environments. However, the production processes can be complicated and costly, creating barriers for broader adoption in the automotive industry.
In summary, the applications of ceramic technology demonstrate its remarkable adaptability and efficiency across varied fields, paving the way for further advancements and increased integration into modern technologies. In understanding these applications, one can truly appreciate the pivotal roles ceramics play in enhancing performance, safety, and sustainability across sectors.
Challenges in Ceramic Technology
The exploration of ceramic technology doesn't come without hurdles. Understanding the challenges associated with ceramics is crucial. These challenges can dictate the pace at which innovations transform into practical solutions across industries. Each problem presents unique obstacles that technologists and researchers need to address to harness the full potential of ceramic materials.
Material Limitations
Ceramics are renowned for their hardness and heat resistance. However, these traits can be a double-edged sword. For instance, while ceramics boast high compressive strength, their tensile strength often lags behind that of metals. This imbalance can lead to brittleness under stress, making certain applications risky.
Additionally, ceramics typically have limitations in terms of fracture toughness, which can restrict their usage in environments that require high-impact resistance. As we push the boundaries of ceramic applications, the quest for materials that balance strength with versatility becomes increasingly important.
Cost-Effectiveness
In the realm of ceramic production, high-quality materials and advanced fabrication processes often come with a hefty price tag. This raises significant questions about economic viability. For example, while advanced ceramics can outperform traditional ones in terms of performance and durability, the high initial production costs can deter widespread adoption. Businesses might favor cost-effective alternatives even if they do not meet long-term needs.
An astute analysis of the total cost of ownership often reveals that investing in superior ceramics could lead to long-term savings. However, the initial barriers are daunting. Organizations must weigh immediate financial constraints against potential future benefits. Implementing innovative manufacturing techniques that reduce costs without sacrificing quality is an ongoing pursuit in the industry.
Sustainability Concerns
Sustainability is a pressing concern across all sectors, including ceramic technology. The extraction and processing of raw materials can leave a huge ecological footprint. For example, mining practices, which can disrupt local ecosystems, are a key area of concern.
Moreover, many ceramics are not biodegradable. Their lengthy decomposition periods pose environmental challenges once products reach the end of their lifecycle. This reality has prompted a movement towards exploring eco-friendly materials and recycling methods. Researchers are investigating ways to create sustainable alternatives that don’t compromise performance. The shift toward greener practices is not just a trend but a necessary evolution for the survival of the industry.
"The future of ceramics lies not only in their capability to enhance technology but also in their potential to harmonize with the environment."
In summary, the journey of ceramic technology is paved with challenges that spark innovation. Addressing material limitations, ensuring cost-effectiveness, and championing sustainability are essential for the viability and growth of ceramics in the future. As we aim to resolve these issues, the advancement of ceramic technology will continue to soar, yielding benefits across multiple sectors.
The Future of Ceramic Technology
The realm of ceramic technology is ever-evolving, characterized by both challenges and opportunities. As we look ahead, it's clear that the trajectory of this field is nothing short of promising. With remarkable advancements in materials science, manufacturing processes, and applications across various sectors, the future of ceramic technology holds considerable potential for innovation and growth. This section discusses the significance of these developments while providing insights into prospective outcomes of research and applications in the industry.
Research and Development Trends
In recent years, there has been a noticeable shift in research and development within ceramic technology. The focus is now on creating materials that meet the demands of contemporary applications. One area of keen interest is smart ceramics, which integrate functionalities like self-healing and shape-memory capabilities. Innovations in nanoparticle synthesis are also gaining traction, leading to ceramics with enhanced chemical and mechanical properties.
The advent of additive manufacturing, often referred to as 3D printing, has revolutionized how ceramics are processed. This technique allows for complex geometries that were previously unattainable, resulting in bespoke designs for specific applications. Future research is likely to delve deeper into hybrid materials, combining ceramics with polymers or metals to create composites with superior performance.
Potential Market Growth
As industries recognize the benefits and versatility of ceramic materials, market growth seems inevitable. According to various research reports, the global ceramic market is projected to expand significantly in the coming years. The demand in sectors like electronics, biomedical, and aerospace is driving this growth. Companies are increasingly investing in sophisticated ceramic products to capitalize on performance and durability advantages.
Factors like urbanization and the need for sustainable materials are propelling this market. As the focus shifts towards reducing carbon footprints, ceramics are favored due to their energy-efficient production processes.
"The ceramic industry stands at a pivotal point, where innovation meets necessity. Future trends indicate a shift towards eco-friendliness and transformative applications."
The potential for customized applications in industries, particularly in the automotive sector, suggests that ceramics will play a crucial role in manufacturing lighter and more efficient vehicles in the future.
Interdisciplinary Applications
The future of ceramic technology isn't just confined to traditional uses. Interdisciplinary applications are burgeoning, integrating ceramics into new domains. For instance, ceramics are finding their way into construction through engineered materials that enhance the structural integrity and energy efficiency of buildings.
In IT and telecommunications, ceramic substrates are used in high-frequency circuits, benefiting the production of faster and more reliable devices. The integration of ceramic components into renewable energy technologies, like solar panels and batteries, highlights their growing relevance in addressing today's energy challenges.
Furthermore, the collaboration between different disciplines like biology and materials science is giving rise to innovative bioceramics for advanced medical implants. These developments underscore the adaptability of ceramic technology in meeting diverse and evolving needs across sectors.
In summary, the future of ceramic technology embodies a broad spectrum of possibilities. With ongoing research, a growing market demand, and interdisciplinary collaborations, the outlook is favorable. The advancement in this field reflects not just technological progress but also a response to the pressing demands of modern society.
End
Ceramic technology stands as a beacon of innovation and utility in contemporary industries. In this article, we’ve examined not only the intricacies of ceramic materials but also how their applications have revolutionized areas like electronics, medicine, and aerospace. The summarization of key points highlights that ceramics possess unique properties such as high strength, thermal stability, and electrical insulation, making them vital in the design and functionality of modern devices.
Focusing on advancements, we witnessed the rise of methods like 3D printing, which has redefined the way ceramics are manufactured—allowing for greater customization and efficiency. These innovations also hint at a promising future wherein ceramics can adapt and evolve alongside technological needs. However, challenges like material limitations and sustainability must be navigated with care.
"Innovation does not have to come from scratch, but rather can build upon existing materials, like ceramics, to enhance their utility."
The thoughtful consideration of these aspects ensures that the role ceramics play will continue expanding, integrating more seamlessly into our daily lives. This conclusion serves as a reminder of the potential held within ceramic technology—it is an evolving field, ripe with opportunities for further research and applications.
Summary of Key Points
- Unique Properties of Ceramics: Ceramics exhibit remarkable characteristics such as high compressive strength, low thermal conductivity, and excellent resistance to oxidation. These properties facilitate their application in diverse fields.
- Innovative Manufacturing Techniques: Recent advancements like 3D printing have opened new avenues for production and application, enabling better precision and functional diversity in ceramic products.
- Multifaceted Applications: The use of ceramics extends across various sectors— from high-tech electronics (like capacitors) to crucial medical implants, underlining their versatility.
- Present Challenges: Despite their advantages, ceramics face hurdles such as high production costs and issues surrounding sustainability, which must be addressed for broader adoption in future applications.
Final Thoughts on Ceramic Technology
Ceramic technology reflects a microcosm of human ingenuity, showcasing how traditional materials can gain mainstream importance through innovation. As the demand for specific applications grows, there will undoubtedly be an expanding need for research that pushes the boundaries of existing ceramic properties. Strengthening knowledge about both advantages and limitations empowers researchers and professionals alike to make informed decisions that shape the future of this material.
The path ahead for ceramics seems promising. A synergy between technological advancements and traditional practices will ensure that ceramics not only remain relevant but thrive in an increasingly material-dependent world. Embracing these nuances can ultimately lead to groundbreaking solutions that cater to the future needs of various industries, solidifying ceramics as a cornerstone of modern technology.