Hydrogen Production Processes: An In-Depth Analysis
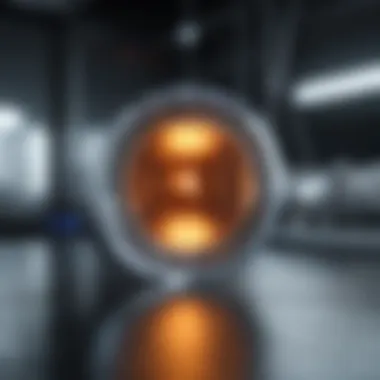
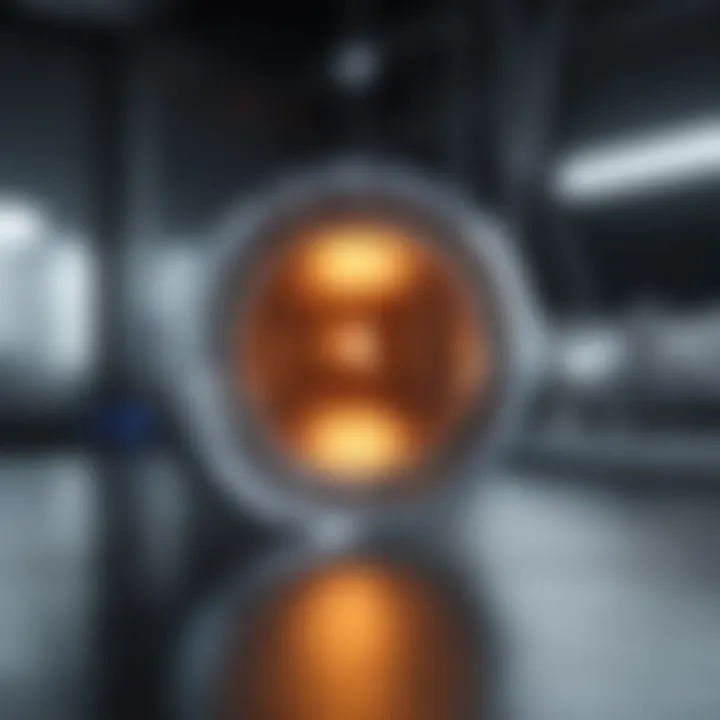
Intro
Hydrogen, as a clean energy carrier, is gaining notable attention in the global quest for sustainable energy solutions. Its production processes vary significantly, and each method presents distinct advantages, limitations, and environmental implications. With the urgency to transition from fossil fuels, understanding these processes becomes crucial for researchers, educators, and professionals in energy innovation.
Different hydrogen production methodologies are in a constant state of development, making this area ripe for exploration. The environmental impacts of these processes also play a vital role in their viability as long-term solutions. Furthermore, emerging technologies promise to enhance efficiency, making hydrogen a more accessible resource. In this overview, we will dissect the primary production methods, delve into their efficiencies and applications, and explore ongoing advancements that hold the potential for a more sustainable energy future.
Prologue to Hydrogen Production
Hydrogen is often considered a key player in the transition towards a sustainable energy future. Its versatile nature and potential for application across diverse sectors make it crucial for various energy strategies. Understanding hydrogen production processes is vital, as they lay the groundwork for harnessing this clean fuel efficiently and sustainably.
In this article, we will explore how hydrogen can serve as a clean energy carrier, its production methodologies, and the impact of these processes on the environment. The significance of this topic lies in the urgent need to address climate change and energy demands, positioning hydrogen not just as a component but a potential cornerstone of future energy systems.
The Role of Hydrogen in Energy Transition
Hydrogen plays a central role in energy transition strategies for several reasons. First, it can be produced from renewable resources, meaning it has the potential to be a carbon-free energy source. This is particularly important in the context of global efforts to reduce greenhouse gas emissions. Hydrogen can be used in fuel cells, which are known for their high efficiency in converting chemical energy to electricity without combustion.
Moreover, hydrogen offers a solution for energy storage, which is critical for balancing the intermittent nature of renewable energy sources like wind and solar. This storage capability allows for better management of energy supply and demand, increasing the resilience of energy systems.
Additionally, hydrogen can be integrated into existing natural gas infrastructure, making it easier for societies to incorporate this technology without major overhauls of current systems. By doing so, hydrogen can facilitate a smoother transition from fossil fuels to clean energy.
A Brief History of Hydrogen Utilization
Hydrogen has a long history of utilization, dating back to the late 18th century when it was first identified as a distinct element. Initially, hydrogen was primarily recognized for its role in combustion, but over the years its applications have evolved significantly.
In the early 20th century, hydrogen began to be used in various industrial applications, particularly in the synthesis of ammonia for fertilizers through the Haber process. The hydrogen economy has been slowly gaining attention since the 1970s, especially during the oil crises, prompting researchers and governments to explore alternative energy sources.
As technology progressed, the resurgence of interest in hydrogen was seen in the late 20th century with the development of the hydrogen fuel cell. This technological advancement has sparked new potential applications, especially in transportation and stationary power generation. Today, hydrogen is increasingly seen not just as a fuel, but as a critical component in achieving energy systems that are both sustainable and efficient.
"Hydrogen has the potential to play a transformative role in our energy landscape. It can bridge the gap between renewable energy production and consumption."
Fundamentals of Hydrogen Chemistry
The understanding of hydrogen chemistry provides a vital foundation for exploring hydrogen production processes. Hydrogen, being the simplest and most abundant element in the universe, possesses unique chemical properties that play a crucial role in various chemical reactions. Grasping the fundamentals of hydrogen allows researchers and professionals to assess the feasibility and efficiency of different production methods.
Chemical properties of hydrogen include its tendency to form covalent bonds, its high energy content per mass, and its reactivity with other elements. These attributes make hydrogen a promising candidate for clean energy applications. For instance, when hydrogen combusts, it primarily produces water as a by-product, leading many to consider it a clean fuel alternative. Furthermore, hydrogen can serve as an energy carrier, facilitating the storage and transportation of energy generated from renewable sources.
Chemical Properties of Hydrogen
Hydrogen is colorless, odorless, and tasteless in its gaseous form. At room temperature and pressure, it exists as a diatomic molecule, Hβ. Its low density and small size enable it to diffuse quickly in the atmosphere. Some key chemical properties include:
- Reactivity: Hydrogen reacts easily with numerous elements and compounds, forming a wide array of hydrides.
- Energy Content: When burned, hydrogen releases significant amounts of energy, making it an efficient fuel source.
- Acid-Base Behavior: Hydrogen ions (HβΊ) play a fundamental role in acid-base chemistry. In aqueous solutions, they determine the acidity or alkalinity of the solution.
These properties pave the way for various applications in energy production and consumption. Successful exploitation of these characteristics is essential for developing innovative hydrogen production technologies.
Hydrogen Isotopes and Their Applications
Hydrogen has three known isotopes: protium, deuterium, and tritium. Protium is the most common form, containing no neutrons. Deuterium has one neutron and is often used in nuclear reactors due to its capacity to undergo nuclear fission. Tritium, which has two neutrons, is radioactive and less common. It holds significant potential in nuclear fusion research.
The unique properties of these isotopes enable their application across various fields:
- Nuclear Energy: Deuterium and tritium can be used as fuel in nuclear fusion, potentially serving as a clean energy source in the future.
- Research and Industry: Deuterium is used in chemical labeling and tracing studies, aiding scientists in tracking molecular mechanisms.
- Medical Applications: Tritium is used in certain medical diagnostics and therapies, albeit under stringent regulatory frameworks due to its radioactive nature.
Hydrogen isotopes contribute to advancements in energy production, research, and healthcare, thereby illustrating the significance of understanding hydrogen chemistry in the broader context of hydrogen production processes.
Traditional Hydrogen Production Methods
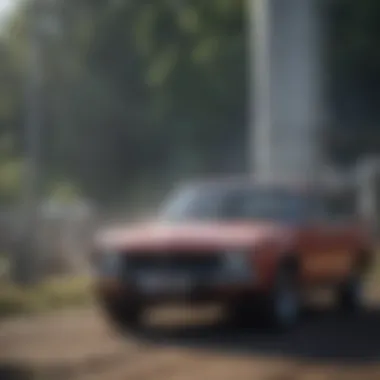
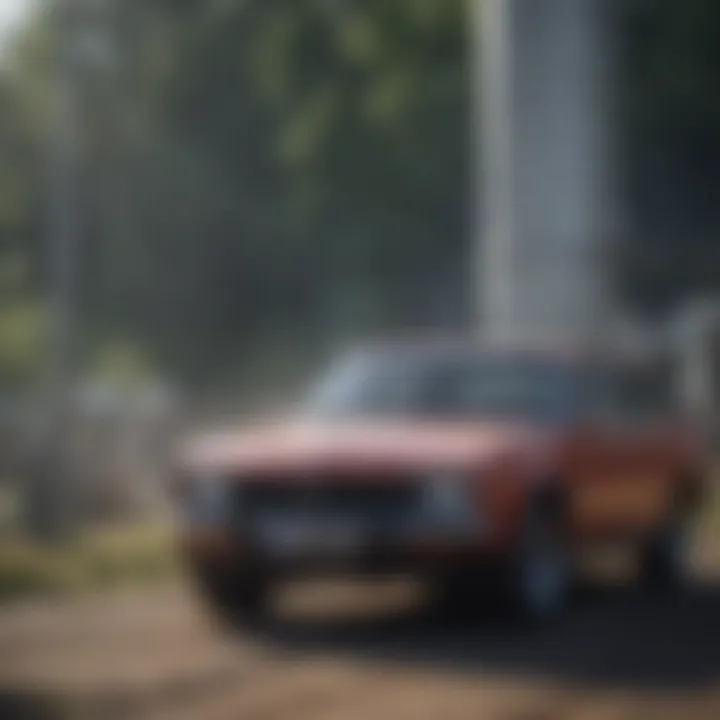
Traditional hydrogen production methods have been essential in meeting industrial demands for hydrogen, particularly in sectors such as oil refining, chemical manufacturing, and metallurgy. As hydrogen emerges as a viable solution for clean energy, understanding these methods becomes increasingly vital. The main benefit of these techniques lies in their maturity and economic viability, which allow for large-scale production. However, alongside their advantages, considerations regarding carbon emissions and sustainability must also be evaluated.
Steam Methane Reforming
Steam Methane Reforming (SMR) is the most widely used method for hydrogen production, accounting for a significant portion of global hydrogen output. In SMR, methane from natural gas reacts with steam under high temperatures in the presence of a catalyst, typically nickel. This process yields hydrogen and carbon monoxide as primary products.
Key Characteristics of SMR:
- Efficiency: SMR is known for its high efficiency, often exceeding 70% in converting methane to hydrogen.
- Cost-Effectiveness: The relatively low market price of natural gas makes SMR economically attractive.
- Carbon Emission: One major downside is that this process generates substantial carbon dioxide as a byproduct, which raises environmental concerns.
The efficiency of SMR can be enhanced through technologies such as carbon capture and storage. Such innovations aim to make SMR more environmentally friendly while maintaining its economic benefits.
Coal Gasification
Another traditional method for hydrogen production is coal gasification. This technique involves converting coal into a gas mixture known as synthesis gas or syngas, which primarily consists of hydrogen and carbon monoxide. The gasification process occurs in a reactor at high temperatures and pressures, often with the introduction of steam and oxygen.
Advantages of Coal Gasification:
- Abundance of Resources: Coal is widely available, especially in regions with significant coal deposits.
- Fuel Diversification: Coal gasification allows for diversification in fuel sources for hydrogen production.
- Potential for Carbon Capture: Similar to SMR, this method can integrate carbon capture technologies to minimize emissions.
Despite these benefits, coal gasification faces criticism due to its environmental impact and the finite nature of coal reserves. As such, it is essential to weigh its viability against cleaner alternatives.
Water Electrolysis
Water electrolysis is an increasingly popular method for hydrogen production, especially in the context of renewable energy integration. In this process, an electric current is applied to water, causing it to dissociate into hydrogen and oxygen gases. Importantly, the source of electricity is crucial for determining the overall sustainability of this method.
Key Considerations for Water Electrolysis:
- Renewable Energy Compatibility: Electrolysis can utilize electricity generated from renewable sources, like wind or solar power, making it a cleaner alternative.
- Scalability: This method can be scaled to meet various production needs, making it suitable for both small and large applications.
- Efficiency Levels: While current efficiencies vary, ongoing research focuses on improving the energy input required for the electrolysis process.
Water electrolysis represents a promising pathway for a hydrogen economy, particularly as advancements continue in both efficiency and renewable energy integration.
The choice of hydrogen production method influences not only cost and efficiency but also environmental impacts, making it a subject of critical importance in the energy transition.
Emerging Hydrogen Production Technologies
Emerging hydrogen production technologies represent a pivotal shift in the landscape of energy generation. As the global focus on sustainability intensifies, these innovations promise to augment traditional methods of hydrogen production. The benefits of these technologies are manifold, offering pathways that might enhance efficiency and reduce environmental impacts. One key aspect of these methods is their potential to utilize renewable resources, thus lowering reliance on fossil fuels. This section will explore three promising technologies: biomass gasification, photocatalytic water splitting, and thermochemical water splitting.
Biomass Gasification
Biomass gasification converts organic materials into hydrogen gas and other valuable by-products. This process involves the thermal decomposition of biomass in a low-oxygen environment, which maximizes the yield of gaseous products.
Key benefits of biomass gasification include:
- Sustainability: Utilizes waste materials, therefore reducing landfill use.
- Carbon Neutrality: Biomass can be considered carbon neutral, as the carbon dioxide released during gasification is roughly equal to that absorbed during the growth of the plants.
- Diverse Feedstocks: A wide range of materials, such as agricultural waste, wood chips, and municipal solid waste, can be used as feedstocks.
However, challenges persist. The economic viability of biomass gasification often hinges on feedstock availability and transportation costs. Despite these hurdles, research into optimizing the gasification process continues, enhancing yield and efficiency.
Photocatalytic Water Splitting
Photocatalytic water splitting is an emerging technology that employs sunlight to drive the chemical reaction necessary for producing hydrogen from water. This process integrates semiconductor materials as catalysts, which absorb light and facilitate the splitting of water molecules into hydrogen and oxygen.
The significance of this method lies in its alignment with renewable energy. Some of the advantages include:
- Low Energy Input: Utilizes sunlight, drastically reducing operational energy costs.
- Scalability: Potential for large-scale operations as solar materials become more efficient and widely available.
- Environmentally Friendly: Produces hydrogen without harmful emissions.
Currently, the main limitation of photocatalytic water splitting is the efficiency of existing photocatalysts. Researchers aim to develop new materials that can absorb a broader spectrum of sunlight and enhance reaction rates.
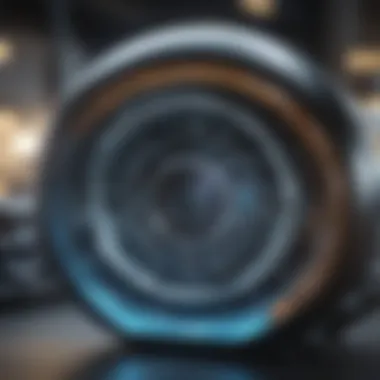
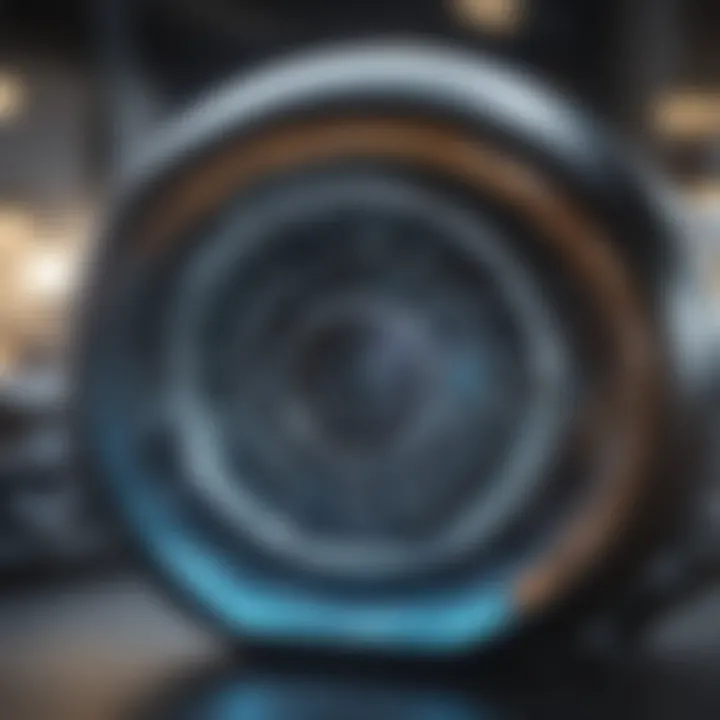
Thermochemical Water Splitting
Thermochemical water splitting is a high-temperature method that utilizes heat from solar or nuclear sources to break down water molecules. The process typically involves a series of chemical reactions which convert water into hydrogen and oxygen.
This method presents several advantages:
- High Efficiency: Can achieve efficiencies greater than traditional electrolysis.
- Heat Utilization: Takes advantage of high-temperature heat sources, enhancing overall system efficiency.
- Lower Capital Costs: Potential for reduced costs compared to electrolysis in large-scale applications.
However, the development of suitable materials that can withstand extreme temperatures remains a challenge. Continuous innovation in materials science will be essential to further advance thermochemical water splitting technologies.
In summary, emerging hydrogen production technologies are not only vital for expanding the hydrogen economy but are also instrumental in facilitating the transition towards a more sustainable energy future. Each method presents its unique set of advantages and challenges, showcasing the diverse approaches needed to optimize hydrogen production.
Comparative Analysis of Hydrogen Production Methods
A comparative analysis of hydrogen production methods is essential in understanding the viability and impact of various techniques in today's energy landscape. This section investigates the effectiveness, economic implications, and environmental consequences of established and emerging hydrogen production methodologies. By evaluating these factors, we can better inform decisions surrounding energy policy, technology development, and sustainability efforts.
Efficiency Metrics
Efficiency is a crucial metric when assessing hydrogen production methods. It refers to the ratio of useful energy output to the energy input required for production. For example, steam methane reforming is one of the most widely used techniques, with efficiencies often ranging from 60% to 80%. In contrast, water electrolysis typically has lower efficiencies, around 50% to 70%, depending on the technology and energy source used.
The efficiency of various processes can significantly influence their economic viability and environmental impact. Higher efficiency means less input energy is needed, thereby reducing operational costs. Moreover, advancements in technology continue to improve these metrics. Technologies like advanced electrolysis or thermochemical cycles show promise in increasing overall efficiency, moving closer to the ideal goals set by researchers.
Economic Considerations
The economic aspect of hydrogen production cannot be overlooked. The cost of hydrogen production is influenced by several factors, including raw material prices, energy source costs, and capital expenditure for plant setup. Traditional methods like steam methane reforming have historically been more cost-effective due to the abundant supply of natural gas. However, as the demand for green hydrogen increases, techniques such as water electrolysis powered by renewable sources are expected to become more economically viable.
Investments in research and development are essential for bringing costs down. For instance, reducing the cost of electrolyzers through innovative designs or cheaper materials can lead to significant decreases in overall hydrogen production costs. Additionally, government policies and subsidies will play a vital role in shaping the economics of different production methods, supporting the transition to cleaner alternatives.
Environmental Impact Assessment
An environmental impact assessment is critical in evaluating the sustainability of hydrogen production methods. Each technique has varying degrees of impact on land, water, and air quality. For example, steam methane reforming emits significant amounts of carbon dioxide, contributing to climate change. In contrast, water electrolysis, especially when utilizing renewable energy, produces no direct emissions, making it a more environmentally friendly option.
Moreover, biomass gasification offers a pathway to utilize waste materials but must be carefully managed to prevent negative environmental consequences such as deforestation or biodiversity loss. Emerging technologies like thermochemical water splitting also need to undergo rigorous environmental evaluations to determine their long-term impact.
As the global push for sustainable energy increases, understanding the comparative environmental impacts of hydrogen production methods will be vital for developing effective policies.
In summary, a thorough comparative analysis of hydrogen production methods is essential for guiding future research, policy decisions, and investment strategies. By focusing on efficiency metrics, economic considerations, and environmental impact, stakeholders can make informed choices that will shape the future of hydrogen as a clean energy carrier.
Integration of Hydrogen Production and Renewable Energy
The integration of hydrogen production with renewable energy sources is a crucial topic in the ongoing discussion about sustainable energy solutions. As the world seeks to reduce its reliance on fossil fuels, combining hydrogen production with renewables can help address both energy demands and environmental concerns. Hydrogen, being a versatile energy carrier, can contribute significantly to achieving a low-carbon economy.
One of the main benefits of integrating hydrogen production with renewable energies like solar and wind is that it enables the storage of excess energy that might otherwise go unused. For instance, during peak production times, renewable sources can generate surplus electricity. This surplus can be employed to produce hydrogen through processes like water electrolysis, thus transforming surplus energy into storable hydrogen fuel. This not only enhances the utility of renewable sources but also provides a buffer during low production periods.
Another significant consideration is the reduction of greenhouse gas emissions. Hydrogen produced from renewable energy is often considered green hydrogen. Unlike hydrogen derived from fossil fuels, green hydrogen does not contribute to carbon emissions, making it an ideal option for mitigating climate change. This method supports regulations addressing environmental impacts, as it aligns with the commitments many countries are making towards net-zero goals.
Furthermore, integrating hydrogen production with renewable energy can also lead to economic benefits. Investments in hydrogen infrastructure stimulate job creation and can support rural economies, especially where wind farms or solar plants are located. This opens pathways for innovative technology collaborations as companies seek efficient methods for hydrogen production. In summary, integrating hydrogen production with renewable energy not only supports sustainable practices but also paves the way for economic growth in the energy sector.
Solar Energy for Hydrogen Generation
Solar energy presents a promising avenue for hydrogen production. Utilizing solar power through photovoltaic panels, hydrogen can be generated via electrolysis of water. When sunlight hits the solar cells, electricity is produced, which is then used to split water into hydrogen and oxygen. This method effectively leverages an abundant resource, optimizing the hydrogen production process when sunlight is available. However, solar energy's intermittent nature necessitates careful planning and energy storage solutions to ensure consistent hydrogen supply.
Some researchers are working on more effective photovoltaic technologies that can increase the efficiency of solar panels, leading to higher hydrogen output. Systems such as concentrated solar power can also be utilized where sunlight is focused to generate high temperatures to facilitate chemical reactions yielding hydrogen. The dual benefit of power generation alongside hydrogen production positions solar energy as a vital component of the hydrogen economy.
Wind Energy Utilization in Electrolysis
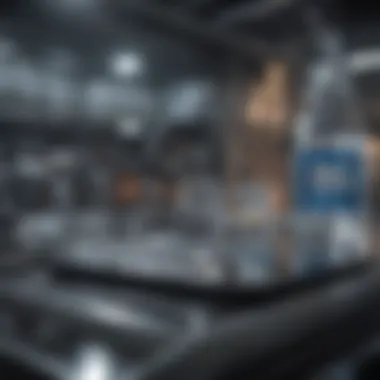
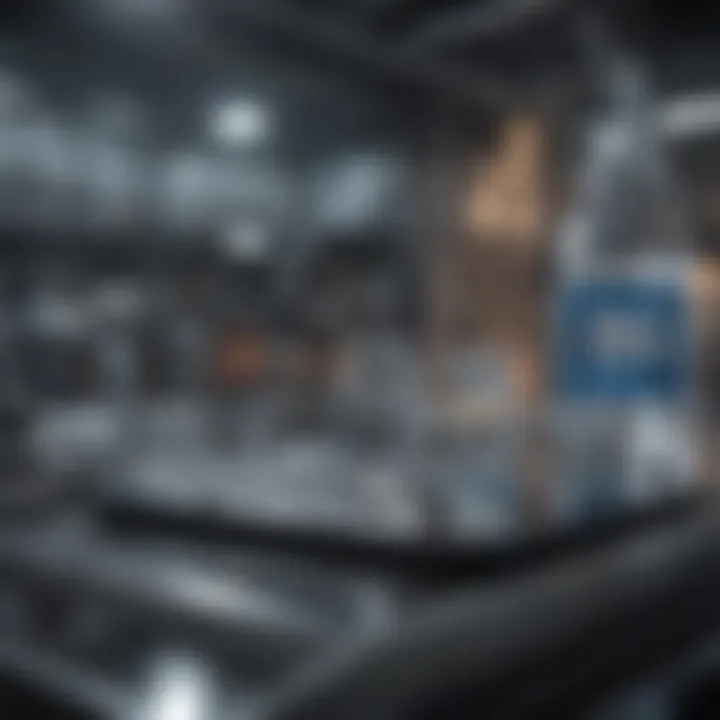
Wind energy also plays a critical role in hydrogen production. Similar to solar energy, electricity generated from wind turbines can be directed towards water electrolysis to produce hydrogen. Wind farms typically operate in remote areas, which may limit grid access. Here, the ability to convert wind energy into hydrogen allows for transport and storage. The hydrogen thus produced can be used on-site or transported to where it is needed, thereby alleviating some of the congestion issues seen with renewable electricity.
As wind technology advances, innovative turbine designs are allowing for more efficient energy extraction even at low wind speeds. Furthermore, hybrid systems merging wind energy with other forms of renewable energy present great potential for hydrogen production. This flexibility is essential as energy demands increase and calls for sustainability grow louder across the globe.
Implications for Policy and Regulation
In the realm of hydrogen production, policy and regulation play crucial roles in determining the trajectory of technological advancements and market adoption. The increasing awareness of climate change and the urgent need for sustainable energy solutions have made hydrogen a focal point in energy discussions. Governments worldwide are recognizing the importance of clear frameworks and incentives to boost hydrogen production and utilization. Such frameworks are not only essential for industry stakeholders but also for achieving global climate goals.
Government Incentives and Support
Incentives from governments can significantly enhance hydrogen production processes. Financial support can take various forms, including grants, tax credits, and subsidies. Here are some critical points about the role of government incentives:
- Funding Research and Development: Governments can fund research initiatives aimed at improving hydrogen production efficiency. Many cutting-edge technologies require substantial upfront investment, and funding can bridge that gap.
- Stimulating Private Investment: By offering incentives, governments can stimulate private sector investment. When companies perceive government support as a sign of stability and growth potential in the hydrogen market, they are more likely to invest.
- Facilitating Infrastructure Development: Incentives can also support the development of necessary infrastructure, such as hydrogen refueling stations and pipelines. This infrastructure is crucial for a robust hydrogen economy.
- Encouraging Collaboration: Programs that promote collaboration between government, industry, and academia can lead to shared knowledge and innovation. For example, joint ventures can help leverage resources and expertise while reducing risks.
Thus, government incentives serve as a catalyst for accelerating the adoption of hydrogen technologies.
International Hydrogen Economy Policies
The complexity of the global energy landscape necessitates international cooperation in hydrogen policy-making. Such cooperation can foster a consistent regulatory framework and support the development of a hydrogen economy across borders. Here are some key aspects of international policies:
- Creating Standardized Regulations: Harmonizing regulations can simplify operations for companies working in multiple countries. Standardized safety and quality regulations can facilitate trade and ensure interoperability of hydrogen technologies.
- Promoting Clean Energy Trade: International policies can also focus on creating markets for hydrogen exports. Countries rich in renewable resources can sell hydrogen to energy-importing nations, thus enhancing energy security and fostering economic growth.
- Cooperative Research Initiatives: Establishing international platforms for collaborative research can lead to faster advancements in hydrogen production technologies. Knowledge sharing can accelerate problem-solving and innovation.
"Policies must evolve to reflect the unique characteristics of the hydrogen economy and its potential to contribute to global sustainability efforts."
Overall, the implications for policy and regulation in hydrogen production highlight its importance in shaping the future of energy. With thoughtful incentives and international cooperation, the path to a hydrogen economy can be paved, promoting sustainability and energy security.
Future Prospects in Hydrogen Production
The future of hydrogen production is critical to achieving global energy transition goals. Its relevance is largely tied to the increasing focus on reducing carbon emissions and dependence on fossil fuels. Hydrogen stands out as a potential clean energy carrier, with various production methods being explored and improved. This section seeks to delve into the specific elemental considerations regarding future prospects and their implications for both industry and policy.
Trends in Research and Development
Ongoing research and development in hydrogen production is noteworthy. A significant trend is the enhancement of renewable-powered methods such as electrolysis, where researchers are aiming to increase efficiency. Technologies like proton exchange membrane electrolysis and alkaline water electrolysis are becoming focal points.
Another trend is the integration of hydrogen production with energy systems. Hybrid systems that combine solar or wind energy with hydrogen production methods appear promising. The focus on reducing costs remains paramount. Advanced materials for better catalysts and membranes can lower the overall expense of electrolysis processes. Developing bioreactors for biological hydrogen production represents another innovative path.
"The advancement in materials science will determine the pace of hydrogen production evolution."
Challenges and Opportunities
The future of hydrogen production is not without challenges. Cost-effective production is still a barrier. Many existing methods of hydrogen production are expensive and reliant on substantial initial investments. Another challenge includes the storage and transportation of hydrogen, which requires robust and safe systems.
However, opportunities abound. The push for green hydrogen, produced exclusively from renewable resources, opens new avenues. Increased public and private investments in this sector are creating an environment ripe for innovation.
Moreover, the alignment of hydrogen resources with electric grids can capitalize on surplus renewable energy. Developing policies that encourage a hydrogen economy can also trigger state and federal initiatives to enhance production capabilities.
In summary, the future of hydrogen production offers a blend of complex challenges and exciting opportunities. With advancements in technology and supportive legislation, the pathway to a sustainable hydrogen economy appears increasingly feasible.
Culmination
In reviewing the various hydrogen production processes, it becomes evident why this topic holds significant importance in contemporary energy discourse. The potential of hydrogen as a clean energy carrier is profound. As nations move towards decarbonization, understanding these methods is crucial.
Key Elements:
The article delves into established techniques like steam methane reforming and water electrolysis, as well as emerging technologies like biomass gasification. Each method has its efficiencies and shortcomings. Recognizing these nuances allows policymakers and researchers to make informed decisions regarding future investments.
Benefits of Hydrogen Production:
- Environmental Impact: Hydrogen can significantly reduce greenhouse gas emissions when sourced sustainably.
- Energy Security: Producing hydrogen domestically can help nations rely less on foreign energy sources.
- Economic Opportunities: Advancements in hydrogen production technology can create new jobs and industries.
Considerations:
While exciting prospects exist, challenges remain. Technological barriers, economic feasibility, and infrastructure requirements must be addressed. Furthermore, ongoing research is vital for improving efficiency and reducing costs.
As we synthesize information presented throughout this article, it is critical to recognize hydrogen's role in a larger energy ecosystem. Plans for future hydrogen economies should reflect a balance between ambition and practicality.
"The exploration of hydrogen as an energy source represents not just an opportunity but an imperative toward a sustainable future."