Innovative Applications of Functionalized Nanoparticles
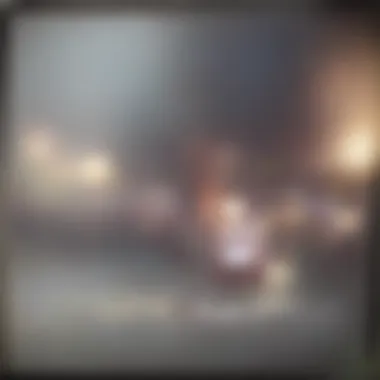
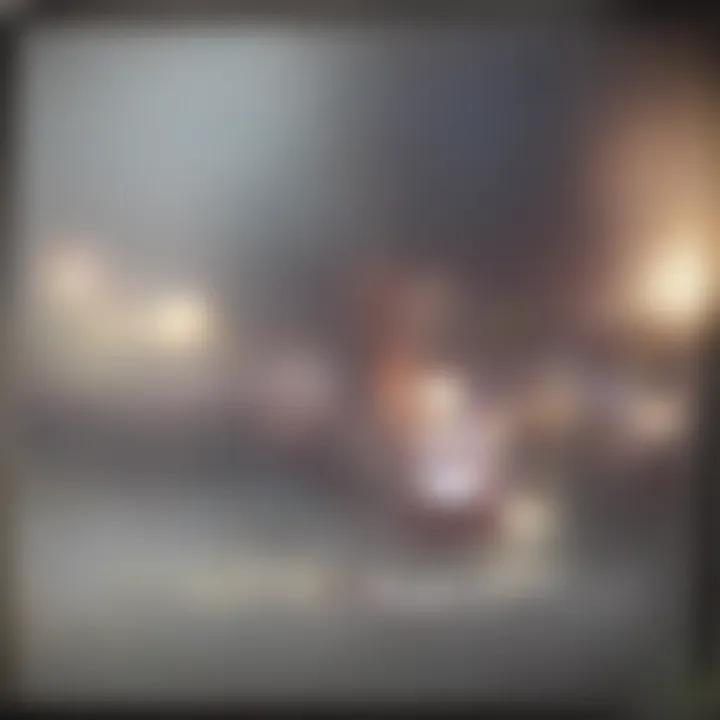
Intro
Functionalized nanoparticles represent a fascinating convergence of science and technology. Their ability to be tailored for specific applications makes them invaluable in many fields. These tiny particles, typically in the range of 1 to 100 nanometers, can be designed to interact with biological and environmental systems in unique ways.
Their small size allows for extensive surface area, which enhances reactivity and interaction. As such, the understanding of their synthesis and surface modification is critical for researchers and practitioners alike. The potential of these nanoparticles spans from medicine to environmental solutions, further necessitating a comprehensive discourse on their innovative applications and methodologies.
This article will delve deep into the key findings surrounding these nanoparticles to illuminate their impact across various scientific domains.
Intro to Functionalized Nanoparticles
The topic of functionalized nanoparticles holds critical importance in various fields such as biomedicine, environmental science, and material engineering. These nanoparticles serve as a bridge between chemistry and advanced applications, providing unique properties that enhance performance in numerous systems. Their synthesis and modification open new frontiers, allowing researchers to manipulate size, shape, and surface characteristics to tailor-make properties for specific uses.
Functionalized nanoparticles can improve the efficacy of drug delivery systems, enable precise diagnostics, and enhance the mechanical, thermal, and electrical properties of materials. Their ability to interact at the molecular level offers exceptional opportunities to address challenges inherent in modern technology and healthcare. This uniqueness makes them a focal point in ongoing research and development, invigorating scientific dialogue around their potential and the methodologies involved in their creation.
"Functionalized nanoparticles represent a next-generation technology that merges material science with innovative medicinal applications."
Definition and Characteristics
Functionalized nanoparticles refer to nanometer-sized particles that have been modified to exhibit specific properties through a surface modification process. This process gives these nanoparticles enhanced stability, dispersibility, and functionality. Functionality is often achieved by attaching ligands, polymers, or other chemical groups to the surface. This tailored surface chemistry allows for selective targeting in drug delivery applications or enhanced adsorption abilities in environmental remediation efforts.
Characteristics of functionalized nanoparticles can include:
- Size: Typically in the range of 1 to 100 nanometers, providing a high surface area to volume ratio.
- Shape: Can vary from spherical to rod-like or even complex geometries, influencing their behavior in applications.
- Surface Charge: A key factor in interaction with biological systems, affecting cellular uptake and toxicity.
- Composition: Various materials can be utilized, including metals, oxides, and polymers, to yield desired properties.
Historical Context
The development of functionalized nanoparticles can be traced back to the advent of nanotechnology in the late 20th century. Initial research focused on the basic properties of nanoparticles. Scientists began to recognize the potential for modifying the surface of particles to enhance their performance for specific applications. For example, the work on gold nanoparticles in the early 2000s demonstrated their utility in biological imaging and drug delivery.
Since then, significant progress has been made in various synthesis methods, expanding the library of available nanoparticles and their functionalities. Many important studies and innovations have contributed to the evolution of this field, with ongoing research by institutions worldwide. Technological advancements have allowed the integration of functionalized nanoparticles into commercial products, showcasing their importance not only in research but also in practical applications. Retrieval of knowledge from literature has become a cornerstone in shaping the future of functionalized nanoparticles.
Synthesis of Functionalized Nanoparticles
The synthesis of functionalized nanoparticles is crucial for developing innovative materials that exhibit unique properties for various applications. It establishes the foundation for achieving desired characteristics like size, shape, and surface chemistry, which dictate how these particles will interact in different environments. This section delves into the various methodologies employed in the synthesis of these nanoparticles, highlighting their advantages and considerations. The selection of an appropriate synthesis method can significantly influence the effectiveness of the functionalization process and, in turn, the nanoparticle's performance in practical applications.
Chemical Methods
Chemical methods are widely used for producing functionalized nanoparticles due to their ability to offer precise control over size and composition. One common approach is sol-gel synthesis, which involves the transition of a solution into a solid gel phase. This method provides high homogeneity and tunable properties by adjusting the precursor compounds and reaction conditions.
Another important technique is chemical vapor deposition (CVD). In CVD, precursor gases react at elevated temperatures to form a solid material on a substrate. The benefits of CVD include high purity and the ability to produce thin films with excellent uniformity.
However, it is essential to consider factors such as reaction temperature, pressure, and the choice of solvents during the chemical synthesis process. By optimizing these parameters, researchers can create nanoparticles that meet the specific requirements for applications in biomedicine, environmental remediation, and materials science.
Physical Methods
Physical methods for synthesizing functionalized nanoparticles often involve non-chemical processes, focusing on physical principles. A popular method is laser ablation, where a high-energy laser is directed at a target material, producing nanoparticles by vaporization and subsequent condensation in a gas or liquid medium. This technique allows for precise control over particle size and morphology, contributing to its adoption in research settings.
Another notable approach is ball milling, which mechanically grinds bulk materials into nanoparticles. This method is applicable for a variety of materials and is advantageous due to its simplicity and scalability. However, care must be taken to minimize contamination and ensure uniform particle distribution.
Thanks to their distinctive benefits, these physical methods suit various applications in electronics and catalysis, emphasizing the versatility of functionalized nanoparticles in technology.
Biological Methods
Biological methods present an eco-friendly alternative for synthesizing functionalized nanoparticles. These methods typically utilize biological entities such as microorganisms, plants, or biopolymers to produce nanoparticles through bioreduction or biosorption. For example, certain fungi and bacteria can convert metal ions into nanoparticles, thanks to their inherent metabolic processes.
Using biological methods often results in nanoparticles with unique characteristics, such as biocompatibility and reduced toxicity. One noteworthy example is the green synthesis of gold nanoparticles using plant extracts, which not only simplifies the synthesis process but also imparts added biological attributes to the nanoparticles.
However, the variability in biological reactions can pose challenges related to reproducibility and scalability. Despite these challenges, the growing emphasis on environmentally benign processes positions biological synthesis as a significant avenue for future research in functionalized nanoparticles.
"The synthesis techniques are integral to effectively harnessing the potential of functionalized nanoparticles across diverse fields, from medicine to environmental science."
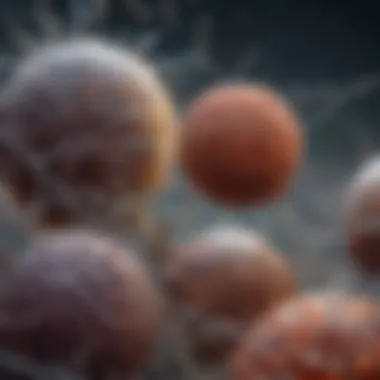
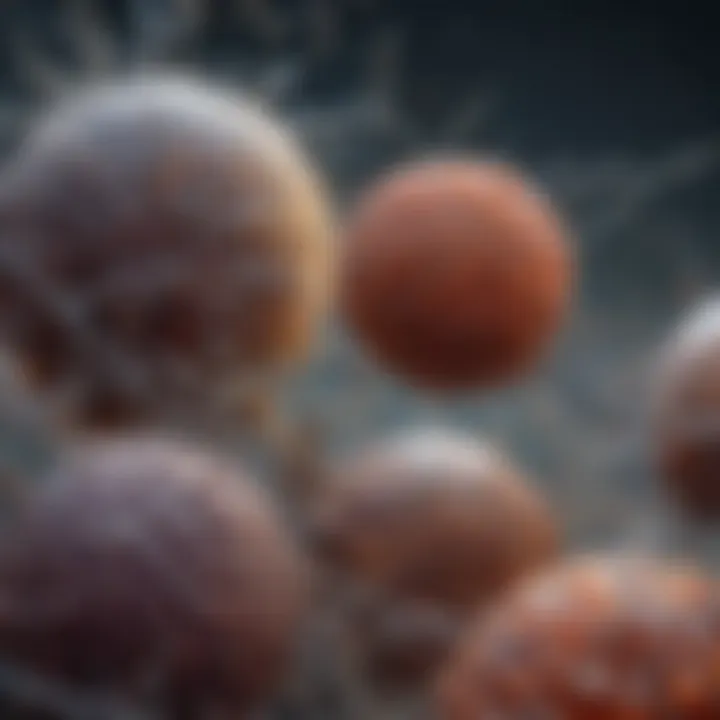
This section serves as an essential primer on the synthesis of functionalized nanoparticles, laying the foundation for understanding their subsequent applications in various scientific realms.
Surface Modification Techniques
Surface modification of nanoparticles is crucial for enhancing their functionality and application potential. This process adjusts the surface properties of nanoparticles, impacting their interaction with other substances in various environments. The modifications can lead to improved stability, biocompatibility, and target selectivity, significantly increasing their effectiveness in diverse fields like biomedicine, environmental remediation, and material science.
The importance of surface modification techniques lies in their ability to:
- Tailor chemical properties: By altering the surface chemistry, nanoparticles can better interact with biological systems, enabling more effective drug delivery systems or diagnostic imaging agents.
- Enhance stability: Modifications can protect nanoparticles from agglomeration or degradation in harsh environments, prolonging their functional lifespan.
- Facilitate functionalization: Surface modifications allow for the attachment of specific molecules or ligands, which can guide nanoparticles to specific sites or improve their interaction with target molecules.
This section will focus on three primary surface modification techniques: ligand exchange, polymer coating, and self-assembly approaches.
Ligand Exchange
Ligand exchange is a commonly employed technique involving the replacement of surface ligands on nanoparticles with new ligands that provide desired properties. This method allows for the customization of the nanoparticle's surface chemistry and can significantly alter its behavior in solution.
Some benefits of ligand exchange include:
- Improved targeting capabilities, which allow for more precise delivery of drugs.
- Enhanced biocompatibility, making nanoparticles safer for biomedical applications.
- Modification of optical properties, enabling their use in imaging applications.
Moreover, the choice of ligands can affect the overall effectiveness of the nanoparticles. Therefore, careful selection of ligands is essential for achieving specific outcomes in various applications.
Polymer Coating
Polymer coating involves the application of polymeric materials onto the surface of nanoparticles to modify their properties. This method is advantageous because it can encapsulate nanoparticles within a polymer matrix, enhancing their stability and protecting them from environmental factors.
Key advantages of polymer coatings include:
- Increased dispersibility in biological environments, improving interactions with cells or tissues.
- The ability to control the rate of drug release, enabling sustained therapeutic effects.
- Lower toxicity profiles, as polymers can shield active agents from causing harm to healthy cells.
Different polymers can be used, with choices depending on the intended application, thus making polymer coating a versatile approach in nanoparticle modification.
Self-Assembly Approaches
Self-assembly approaches utilize the spontaneous organization of molecules into structured arrangements on the nanoparticle surface. This technique is driven by various intermolecular forces, allowing nanoparticles to achieve specific architectures without external guidance.
The advantages of self-assembly include:
- Enhanced stability and integrity of the nanoparticle formulation.
- The creation of highly organized structures that can improve functionality in applications such as sensors or drug delivery.
- The potential for large-scale production with uniform properties, which is ideal for commercialization.
Self-assembly can lead to innovative designs that improve the performance of nanoparticles in their applications. This method stands at the intersection of chemistry, physics, and materials science, making it a highly interdisciplinary and promising technique.
In summary, surface modification techniques are vital for enhancing the utility of functionalized nanoparticles across diverse applications, from biomedicine to environmental science. Their ability to tailor properties and improve performance makes them a focal point of ongoing research.
Biomedical Applications
Biomedical applications of functionalized nanoparticles represent a significant area of research and innovation, offering novel solutions to complex medical challenges. The relevance of these applications cannot be overstated, as they bridge gaps between science and real-world needs in healthcare. From targeted drug delivery to enhanced imaging techniques, functionalized nanoparticles are transforming how we approach treatment and diagnostics in medicine.
Drug Delivery Systems
One of the most promising uses of functionalized nanoparticles is in drug delivery systems. Traditional drug delivery methods often face obstacles such as low bioavailability and systemic side effects. Functionalized nanoparticles, however, provide a means to improve drug solubility and stability, allowing drugs to be more effectively targeted to specific tissues or cells.
By modifying the surface of these nanoparticles with specific ligands, it becomes possible to enhance the interaction with targeted cells. This selectivity is crucial in minimizing side effects and maximizing therapeutic efficacy. For instance, drugs can be delivered directly to cancer cells, thereby sparing healthy cells from harmful side effects associated with conventional treatments. Research shows that systems utilizing functionalized nanoparticles for drug delivery can increase the concentration of drugs in the desired area while reducing overall dosage requirements.
Imaging and Diagnostics
Functionalized nanoparticles also play a critical role in imaging and diagnostics, significantly advancing techniques such as magnetic resonance imaging (MRI) and computed tomography (CT). Diagnostics therapies benefit from the ability of nanoparticles to improve the contrast and resolution of imaging techniques.
Gold nanoparticles and quantum dots are widely used in imaging because they can be easily modified and provide unique optical properties. They can be entwined with specific biomarkers, allowing for the visualization of cellular processes in vivo. This enables early diagnosis and better monitoring of diseases, particularly cancers.
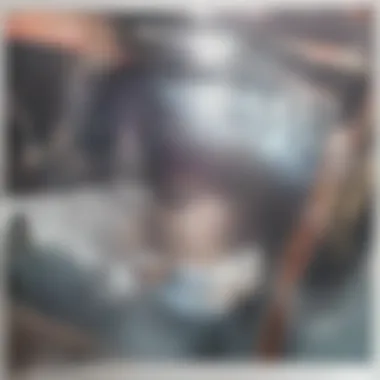
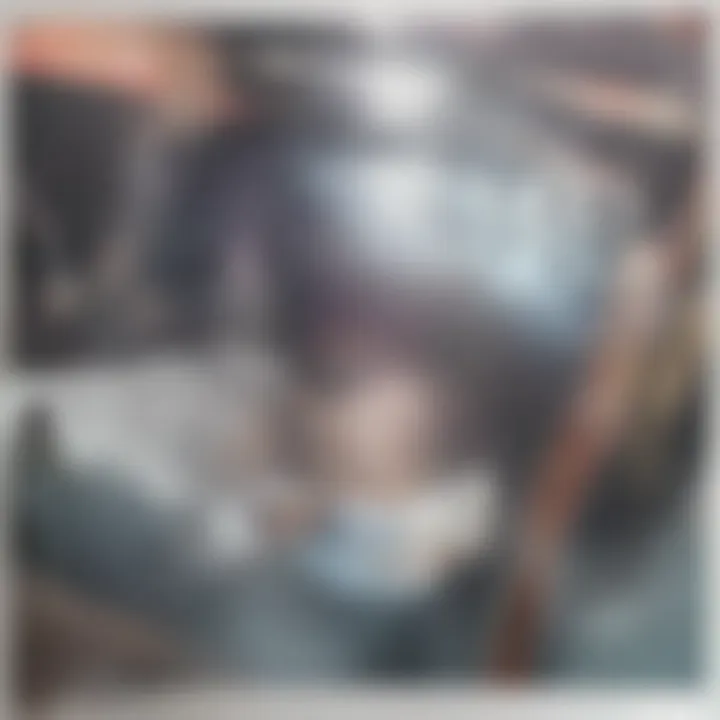
Furthermore, the capability of these nanoparticles to herald a metabolic change or disease progression can vastly enhance the understanding of patient conditions, leading to more informed decision-making in treatment.
Cancer Therapeutics
Cancer therapeutics is another key area where functionalized nanoparticles show exceptional promise. With cancer being a complex disease, it requires innovative approaches for treatment. Nanoparticles offer a platform for developing targeted therapy, reducing the reliance on conventional methods that often yield poor outcomes due to non-specific targeting.
Functionalized nanoparticles can be used to encapsulate cytotoxic agents and release them at the tumor site. This targeted release substantially enhances the local concentration of the drug at cancer cells while decreasing exposure to healthy tissues. Some recent studies have highlighted the effectiveness of using liposomal formulations of doxorubicin, a common chemotherapeutic drug, where doxorubicin is encapsulated in liposomes made from functionalized nanoparticles.
The potential advantages of using nanoparticles in cancer therapeutics also include the ability to overcome drug resistance, as they can bypass mechanisms that normally prevent effective treatment.
"Functionalized nanoparticles can potentially redefine cancer treatment paradigms by facilitating precise drug delivery while minimizing systemic toxicity."
Environmental Remediation
Environmental remediation is a critical aspect in the context of functionalized nanoparticles. As our planet faces increasing pollution from industrial processes, agricultural runoff, and urban development, the need for effective remediation techniques becomes more urgent. The use of functionalized nanoparticles offers unique advantages that traditional remediation methods cannot achieve.
Functionalized nanoparticles can be engineered at the nanoscale to possess specific properties that enhance their ability to remove contaminants from the environment. The versatility of these materials makes them suitable for various applications, including water purification, soil decontamination, and air quality improvement. Their small size provides a high surface area-to-volume ratio, which is essential for efficient adsorption of pollutants.
Benefits of Functionalized Nanoparticles in Environmental Remediation:
- Targeted Contaminant Removal: Tailoring the surface of nanoparticles allows for the selective targeting of specific pollutants. This means that harmful substances can be absorbed while leaving essential nutrients untouched.
- Enhanced Reactivity: Functionalized nanoparticles can be designed to react with pollutants chemically, breaking them down into less harmful substances more efficiently.
- Cost-Effectiveness: The efficiency of nanoparticles can reduce the overall cost of remediation processes, making it a more accessible solution for various applications.
However, the integration of functionalized nanoparticles in environmental remediation also raises considerations regarding their potential impact on ecosystems. Issues such as their long-term stability, possible toxicity, and accumulation in the environment warrant thorough research. Addressing these factors is crucial to ensuring that the benefits outweigh any risks associated with their use.
Pollutant Adsorption
Pollutant adsorption is a primary function of functionalized nanoparticles in environmental remediation. The mechanism involves the adherence of pollutants onto the surface of nanoparticles, effectively removing these harmful substances from the environment. This process benefits from the nanoparticlesโ high surface area, which allows for a greater interaction with pollutants.
The choice of functionalization plays a significant role in enhancing adsorption capabilities. For instance, modifying the surface with specific chemical groups can create strong affinities for various pollutants, including heavy metals, organic compounds, and dyes. This tailored approach improves the overall efficiency and selectivity of the adsorption process.
Furthermore, pollutant adsorption can be influenced by environmental factors such as pH, temperature, and the presence of other chemicals. Researchers continually study these variables to optimize the use of functionalized nanoparticles in adsorption-based remediation strategies. This ensures that practical applications in real-world environments are effective and sustainable.
Nanoparticle-Enhanced Filtration
Nanoparticle-enhanced filtration represents another innovative application in environmental remediation. This technique involves using functionalized nanoparticles as filtration media to remove contaminants from water and air. The incorporation of nanoparticles within traditional filtration systems enhances their performance dramatically.
By embedding nanoparticles in filtration membranes, it is possible to achieve finer filtration, allowing for the removal of even the smallest particulates and dissolved contaminants. Notably, certain nanoparticles can also provide additional functionalities, such as photocatalytic activity, enabling the breakdown of organic pollutants under light exposure.
The integration of functionalized nanoparticles into existing filtration systems can significantly improve the efficiency and effectiveness of water and air purification processes. Studies have shown that these enhanced systems can reduce pollutant levels to very low concentrations, resulting in cleaner drinking water and improved air quality.
Nanoparticles in Material Science
The incorporation of nanoparticles in material science has led to significant advances across various applications. Functionalized nanoparticles have unique properties that contribute to the development of innovative materials with enhanced functionalities. Researchers are particularly interested in this domain due to the ability of nanoparticles to impact physical, chemical, and biological behavior of materials. The micro-structural design afforded by these nanoparticles opens pathways for new applications in electronics, optics, and energy production.
The use of nanoparticles often results in improvements in strength, durability, and responsiveness of materials. Moreover, these enhancements can lead to lighter materials without compromising quality, which is pivotal in industries like aerospace and automotive. Key considerations around these materials include their stability, environmental impact, and effective integration into larger systems.
Smart Materials Development
Smart materials, comprising functionalized nanoparticles, are designed to adapt to external stimuli such as temperature, pressure, or electric fields. The nanoparticles enable these materials to 'respond' dynamically to changing conditions. This responsiveness can improve safety and efficiency in several sectors, including construction and consumer electronics.
For instance, thermochromic materials change color in response to temperature changes, useful in applications ranging from safety indicators to artistic displays. In sensors, nanoparticles augment sensitivity and performance. This technology promises to impact smart textiles, responsive coatings, and adaptive architecture profoundly.
Nanocomposites
Nanocomposites represent a significant focus of material science. By combining traditional materials with nanoparticles, researchers create composites with superior properties. For instance, adding silica nanoparticles to polymers can enhance tensile strength and improve thermal stability. This composite approach enables the creation of lightweight yet durable materials applicable in various industries.
When formulating nanocomposites, the geometry and dispersion of nanoparticles play crucial roles. Uneven distribution or improper integration can affect material properties negatively. Therefore, precise synthesis and processing methods are vital. The ability to control these factors allows for customization of mechanical and thermal properties, paving the way for highly specialized materials tailored for specific functions.
The integration of functionalized nanoparticles into material science is not merely an augmentation of existing technologies but a pathway to entirely new solutions in engineering and design.
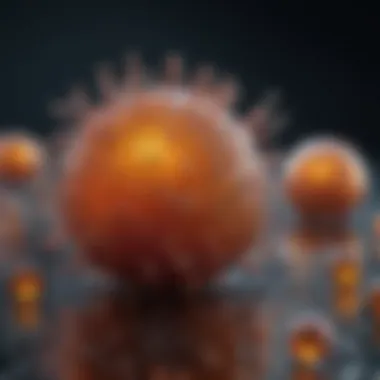
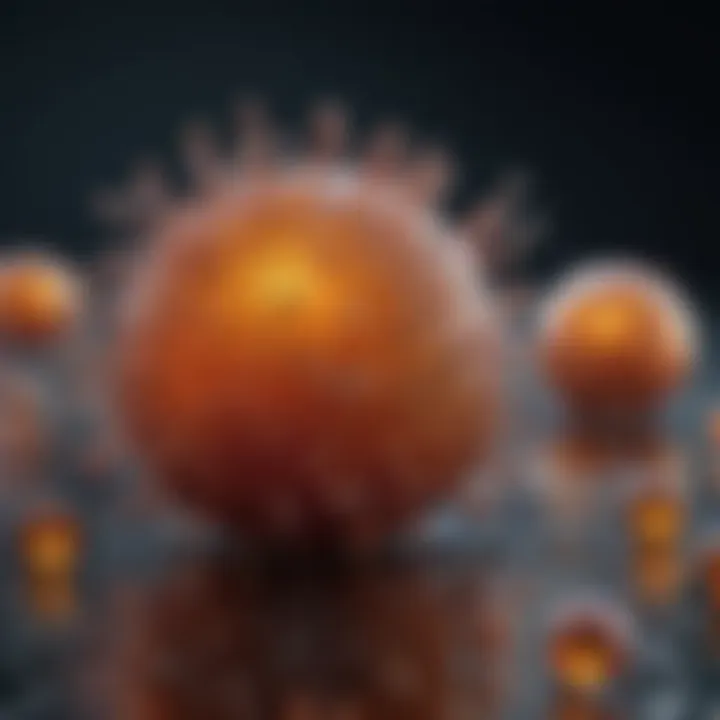
Through careful research and innovative approaches, the potential applications of nanoparticles in material science continue to expand, driving forward the boundaries of what is possible in this evolving field.
Challenges in Research and Development
The field of functionalized nanoparticles holds immense promise but is not without its significant challenges. These challenges can hinder progress and development in various applications. Understanding these issues is crucial for researchers and practitioners in the field, as they have direct implications for the future of functionalized nanoparticle technology. Some key areas of concern include regulatory issues, stability, and toxicity.
Regulatory Issues
Regulatory challenges are one of the main roadblocks in the application of functionalized nanoparticles. Different countries have diverse regulations regarding the use of nanoparticles in products and processes. These regulations need to address safety, efficacy, and environmental impact.
For instance, in the United States, the Environmental Protection Agency (EPA) and the Food and Drug Administration (FDA) play critical roles in overseeing the introduction of nanoparticle-based formulations. Regulations often lag behind scientific developments, leading to uncertainty in the market. This situation can stifle innovation, as businesses may hesitate to invest in new technologies that lack clear regulatory pathways. Navigating these complex regulatory landscapes is essential for successful deployment.
Stability and Toxicity
Another pressing challenge relates to the stability and toxicity of functionalized nanoparticles. Stability refers to the ability of nanoparticles to maintain their properties and effectiveness over time. Factors like environmental conditions and formulation specifics can impact stability, which is vital when considering the product's shelf life and performance.
Toxicity is also a major concern. Researchers are still trying to understand the potential health risks posed by these nanoparticles to human health and the environment. Studies have indicated that nanoparticles can exhibit unique toxicological profiles compared to their bulk counterparts. Such differences necessitate comprehensive testing to determine the safety and biocompatibility of these materials.
"Safety testing and standards must evolve alongside technological advancements in nanoparticle research to ensure that innovative applications do not come at the cost of public health."
In summary, regulatory issues and concerns regarding stability and toxicity are critical challenges that need to be addressed in the field of functionalized nanoparticles. Addressing these challenges effectively is essential for advancing research and applications, ultimately unlocking the full potential of these remarkable materials.
Future Directions in Functionalized Nanoparticle Research
The field of functionalized nanoparticles is constantly evolving. Research and development focus on various innovative directions that aim to enhance their applications and efficiency. This section examines crucial elements and benefits shaping the future of this field.
Understanding these future directions is essential as they will define the trajectory of functionalized nanoparticles in solving pressing scientific challenges. Exploring innovative applications in healthcare, environment, and material sciences can lead to significant breakthroughs in each discipline. In particular, the integration of advanced technologies will enhance the efficiency and effectiveness of nanoparticles.
Emerging Trends
Recent trends indicate a strong shift towards highly specialized applications of functionalized nanoparticles. This includes customization of their surface properties to meet specific needs in various fields. Some notable trends are:
- Personalized Medicine: Tailored drug delivery systems can improve therapeutic outcomes and reduce side effects.
- Sustainable Development: Innovations aim at reducing waste during synthesis and enhancing recyclability, addressing environmental concerns.
- Nanomedicine: A growing focus on using nanoparticles for imaging and targeting specific sites in the body, enhancing diagnostic accuracy.
Each of these trends highlights not only technological advances but also societal needs driving the research.
"Future research will likely incorporate advanced computational tools to better understand and predict the behavior of nanoparticles in various environments."
Interdisciplinary Approaches
An interdisciplinary approach is becoming increasingly important in functionalized nanoparticle research. Collaboration between different scientific fields can lead to more comprehensive solutions. Key areas of interest include:
- Chemistry and Biology: Interactions at the molecular level are vital for designing effective nanoparticles that interact well with biological systems.
- Physics and Materials Science: Understanding the physical properties of nanoparticles can facilitate their integration into new materials, enhancing functionalities such as strength and conductivity.
- Engineering and Environmental Science: Developing systems for pollution control and remediation using nanoparticles requires a blend of engineering practices and environmental assessments.
By embracing these interdisciplinary strategies, researchers can pave the way for innovative applications in functionalized nanoparticles, leading to advancements that can significantly impact health, technology, and the environment.
Finale
The exploration of functionalized nanoparticles has emerged as a crucial area of research in various fields, notably in biomedicine, environmental science, and material engineering. This article has detailed the synthesis methods, surface modification techniques, and innovative applications of these nanoparticles. Importantly, the discussion emphasizes how tailored properties of functionalized nanoparticles can directly influence their effectiveness in real-world applications.
In summary, three key developments stand out:
- Advancements in Synthesis: The continuous evolution of methods used to synthesize functionalized nanoparticles has enabled researchers to create more efficient and targeted solutions.
- Diverse Applications: Their applications are sprawling, ranging from drug delivery systems to advanced materials that enhance efficiency in various technologies.
- Interdisciplinary Collaboration: The future potential of functionalized nanoparticles will largely depend on interdisciplinary approaches, integrating knowledge from various scientific domains.
Summary of Key Developments
The significant advancements in the field of functionalized nanoparticles have unlocked new possibilities.
- Synthesis Techniques: Modern techniques have made it easier to customize nanoparticle characteristics. Methods like chemical vapor deposition and biosynthesis are leading the way, offering both reliability and environmental sustainability.
- Functionality and Performance: Enhanced surface modification techniques, such as ligand exchange and polymer coating, have significantly improved performance across applications, making nanoparticles more suitable for targeted use.
- Regulatory Compliance: Increasing awareness regarding safety and regulatory measures is essential. Researchers are focusing on balancing innovation with compliance, ensuring nanoparticles are safe for use in various environments.
"The versatility of functionalized nanoparticles is not just a boon for research; it is reshaping industries and providing solutions to global challenges," notes an expert in the field.
The Road Ahead
As the field evolves, several critical paths emerge for future research:
- Sustained Research Focus: Continuous research is needed to address challenges such as stability and toxicity of nanoparticles, ensuring their practical and safe application.
- Real-World Applications: The translation of laboratory successes into industrial applications should be prioritized. Researchers aim to develop nanoparticles that can perform reliably in unpredictable environments.
- Global Collaboration: The future may hold greater collaboration across geographic and academic boundaries, fostering a rich exchange of ideas and innovations.