Exploring the Measurement of Brainwaves in Neuroscience
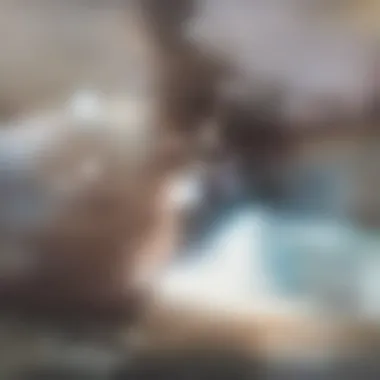
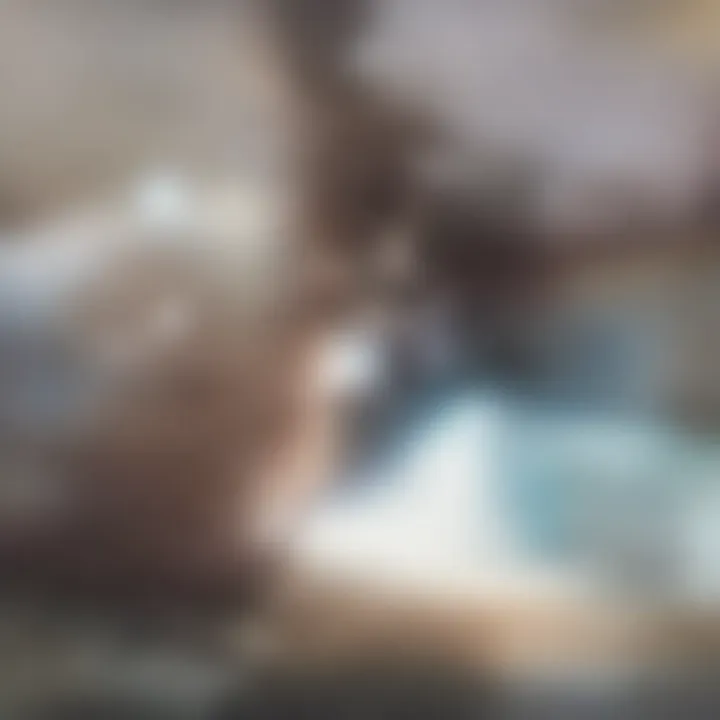
Intro
The study of brainwaves is a cornerstone of neuroscience and psychology, with various implications across healthcare, cognitive research, and artificial intelligence. The measurement of brainwaves reveals essential insights into cognitive states and processes. Understanding how we measure these brainwaves allows one to grasp the fundamental principles that govern human thought and behavior.
As technology advances, the methodologies involved in brainwave measurement have evolved significantly. The historical backdrop of this field is as fascinating as the science itself. It combines ancient philosophies with modern computational analyses, revealing how far we have come and paving the way for future innovations in neurotechnology.
Key Findings
Major Results
Research indicates that brainwaves can be categorized based on their frequency ranges, each associated with specific mental states. Delta waves, for instance, are linked to deep sleep, while beta waves correlate with active thinking and problem-solving. Recognizing these patterns empowers researchers to interpret brain activity effectively.
Furthermore, advancements in typical techniques, such as Electroencephalography (EEG), have improved our ability to assess brain activities accurately. Researchers have leveraged these technologies for applications in treating neurological disorders, enhancing cognitive functions, and even developing brain-computer interfaces.
Discussion of Findings
Consequently, the implications of these findings stretch beyond mere academic interest. The insights gained can lead to impactful developments in various fields. For example, companies like Emotiv are creating devices that monitor emotional states using brainwave data. This kind of advancement has the potential to revolutionize mental health interventions and user experience design.
"Understanding brainwave measurements can pave the way for groundbreaking innovations in various sectors, from healthcare to gaming and artificial intelligence."
Methodology
Research Design
The methodologies employed in measuring brainwaves incorporate both quantitative and qualitative designs. Utilizing EEG, researchers can record the electrical activity in the brain, which serves as a reliable primary source for understanding cognitive functions. Complementary approaches, such as fMRI and PET scans, offer additional layers of data, revealing more comprehensive pictures of brain activity.
Data Collection Methods
The data collection methods are critical to ensuring validity and reliability in results.
- Electroencephalography (EEG): This method uses electrodes placed on the scalp to measure electrical activity. It is both non-invasive and widely accessible.
- Functional Magnetic Resonance Imaging (fMRI): This imaging technique maps brain activity through changing blood flow, offering insights into brain functions.
- Magnetoencephalography (MEG): Measures magnetic fields produced by neural activity, providing a high temporal resolution.
Each method has its strengths and weaknesses, thus understanding the nuances of these approaches is crucial for interpreting brainwave data accurately.
As we further explore the advancements in brainwave measurement technologies, we encounter more complex ethical considerations. This plays a vital role in shaping the future landscape of neuroscience.
Prelude to Brainwaves
The study of brainwaves offers insights into the functioning of the human brain. Understanding brainwaves is crucial as it directly ties to how we interact with the world, both consciously and subconsciously. This section lays the groundwork for comprehending the more intricate aspects of brainwave measurement. The significance of this topic extends to various fields, including psychology, neuroscience, and even artificial intelligence.
Definition of Brainwaves
Brainwaves are electrical impulses in the brain, produced when neurons communicate with one another. They reflect the brain's activity levels and are classified based on their frequency. These frequencies are measured in hertz (Hz) and range from very slow to fast waves. Brainwaves play a significant role in determining our mental state, influencing behavior, cognitive processes, and overall mental health.
Types of Brainwaves
The exploration of different types of brainwaves is essential for understanding how brain activity varies under different conditions. Each type of brainwave has unique characteristics and functions.
Delta Waves
Delta waves are the slowest brainwaves, with a frequency of 0.5 to 4 Hz. They are most prominent during deep sleep and are crucial for restorative sleep phases. These waves are associated with healing and regeneration. Their primary contribution is the facilitation of bodily recovery while the brain is at rest.
"Delta waves are essential to support various physiological processes during sleep, indicating their importance in healthy functioning."
Theta Waves
Theta waves range from 4 to 8 Hz and are commonly linked to light sleep, relaxation, and creativity. They can be prominent during meditation or daydreaming. Theta waves play a critical role in memory processing and emotional regulation. Their unique feature often involves deep insights and intuition, making them valuable in therapeutic contexts. However, high levels of theta activity can also influence attention negatively.
Alpha Waves
Alpha waves have a frequency of 8 to 12 Hz and are typically present during states of calm alertness or meditation. They signify a bridge between conscious thinking and deep relaxation. Their presence is often a sign of readiness and receptivity, providing benefits in learning and creativity. Yet, excessive alpha activity may indicate disengagement from the external environment.
Beta Waves
Beta waves range from 12 to 30 Hz. They dominate our normal waking state, being associated with active thinking, decision-making, and problem-solving. The presence of beta waves is beneficial for productive activities, as they enhance focus and cognitive alertness. However, increased beta activity can lead to stress or anxiety, indicating a delicate balance.
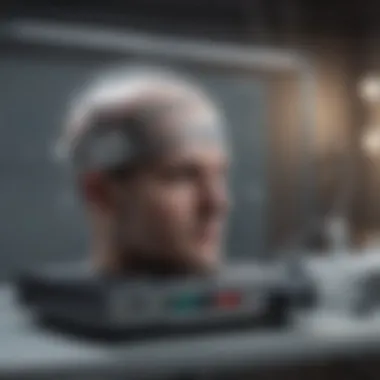
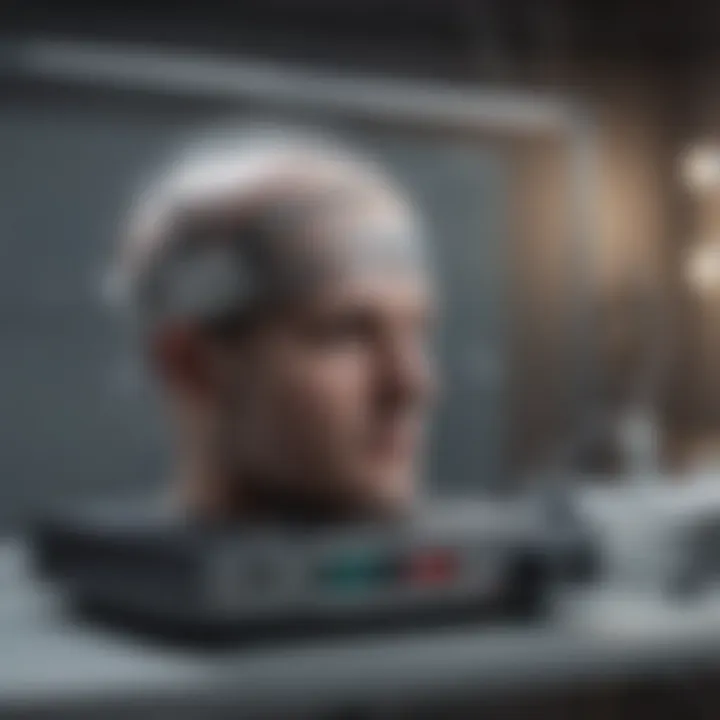
Gamma Waves
Gamma waves operate at frequencies above 30 Hz, and they are associated with higher-order cognitive functions such as problem-solving, memory retrieval, and information processing. Their presence is vital during intense mental activity and learning tasks. Gamma waves are considered beneficial for cognitive enhancement; however, they also require substantial mental energy and can lead to fatigue when overly accessed.
Historical Context of Brainwave Measurement
Understanding the historical context of brainwave measurement provides essential insights into the evolution of neuroscientific methods. This section will illuminate how early research laid the groundwork for modern practices, emphasizing the significance of past discoveries and technological advancements. Exploring this timeline helps us appreciate current methodologies, evolving insights, and the implications of brainwave research.
Early Discoveries
The journey began in the late 19th century. The first significant break through came from Hans Berger, a German psychiatrist, who is credited with the discovery of brainwaves in 1924. His pioneering work lead to the recording of electrical activity from the human brain using a technique he called electroencephalography, or EEG. This development was revolutionary, revealing that the brain's electrical signals change with different states of consciousness, such as sleep or wakefulness.
Before Berger, scholars were aware of the brain's role in behavior but lacked tools to study its electrical activity in real-time. Early discoveries, though rudimentary, pointed to the idea that mental states could be linked to measurable electric signals.
These insights raised various questions about the interpretative aspects of brain activity. As researchers began to correlate specific wave patterns with certain cognitive functions or emotional states, it became evident that brainwaves are not only vital to understanding brain health but also serve an important role in developing treatments for neurological conditions.
Development of EEG Technology
The advancement of EEG technology progressed significantly in the decades following Berger's seminal work. Initially, EEG was a labor-intensive process. It involved attaching multiple electrodes to the scalp and interpreting the output manually. Over time, refinement and miniaturization of electrodes made the process less cumbersome.
In the 1940s and 1950s, progress was made in amplifying brain signals and filtering noise. Researchers, utilizing analog devices, improved the quality and clarity of the recorded brainwaves. The introduction of computer technology in the latter half of the 20th century revolutionized EEG further. Words like 'waveforms' and 'spectral analysis' became common as digital technologies enabled detailed analysis of brain activity patterns.
This period also saw significant collaboration across disciplines. Neuroscience, psychology, and engineering converged to innovate methods for brainwave measurement. The adaptation of EEG to monitor various states such as sleep stages, seizures, and even cognitive load has become a standard practice in both clinical and research settings today.
The early instances of EEG measurements catalyzed an expansive field of research, paving the way for numerous applications. Researchers began exploring its capabilities in various fields like psychology, neurology, and even marketing.
"Electroencephalography has transformed from a simple recording technique into a powerful tool for understanding complex neural dynamics."
The history of brainwave measurement thus illustrates continuous improvement and adaptation. Each technological leap has allowed scientists to probe deeper into the mysteries of the human brain, offering better insights and applications of the knowledge gained.
Scientific Principles of Brainwave Measurement
The measurement of brainwaves is underpinned by fundamental scientific principles that govern how we understand and interpret these electrical activities. Understanding these principles provides clarity on the mechanisms that drive brainwave activity, yielding insights applicable in various fields. Such knowledge is essential not only for enhancing diagnostic capabilities in clinical settings but also for advancing research in cognitive science.
Neurophysiology Basics
Neurophysiology explores the functions of the nervous system, particularly focusing on neurons. Neurons are specialized cells responsible for transmitting information throughout the brain and body. Each neuron communicates through electrical impulses, which are crucial for brainwave formation. These impulses lead to the generation of brainwaves that can be captured and analyzed.
In essence, brainwaves originate from synchronized electrical activity of groups of neurons firing together. Different frequencies denote various states of mind—from deep sleep to intense focus. By examining these brainwave patterns, researchers can infer a wide range of cognitive and emotional states.
Brainwaves are categorized into five main types: Delta, Theta, Alpha, Beta, and Gamma, each with its own implications. For instance, Delta waves indicate deep sleep, whereas Beta waves are linked to active thinking and concentration. Knowing how these brainwave types relate to our mental states allows for better comprehension of various psychological and neurological conditions.
Electrical Activity of Neurons
The electrical activity of neurons is a complex process defined by both the resting potential and action potentials.
- Resting Potential: When neurons are inactive, there exists a voltage difference across their membranes due to an unequal distribution of ions. This voltage is termed the resting potential, typically around -70 mV. It reflects the neuron's readiness to fire.
- Action Potentials: When a neuron receives adequate stimulus, it becomes depolarized. This change in voltage initiates an action potential, where the membrane potential rapidly rises and falls. This wave of depolarization propagates along the neuron, ultimately leading to neurotransmitter release at a synapse.
The collective action potentials from thousands of neurons create fields of electrical activity detectable by sensitive electrodes during EEG. Such measurements represent a snapshot of brain activity at any given time.
Understanding the solid foundations of neurophysiology and neuronal electrical activity equips researchers to make informed decisions about how to utilize brainwave data effectively. As brainwave measurement technologies evolve, insights derived from these scientific principles will unlock new applications in health, education, and personal development.
Advances in technology, combined with understanding of neurophysiology, bring possibilities for better health outcomes.
Exploring these layers teaches about the intricate relationships between neuronal operation and psychological phenomena. Whether for academic research or clinical application, grasping the scientific underpinnings of brainwave measurement is notably valuable.
Electroencephalography (EEG) Methodology
Electroencephalography, commonly known as EEG, is a critical part of understanding brainwave measurement. This methodology enables researchers to capture the electrical activity of the brain in both clinical and research settings. The ability to monitor brainwaves in real-time provides unique insights into cognitive functions, sleep patterns, and various neurologic conditions.
The effectiveness of EEG as a measurement tool lies in its relatively simple setup and non-invasive nature. Patients and subjects can go about their activities or rest comfortably while their brain activity is recorded. However, this method does come with its set of challenges and considerations. Careful planning of electrode placement, signal processing, and final data interpretation are crucial for acquiring accurate results.
Electrode Configuration
The electrode configuration in EEG is vital for capturing the brain's electrical activity effectively. Typically, electrodes are placed on the scalp according to the international 10-20 system. This standardization helps in maintaining consistency across studies and applications.
Each electrode detects the electrical signals produced by underlying neurons. It is essential to consider factors such as:
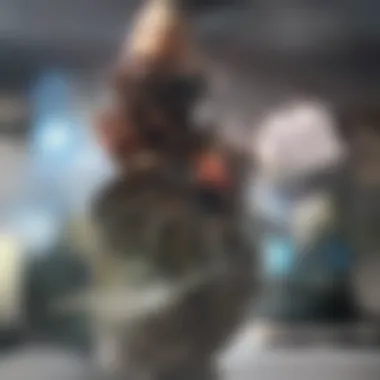
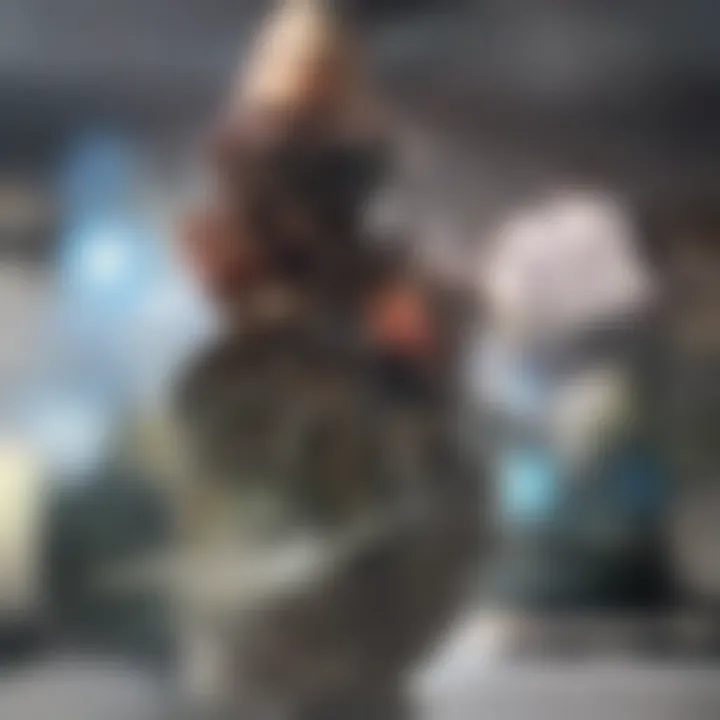
- Electrode Type: Different types of electrodes—such as silver/silver chloride or gold—vary in their conductivity and ease of use.
- Placement: Correct positioning according to brain regions aligns the readings to specific cognitive functions.
- Skin Preparation: Proper skin preparation enhances contact and reduces noise in the signals.
By strategizing how electrodes are placed, researchers can target specific areas of interest, ensuring that the data gathered is relevant and precise.
Signal Processing Techniques
Once the electrode configuration is established, the next step involves signal processing techniques. Raw EEG signals often contain noise and artifacts that can obscure the underlying brain activity. Thus, effective signal processing is necessary, and this can involve multiple methods:
- Filtering: High-pass and low-pass filters help eliminate unwanted frequency components, allowing only the relevant brainwave activity to be analyzed.
- Artifact Removal: Techniques such as Independent Component Analysis (ICA) are utilized to identify and remove eye movements or muscular activity that distort readings.
- Power Spectral Density (PSD) Estimation: This analysis helps quantify the power of different brainwave types, offering insights into the state of consciousness or cognitive engagement.
These methods enhance the clarity and fidelity of the data, ensuring that subsequent analysis is based on high-quality signals.
Data Interpretation
Data interpretation is the final phase where processed EEG results are analyzed to draw meaningful conclusions. This step requires a deep understanding of both the methodology and the neuroscience involved. Several considerations should be noted:
- Waveform Analysis: Identifying specific brainwave patterns, such as alpha, beta, and gamma waves, provides insights into different mental states.
- Comparative Studies: Comparing EEG data across different populations, such as those with neurological disorders versus healthy individuals, helps identify abnormal patterns.
- Clinical Correlations: Interpretation should also consider clinical signs or symptoms to make assessments valid and applicable.
The interpretation of EEG data is an art that combines technical skill with clinical acumen to inform treatment and further research directions.
In summary, EEG methodology involves a robust framework, starting from electrode configuration to data interpretation, requiring attention to detail and understanding of both technology and neuroscience. Proper application of these elements results in meaningful brainwave measurement that can significantly contribute to both clinical and cognitive research.
Advanced Brainwave Measurement Technologies
Advanced brainwave measurement technologies are critical in the ongoing exploration of how brainwave patterns relate to cognitive processes and health. These technologies not only offer more precise and detailed data but also expand the realm of possibility for neuroscience research and clinical applications. The integration of different methodologies allows researchers to achieve a multidimensional view of brain activity, which can inform treatment protocols and cognitive enhancement strategies. In this section, we will examine three prominent technologies: Functional Magnetic Resonance Imaging, Magnetoencephalography, and Wireless EEG Systems.
Functional Magnetic Resonance Imaging (fMRI)
Functional Magnetic Resonance Imaging, often abbreviated as fMRI, is a powerful neuroimaging technique. It assesses brain activity by detecting changes associated with blood flow, known as the hemodynamic response. This method is significant because it provides high spatial resolution, allowing researchers to localize brain activity with precision.
- Pros of fMRI
- Cons of fMRI
- Provides detailed anatomical imagery.
- High spatial resolution.
- Non-invasive; no harmful radiation.
- Lower temporal resolution than other methods.
- Sensitive to motion; participant stillness is crucial.
The practical application of fMRI spans from clinical diagnosis, such as identifying tumors and other abnormalities, to cognitive research. It helps in understanding which brain areas are active during specific tasks, showing how different regions communicate during cognitive activities.
Magnetoencephalography (MEG)
Magnetoencephalography, or MEG, measures the magnetic fields produced by neuronal activity. This technology offers unparalleled temporal resolution compared to other imaging techniques, making it particularly suited for capturing quick changes in brain activity.
- Benefits of MEG
- Exceptional temporal resolution.
- Ability to track dynamic processes in the brain in real time.
- Non-invasive and relatively comfortable for subjects.
Nonetheless, MEG's spatial localization is not as refined as fMRI. While it can detect brain activity, pinpointing the exact location can be challenging. This makes a combination of fMRI and MEG a viable option for researchers seeking comprehensive insights.
Wireless EEG Systems
Wireless EEG systems represent a significant advancement in the measurement of brainwaves. Traditional EEGs involve cumbersome wires, which can limit the natural behavior of subjects during testing. Wireless systems allow for more flexibility and realism in experiments, leading to more authentic results.
- Advantages of wireless EEG
- Enhanced mobility of subjects.
- Greater comfort leads to more natural behaviors.
- Simplifies the setup and reduces preparation time.
Overall, wireless EEG systems expand the potential for applications in various settings, including cognitive psychology, education, and rehabilitation. With these systems, data can be collected in real-world environments, thus enhancing ecological validity in research.
"The future of brainwave measurement lies in the integration of diverse technologies, allowing a comprehensive approach to studying and interpreting brain activity."
To summarize, these advanced technologies in brainwave measurement play a pivotal role in enhancing our understanding of the brain. Each technology, with its unique strengths and weaknesses, contributes to a more nuanced appreciation of brain activity, facilitating groundbreaking research and applications in the field.
Applications of Brainwave Measurement
The study of brainwave measurement extends far beyond simple data collection. Its applications impact numerous fields, particularly in clinical, cognitive enhancement, and neurofeedback training. Understanding these applications is vital for grasping the relevance of brainwave research and its potential benefits for society.
Clinical Applications
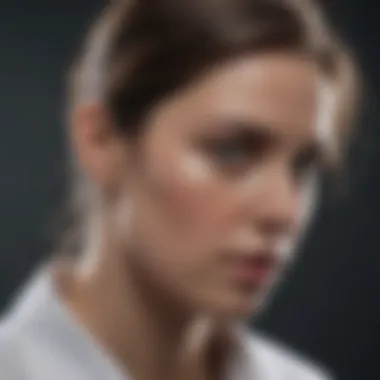
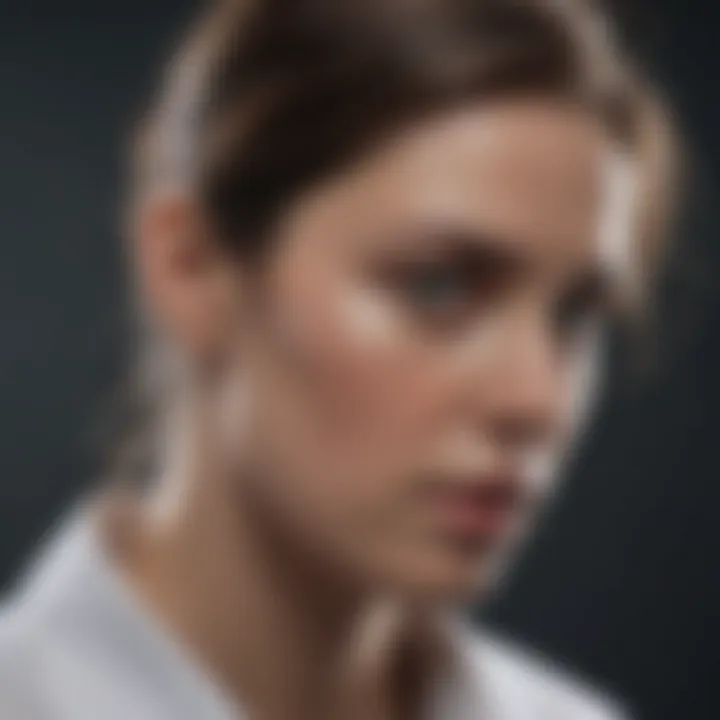
In the medical field, brainwave measurement is crucial for diagnosing and treating various neurological disorders. Conditions such as epilepsy, sleep disorders, and cognitive impairments can be better managed through the insights gained from monitoring brainwaves.
For instance, electroencephalography (EEG) is routinely used to detect abnormal electrical activity in patients with epilepsy, providing guidance on appropriate treatments. Additionally, sleep studies utilize EEG data to identify sleep patterns, aiding in the diagnosis of sleep apnea and other disorders. Such clinical applications not only enhance patient outcomes but also inform ongoing research into the etiology and progression of neurological diseases.
Cognitive Enhancement
Another vital domain is cognitive enhancement. Understanding brainwaves enables researchers and individuals to boost cognitive performance. Various techniques employ brainwave measurement to enhance focus, relaxation, and overall mental agility. Products like neurostimulation devices often leverage this knowledge to optimize the user’s cognitive state by modulating brainwave activities. This area holds promising potential, especially in educational settings, where enhancing learning experiences could lead to improved academic performance.
Neurofeedback Training
Neurofeedback training is an emerging practice that applies principles of brainwave measurement to facilitate self-regulation of brain activity. Through real-time feedback, individuals can learn to alter their brainwave patterns, which can have significant therapeutic effects. This technique has shown potential in treating conditions such as ADHD and anxiety, combining elements of traditional therapy with innovative technology. Participants can work directly with their brain's data, fostering learning that can lead to lasting change in behavior and cognitive processes.
"Neurofeedback holds potential as a non-invasive method for improving mental health and cognitive skills, opening doors to personalized treatment protocols."
Ethical Considerations in Brainwave Research
The exploration of brainwave measurement is not only a scientific endeavor but also embodies deep ethical concerns. As researchers venture further into understanding brain activity through technologies such as EEG, FMRI, and MEG, the ethical implications of their work become increasingly significant. This section outlines the critical ethical considerations that must be taken into account, including informed consent, privacy, and the potential misuse of data. Addressing these issues ensures that research is conducted responsibly, fostering trust between scientists and participants, and promoting the advancement of neuroscience as a discipline that respects individual rights.
Informed Consent and Privacy
Informed consent serves as a cornerstone in ethical research practices. It ensures that participants are fully aware of what their involvement entails, including potential risks and benefits. In the context of brainwave measurement, participants should clearly understand how their brain data will be collected, analyzed, and utilized. This involves an obligation for researchers to provide detailed explanations about the procedures and implications of their participation.
Additionally, privacy is a significant concern in brainwave research. Brain activity data can reveal intimate details about an individual's mental state and cognitive processes. It is crucial for researchers to implement stringent data protection measures to maintain participant anonymity. Breaches of privacy can lead to significant psychological harm, as well as potential social repercussions. Therefore, ensuring the confidentiality of brainwave data is as important as gaining informed consent.
"Respect for persons necessitates that participants are treated as autonomous agents capable of making decisions regarding their involvement in research."
(Source: Wikipedia - Informed Consent)
Potential Misuse of Data
The potential for data misuse in brainwave research raises another layer of ethical complexity. As advances in technology allow for more detailed and precise brain measurements, the possibility of this information being exploited grows. For instance, data could be used in ways that infringe on personal liberties, such as profiling individuals based on their brain activity or creating predictive models that could unfairly stigmatize certain groups.
Moreover, organizations may utilize this data without proper oversight, leading to possible unethical applications in areas such as marketing, surveillance, or even in law enforcement. Researchers must advocate for protocols that limit access to sensitive data and promote its ethical use.
In summary, as brainwave measurement techniques advance, it is imperative to maintain ethical standards that protect the rights of participants. Researchers need to prioritize informed consent and privacy while actively mitigating any potential for misuse of the data collected. Such considerations are not just regulatory obligations but essential for the integrity of neuroscience research.
Future Directions in Brainwave Measurement
The domain of brainwave measurement is rapidly evolving. Understanding the future directions in this field is essential as these advancements can greatly influence both scientific discovery and practical application. With new technologies and methodologies emerging, researchers are poised to explore uncharted territories that hold significant implications for neuroscience. Innovative approaches such as integrating brainwave data with artificial intelligence and expanding research frontiers will change how we perceive brain activity and its impact on human behavior.
Integration with Artificial Intelligence
Artificial intelligence (AI) is making profound inroads into brainwave measurement. AI systems can analyze vast quantities of EEG data more swiftly and accurately than humans. This efficiency enables researchers to identify patterns that may go unnoticed through traditional analysis. Machine learning algorithms, for example, can learn from existing datasets and improve their predictive accuracy over time.
The potential applications of AI in this field are extensive:
- Enhanced data interpretation: Sophisticated algorithms can interpret patterns in brainwaves related to specific cognitive states or emotional responses.
- Personalized medicine: AI can assist in developing tailored interventions for neurological conditions, predicting patient responses to treatments based on brainwave data.
- Real-time monitoring: AI-driven solutions can provide instantaneous feedback, crucial for tasks requiring immediate awareness of brain states, such as neurofeedback training.
Nonetheless, the integration of AI also raises challenges. Ensuring the ethical use of data derived from brainwave measurements and maintaining privacy stand as pivotal areas for consideration.
Expanding Research Frontiers
As technology advances, researchers are continually pushed to expand the frontiers of brainwave measurement. One significant trend is the integration of multimodal methodologies. By combining EEG with other neuroimaging techniques, such as fMRI and MEG, researchers can glean a more comprehensive understanding of brain processes. This fusion of data types can offer insights into how brain activity correlates with complex behaviors.
Other avenues for exploration include:
- Neuroplasticity Investigation: Understanding how brainwaves adapt in response to learning and experience is crucial for advancing educational and rehabilitation strategies.
- Cognitive Robotics: Applying brainwave data can aid in developing robots that respond to human thought patterns, making them more intuitive and effective in performing tasks alongside people.
- Mental Health Research: Investigating correlations between brainwave patterns and mental health disorders can pave the way for novel therapeutic interventions.
Advancements in brainwave measurement technology are necessary for securing the future of neuroscience. The challenges in ethical considerations, data interpretation, and interdisciplinary collaboration will shape these developments significantly.
The future of brainwave measurement is promising, with the potential for groundbreaking discoveries on the horizon. By embracing both the technological advancements and the ethical dimensions, this field can unlock new understandings of the human brain.
Closure
The importance of the conclusion in this article lies in its ability to consolidate the various elements discussed regarding the measurement of brainwaves. It serves to synthesize insights from methodology, technological advancements, applications, and ethical considerations. This holistic view is crucial for providing a comprehensive understanding of how brainwaves can be measured and interpreted.
Key insights summarized include:
- The varying types of brainwaves and their significance to cognitive processes.
- The evolution of methodologies, particularly the introduction of EEG and its refinements over the years.
- The operational shift brought about by advanced technologies like fMRI and MEG, which have enhanced the precision of brainwave measurement.
- The applicative spectrum of brainwave data across clinical settings, cognitive enhancements, and neurofeedback training.
- The ethical dimensions that must be navigated as research continues to evolve and the potential for misuse of brain data.
"Understanding brainwaves provides insight into the workings of the human mind, fundamentally bridging gaps between neuroscience, psychology, and technology."
This conclusion not only emphasizes the interconnectedness of the discussed elements but also highlights their implications for future research. The integration of these insights can inform educators, clinicians, and researchers about the value of brainwave measurement. As the field progresses, understanding these variables becomes essential for those involved in related areas of practice and study.
In summary, the journey through the intricate landscape of brainwave measurement reinforces its importance in advancing neuropsychological knowledge and enhancing therapeutic approaches. It lays a foundational framework for continued exploration, inviting ongoing scholarly dialogue and innovative developments in neuroscience.