Exploring the Complex World of Polyethylene Plastics
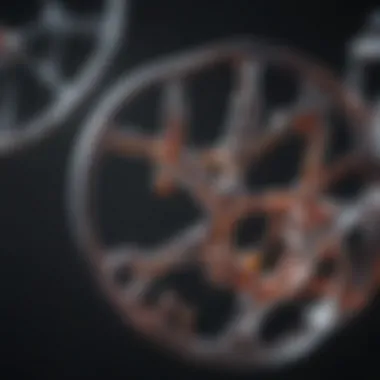
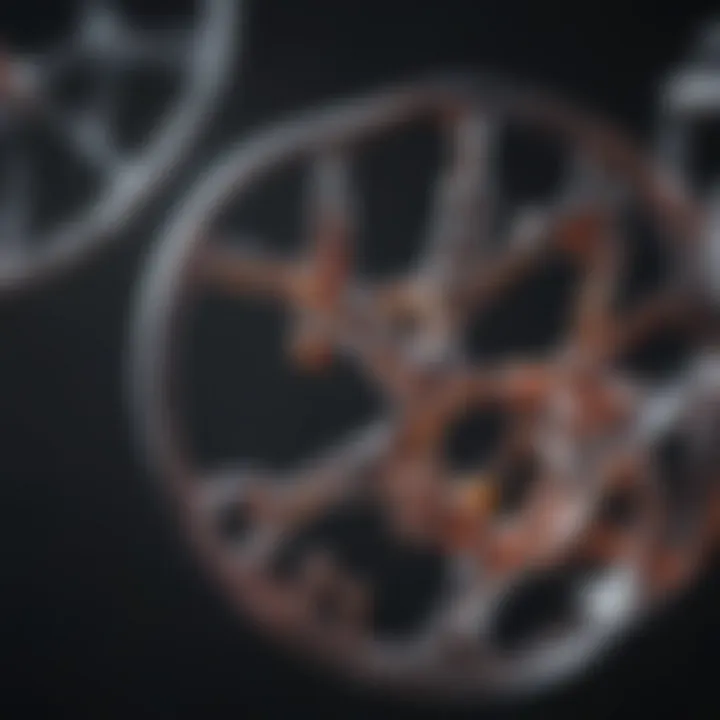
Intro
Polyethylene plastics play a critical role in numerous aspects of modern life and industry. This article delves into the core elements of polyethylene, including its chemical structure, various types, and the processes involved in its production. The examination will also touch upon the applications of polyethylene, elucidating how it shapes industries ranging from packaging to automotive. Furthermore, the environmental implications of polyethylene use and recycling mechanics are essential components of this discussion. Understanding these factors sheds light on polyethylene's significance in contemporary society, not only for industrial purposes but also for sustainability efforts.
Key Findings
Major Results
The examination of polyethylene reveals several notable findings:
- Chemical Composition: Polyethylene is primarily a polymer composed of repeating units of ethylene, a simple hydrocarbon. Its properties can vary based on the arrangement of molecules.
- Manufacturing Techniques: Polyethylene is manufactured through processes such as polymerization, utilizing high pressure and specific catalysts.
- Types and Applications: There are various types of polyethylene, including Low-Density Polyethylene (LDPE) and High-Density Polyethylene (HDPE), each suited to different applications, reflecting its versatility.
- Environmental Concerns: While polyethylene is widely used, its environmental impact is a growing concern, particularly regarding pollution and waste management.
- Recycling Processes: There are established methods for recycling polyethylene, which help mitigate some negative effects on the environment.
Discussion of Findings
The discussion surrounding polyethylene encompasses both its advantages and shortcomings. On one hand, its versatility and light weight make it an essential material in multiple sectors. For instance, in the packaging industry, polyethylene's properties contribute to the longevity and safety of products.
However, the environmental challenges cannot be overlooked. The increasing accumulation of polyethylene waste in landfills and oceans exemplifies the urgent need for effective recycling solutions. A concerted effort from both industry stakeholders and consumers is necessary to address these problems.
"Understanding polyethylene's life cycle can guide better practices and innovation in sustainable materials management."
Methodology
Research Design
This inquiry adopts a mixed-method approach, combining qualitative insights from industry reports and quantitative data from manufacturing sources. This design allows for a holistic view of polyethylene plastics, capturing both the technical and sociocultural dimensions.
Data Collection Methods
Data was collected through various means, including:
- Academic journals and articles from experts in polymer chemistry.
- Industry reports from companies involved in polyethylene production.
- Environmental studies highlighting the impact of plastic waste.
By synthesizing these diverse sources, the article provides a rigorous examination of polyethylene plastics, catering to students, researchers, educators, and professionals seeking an in-depth understanding of the topic.
Foreword to Polyethylene Plastics
Polyethylene plastics play a crucial role in modern society. They are among the most widely used types of plastic due to their versatility and cost-effectiveness. Understanding their properties, applications, and impact on the environment is essential for students and professionals alike. This section provides a comprehensive overview of polyethylene, highlighting its significance across various industries.
Definition and Characteristics
Polyethylene is a polymer composed of long chains of repeating ethylene units. It comes in different forms, experiencing variations in density, branching, and molecular weight. These characteristics determine its physical properties, influencing its behavior in applications. For instance, low-density polyethylene (LDPE) is flexible and used in plastic bags, while high-density polyethylene (HDPE) is more rigid and commonly found in containers and pipes.
Some notable characteristics of polyethylene include:
- Chemical resistance: Polyethylene is resistant to various chemicals, making it suitable for packaging and containers.
- Moisture resistance: This property is critical in food packaging and many other applications.
- Low density: It is relatively light, making it economical for transport and usage.
- High tensile strength: It can bear significant weight, which is crucial in many structural applications.
Historical Context
The story of polyethylene began in 1898, when the German chemist Hans von Pechmann accidentally synthesized it while experimenting with diazomethane. However, commercial production did not begin until the 1930s, when it was independently developed by two different teams: one in the UK by Eric Fawcett and Reginald Gibson, and the other in the US by Wallace Carothers and his team at DuPont.
Once discovered, polyethylene's uses expanded rapidly. In World War II, it was employed for radar insulation, further proving its utility. Post-war demand for consumer products led to an explosion in manufacturing. Today, polyethylene is integral in packaging, automotive, and construction sectors among others. Its adaptability and cost efficiency continue to influence its historical relevance.
"The versatility and low cost of polyethylene have made it a staple material for multiple industries, highlighting its lasting impact on both the economy and daily life."
This section sets the stage for a deeper exploration of polyethylene's chemical composition, manufacturing processes, and its various types. Understanding these fundamentals is key to grasp its applications and implications in today's world.
Chemical Composition of Polyethylene
The chemical composition of polyethylene is crucial to understanding its various properties and applications. Polyethylene is primarily composed of carbon and hydrogen atoms, forming a simple, linear structure. This structure is responsible for the material's unique characteristics, such as its flexibility, strength, and resistance to chemicals.
Molecular Structure
Polyethylene's molecular structure consists of long chains of repeating units called mer units. Each mer unit is a simple ethylene molecule (C₂H₄). These chains can vary in length and arrangement. The arrangement of the chains contributes to the different types of polyethylene, such as low-density and high-density polyethylene.
- Low-Density Polyethylene (LDPE): This has a branched structure, leading to a lower density and higher flexibility.
- High-Density Polyethylene (HDPE): Characterized by a more linear structure, it has a higher density and greater tensile strength than LDPE.
The degree of polymerization, which refers to the number of mer units in a chain, varies widely. This variation affects physical properties such as melting point, density, and mechanical strength. As a result, understanding the molecular structure is fundamental to tailoring polyethylene for specific purposes in various industries.
Polymerization Process
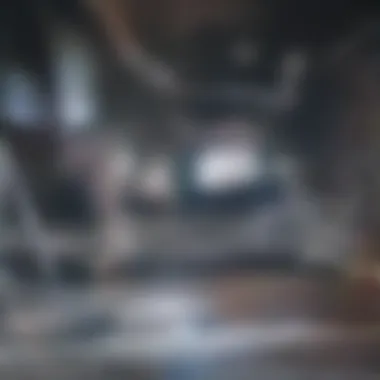
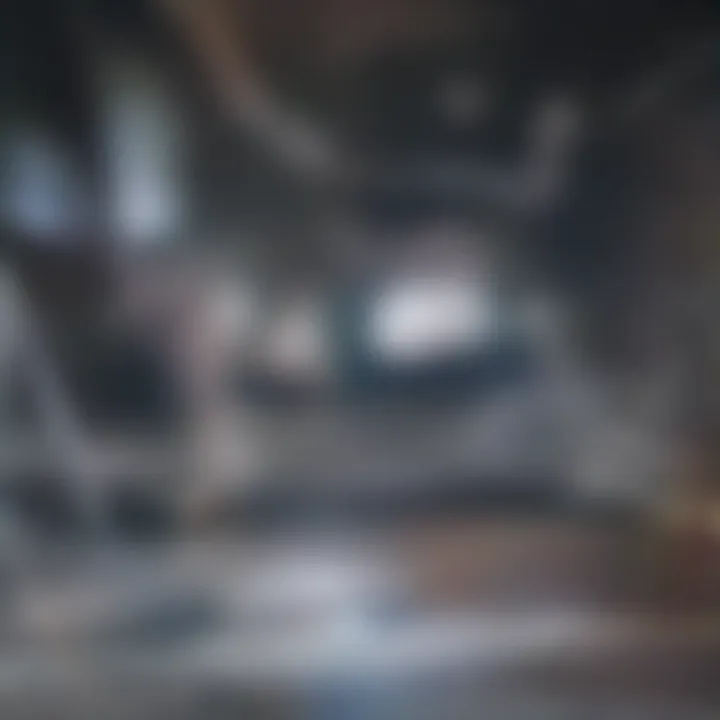
The polymerization process of polyethylene involves converting ethylene gas into a polymer through chemical reactions. There are several methods to achieve this, including:
- Gas Phase Polymerization: This method involves ethylene gas being polymerized in a gaseous state. It is efficient and allows for continuous production.
- Slurry Phase Polymerization: In this method, ethylene is polymerized in a liquid slurry. The solid polymer is suspended in a non-reactive liquid, which helps control temperature and reaction rate.
- Solution Phase Polymerization: Ethylene is polymerized in a solvent, allowing for precise control over the reaction conditions. This method is advantageous for producing specific grades of polyethylene.
Understanding the polymerization process is vital. It influences not only the molecular structure but also the physical properties of the final product, affecting application suitability in industries ranging from packaging to automotive.
"The chemical composition of polyethylene plays a central role in determining its suitability for various applications, influencing everything from environmental impacts to recycling potential."
Types of Polyethylene Plastics
Understanding the various types of polyethylene plastics is crucial for several reasons. Each type possesses unique properties, making it suitable for specific applications. By delving into the characteristics of each variant, one can appreciate the versatility of polyethylene, which plays a significant role in modern materials science and industry. This section will explore low-density polyethylene, high-density polyethylene, linear low-density polyethylene, and ultra-high molecular weight polyethylene, emphasizing their benefits and considerations in various fields.
Low-Density Polyethylene (LDPE)
Low-Density Polyethylene, commonly known as LDPE, is one of the oldest forms of polyethylene. Its structure consists of a high degree of branching which gives it a lower density compared to other types. This branching contributes to its flexibility and low tensile strength. LDPE is often used in applications requiring stretchability, such as plastic bags and various types of packaging materials.
The main characteristics of LDPE include:
- Flexibility: It is highly adaptable, which makes it suitable for objects that need to be easily manipulated.
- Chemical Resistance: LDPE withstands various chemicals, making it a solid choice for containers and packaging of chemical products.
- Lightweight: This characteristic reduces shipping costs and increases convenience in production and usage.
However, LDPE is less durable under stress and is not ideal for products that require high strength.
High-Density Polyethylene (HDPE)
High-Density Polyethylene, abbreviated as HDPE, is another prevalent type of polyethylene. Its molecular structure is less branched than that of LDPE. This results in a higher density and strength. HDPE is well-known for its rigidity and is often utilized in applications demanding sturdiness, such as containers, pipes, and large construction materials.
Key features of HDPE are:
- Strength: Its high density gives it impressive strength and makes it suitable for heavy-duty applications.
- Impact Resistance: HDPE can withstand impacts better than other polyethylene types.
- UV Resistance: This makes it ideal for outdoor applications since it can resist degradation when exposed to sunlight.
While HDPE has numerous benefits, its rigidity can limit its usability in applications that require flexibility.
Linear Low-Density Polyethylene (LLDPE)
Linear Low-Density Polyethylene, or LLDPE, combines properties from LDPE and HDPE. It is known for its linear structure with short branches, which enhances strength while maintaining flexibility. LLDPE is commonly used in film production, packaging, and applications requiring stretchability combined with strength.
Some notable attributes of LLDPE are:
- Durability: Offers improved puncture resistance compared to LDPE.
- Versatile Manufacturing: Suitable for various processing methods, including blow molding and extrusion.
- Cost-Effective: Often provides a balance between performance and manufacturing costs.
These advantages can lead to increased usage in several sectors, elevating its importance in contemporary materials.
Ultra-High Molecular Weight Polyethylene (UHMWPE)
Ultra-High Molecular Weight Polyethylene is characterized by its extremely high molecular weight, contributing to its exceptional toughness and wear resistance. UHMWPE is not commonly used in regular consumer goods but holds significant value in specialized applications such as medical devices, industrial machinery, and high-performance gear.
The defining features of UHMWPE include:
- Exceptional Wear Resistance: This characteristic makes it ideal for applications involving friction and abrasion.
- Low Coefficient of Friction: UHMWPE surfaces are smooth, reducing friction which is crucial in many machinery applications.
- Biocompatibility: UHMWPE is often used in orthopedic implants because it's safe for use within the human body.
Despite the benefits, UHMWPE generally comes at a higher cost compared to other polyethylene types, which can deter its use in more budget-conscious applications.
Manufacturing Processes of Polyethylene
The manufacturing processes of polyethylene are crucial for understanding its properties and applications. These processes dictate not only the material's characteristics but also its usability across diverse industries. The importance of these processes extends beyond the production itself; they influence economic factors, efficiency, and environmental impact. Each method of production comes with its own advantages and drawbacks, shaping how polyethylene is utilized and perceived in the market.
Extraction of Raw Materials
The first step in the manufacturing of polyethylene is the extraction of raw materials, primarily ethylene, which is derived from fossil fuels. Ethylene is primarily obtained from crude oil or natural gas through a process known as cracking. This involves heating these hydrocarbons to high temperatures, resulting in the production of ethylene and other byproducts. The availability and cost of these fossil resources are significant considerations, impacting the overall sustainability of polyethylene production. Additionally, the extraction process itself raises environmental concerns, including greenhouse gas emissions and potential spills.
Polymer Production Techniques
Polyethylene can be produced through several polymerization techniques, which differ in their conditions and resulting properties. Understanding these methods is vital for improving production efficiency and product quality.
Gas Phase Polymerization
Gas phase polymerization involves the conversion of gaseous ethylene into polyethylene within a reactor. This technique is notable for producing high-density polyethylene (HDPE) and allows for greater control over molecular weight distribution. A significant aspect of gas phase polymerization is its efficiency; it typically employs a fluidized bed reactor that promotes continuous cycling of materials, which leads to lower production costs.
However, one unique feature of this technique is its ability to produce materials with a broad range of densities, making it versatile for various applications. Despite its benefits, the process can be sensitive to feedstock variability, which may affect consistency in product quality.
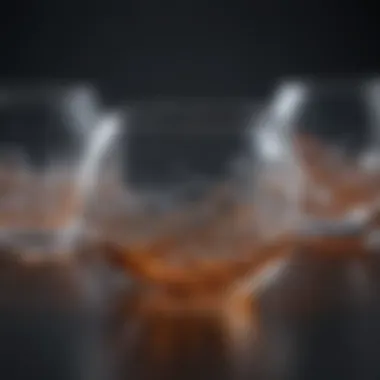
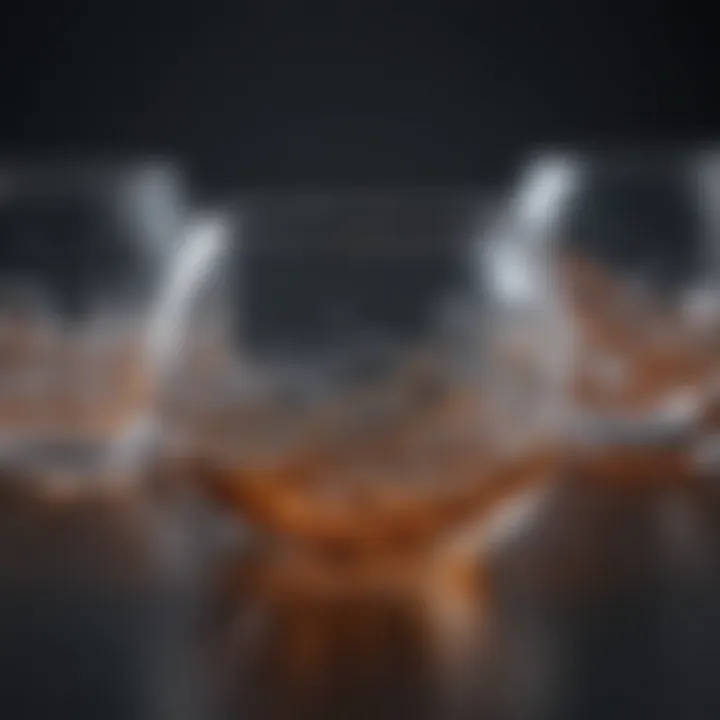
Slurry Phase Polymerization
In this method, ethylene is dissolved in a hydrocarbon solvent, creating a slurry of polyethylene particles suspended in the liquid. Slurry phase polymerization is widely recognized for yielding low-density polyethylene (LDPE) and HDPE. Its key characteristic is the lower reaction temperatures required compared to gas phase polymerization, which can result in enhanced safety by reducing risks associated with high temperatures.
A unique advantage of slurry phase polymerization is its flexibility in producing different types of polyethylene by adjusting catalyst systems and reaction conditions. On the downside, the presence of solvent poses challenges in terms of energy consumption for drying and recycling.
Solution Phase Polymerization
Solution phase polymerization involves dissolving ethylene in a solvent, allowing a chemical reaction to occur that creates polyethylene. This method is attractive for its ability to produce very high molecular weight polyethylene (UHMWPE), which is crucial for applications requiring exceptional strength and durability. The key characteristic of this process is its operational simplicity, as it operates under milder conditions compared to gas phase methods.
A significant benefit of solution phase polymerization is the easier handling of heat due to the solvent's capacity to absorb excess heat during reactions. However, it can involve higher operational costs due to the need for solvent recovery and the potential environmental concerns associated with solvent disposal.
The choice of polymerization process ultimately impacts the properties of polyethylene, leading to its diverse applications in packaging, construction, and consumer goods.
Applications of Polyethylene Plastics
Polyethylene plastics play a crucial role in various sectors, shaping the way we package, consume, and even build. Their versatility is unmatched when compared to other materials. The widespread adoption of polyethylene stems from its unique properties, enabling it to serve diverse applications effectively. As a major contender in the plastics industry, understanding its applications is essential for grasping its importance in modern society.
Packaging Industry
Food Packaging
Food packaging is perhaps one of the most significant applications of polyethylene. It is prominently used to store a variety of food items, providing a barrier against moisture, oxygen, and other contaminants. The key characteristic of polyethylene in food packaging is its ability to be sealed tightly, ensuring that food remains fresh for an extended period. This feature makes it a beneficial choice for manufacturers seeking to enhance shelf life. However, while the convenience of polyethylene is undeniable, concerns regarding plastic waste and food safety must be considered in its continued use.
Cosmetic Packaging
Cosmetic packaging employs polyethylene extensively to house personal care products such as lotions and creams. This material is lightweight and resistant to many chemical reactions, making it ideal for containing a variety of substances. A notable aspect of polyethylene cosmetic packaging is its clear nature, allowing consumers to see the product inside. This transparency can play a strong role in influencing purchasing decisions. However, reliance on single-use plastics within this sector raises questions about sustainability and environmental impact, leading to calls for more eco-friendly alternatives.
Industrial Packaging
Industrial packaging utilizes polyethylene primarily for its strength and durability. Various forms, including stretch films and heavy-duty sacks, are commonly employed to safely transport goods. The essential characteristic of polyethylene in this context is its resistance to punctures and tears, which safeguards the integrity of packed products during transit. This makes it a favorable choice among industries requiring reliable packaging solutions. Yet, the bulky nature of some polyethylene products can lead to increased waste, prompting discussions on recycling and reuse efforts within the industrial sector.
Consumer Goods
Household Items
Polyethylene serves a wide array of household items, from containers to furniture components. Its lightweight nature and resistance to corrosion make it especially useful in everyday products. This durability is one of the main reasons why polyethylene is often chosen for household applications. However, the presence of household items made from polyethylene also raises awareness about their potential environmental effects, largely due to improper disposal practices.
Toys
In the toy industry, polyethylene is frequently used due to its non-toxic properties. This characteristic ensures safety for children playing with these products. Furthermore, toys made with polyethylene are often durable and lightweight. However, the challenge arises from ensuring that these toys are designed for longevity to avoid contributing to plastic waste in landfills.
Stationery
Stationery items, like folders and binders, often incorporate polyethylene due to its flexibility and resistance to wear. This material provides a cost-effective solution for manufacturers, leading to popular products that can withstand frequent use. The availability of various colors and textures adds aesthetic appeal to stationery items. Nonetheless, the environmental concerns associated with polyethylene underline the necessity for consumers to support recycling initiatives and choose more sustainable options.
Construction and Design
In the construction and design sectors, polyethylene holds significant sway. As a waterproofing agent, it is used in various applications, proving invaluable for keeping structures dry. Moreover, its flexibility enables easy installation in various design frameworks. While its utility is clear, the challenge rests in balancing performance with eco-friendly practices, as the construction industry often leads to substantial plastic waste.
"The use of polyethylene plastics in the building sector highlights a critical intersection between functionality and sustainability."
Through various applications across distinct industries, polyethylene's multifaceted capabilities reveal both benefits and challenges. As the world moves towards more sustainable practices, evaluating the role of polyethylene within these domains remains vital.
Environmental Considerations
The discussion about polyethylene plastics cannot be complete without addressing the environmental considerations associated with their production and use. This section explores various aspects of how polyethylene impacts both the environment and wildlife, focusing on pollution, waste management, and the broader ecological footprint. The significance of these topics is crucial for understanding the sustainability of polyethylene plastics in modern society.
Pollution and Waste Management
Polyethylene contributes considerably to global pollution. Its wide usage in packaging, items, and single-use products leads to significant waste generation. Many polyethylene products are not biodegradable, which means they can persist in the environment for hundreds of years. When improperly disposed of, these plastics can end up in landfills, oceans, and natural habitats.
The management of polyethylene waste is therefore an urgent issue. Effective strategies need to be implemented to handle plastic pollution, which can be achieved through:
- Improved recycling programs: Enhancing the efficiency and accessibility of recycling facilities can encourage the proper handling of used polyethylene products.
- Public awareness campaigns: Educating consumers about the importance of proper disposal and the benefits of recycling can lead to decreased waste.
- Policy initiatives: Governments can introduce regulations to limit single-use plastics and incentivize companies to adopt sustainable practices.
"A comprehensive approach to waste management is pivotal to mitigate the impacts of polyethylene pollution, ensuring a healthier environment for future generations."
These practices can help reduce the volume of polyethylene waste, foster a culture of recycling, and ultimately mitigate the negative impacts of plastic pollution.
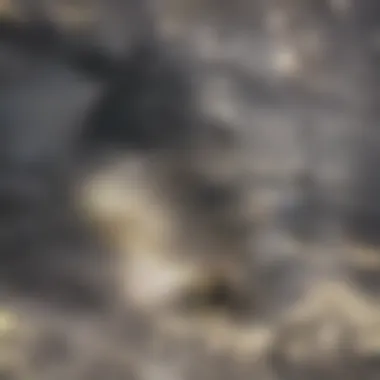
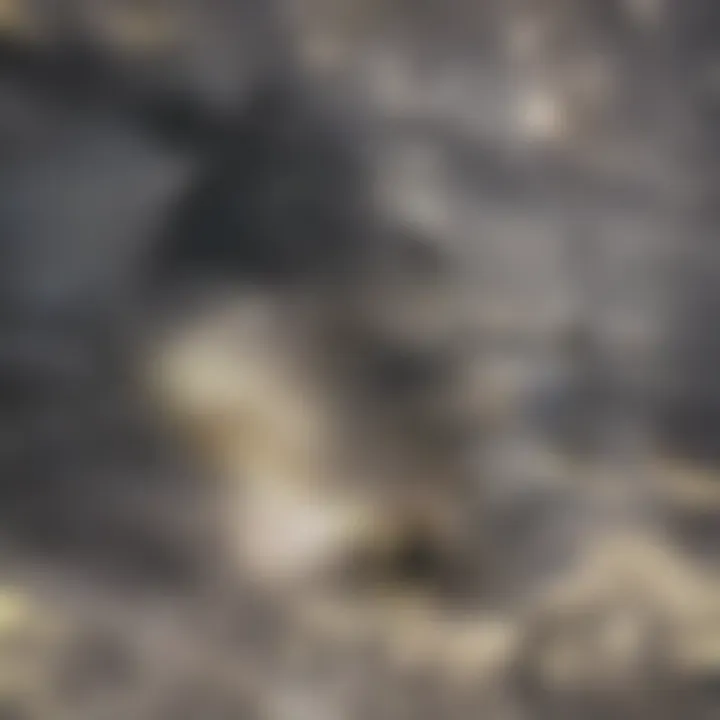
Impact on Wildlife
The effects of polyethylene pollution extend beyond human activities; they pose serious risks to wildlife. Animals can mistake plastic items for food, leading to ingestion, which is often fatal. Marine species, in particular, are greatly affected. Plastic debris in oceans contributes to the decline of many marine habitats and species.
Key impacts include:
- Entanglement: Many aquatic animals become trapped in various forms of plastic, which can cause injury or death.
- Microplastics: As polyethylene breaks down into smaller particles, it enters the food chain, affecting various organisms. These microplastics can disrupt feeding behaviors, reproductive functions, and overall health in wildlife.
- Habitat degradation: Plastics that accumulate in natural environments can alter habitats, making them less hospitable for many species.
To combat these issues, it is essential to implement stricter regulations surrounding plastic production and disposal. Furthermore, initiatives aimed at restoring natural habitats can help support wildlife affected by plastic pollution. Addressing these environmental considerations is crucial for the sustainable future of both polyethylene and its impact on ecosystems.
Recycling of Polyethylene
Recycling of polyethylene is a crucial aspect of the discussion around this type of plastic. It not only helps address the pressing environmental issues related to plastic waste but also provides a means to recover valuable materials. By embracing recycling, industries and individuals contribute to a circular economy, minimising the need for new raw materials and reducing energy consumption associated with plastic production. This section elucidates the types of recycling methods available and the challenges faced in recycling polyethylene plastics.
Types of Recycling Methods
Mechanical Recycling
Mechanical recycling is a process that involves the physical reprocessing of plastic waste. This method entails shredding the polyethylene into small pieces, followed by washing and melting them to create new products. One key characteristic of mechanical recycling is its simplicity and effectiveness in processing clean polyethylene waste. It is widely regarded as a beneficial choice for handling common types of polyethylene like LDPE and HDPE.
A unique feature of mechanical recycling lies in its ability to maintain the basic polymer structure during the process. This helps produce high-quality recycled resin, which can be used in manufacturing new products. However, there are disadvantages. Contamination with other materials can compromise the quality of the recycled product, limiting its applications.
Chemical Recycling
Chemical recycling offers an alternative approach, focusing on breaking down polyethylene back into its monomer form. This method allows for the production of new polyethylene from previously used materials. The main advantage of chemical recycling is its capability to handle contaminated plastics and produce high-quality outputs.
Chemical recycling is known for its ability to recover not just materials but also energy. A unique feature of this process is that it can theoretically produce an infinite loop of recycling without losing the polymer quality. Despite these benefits, challenges include high operational costs and the need for sophisticated technology, which can limit accessibility for smaller recycling facilities.
Challenges in Recycling
The recycling of polyethylene faces significant challenges. One of the primary obstacles is the low rates of recycling for certain types of polyethylene, particularly LDPE. Many communities lack the infrastructure to recycle these materials efficiently.
Moreover, the contamination of plastic waste remains a pivotal issue. Labels, food residues, and other impurities can hinder recycling processes, leading to lower quality recycled materials. Additionally, consumer attitudes toward recycling can vary, affecting participation rates.
Future Prospects of Polyethylene
The future prospects of polyethylene are crucial to understanding its evolving role in various industries and its wider environmental implications. As the global demand for plastic products continues to rise, various innovations in the production and recycling processes are emerging to mitigate the negative impacts associated with plastic use. This section delves into some key innovations and the significance of exploring alternative materials in the context of sustainability.
Innovations in Production
Recent advancements in the production of polyethylene focus on enhancing efficiency and minimizing environmental footprints. The introduction of catalysts such as metallocenes has revolutionized the polymerization processes, leading to more controlled molecular architectures. This results in a variety of polyethylene types with tailored properties suitable for specific applications.
- Advanced Polymerization Techniques: Innovations in polymerization methods include the use of higher reaction temperatures and pressures, which can significantly reduce energy consumption.
- Renewable Feedstocks: Recently, there has been a push towards utilizing renewable resources instead of fossil fuels for the production of polyethylene. Biobased polyethylene, produced from sugarcane, is one promising alternative.
- Process Optimization: Implementing process optimization methods, such as continuous flow systems, can enhance production rates while reducing waste generation and energy needs.
These advancements not only improve production outcomes but also demonstrate a commitment to sustainability. By reducing resource dependency and environmental impact, the future of polyethylene production is likely to lean towards greener practices.
Emerging Alternatives and Bioplastics
The rise of bioplastics presents fertile ground for research and development in the field of polyethylene alternatives. As concerns about plastic pollution grow, the interest in bioplastics has surged, providing a crucial path forward.
- Types of Bioplastics: These can be categorized as biodegradable and non-biodegradable, each with its own set of characteristics and applications.
- Success Stories: Companies like NatureWorks with their Ingeo product line have demonstrated the commercial viability of bioplastics made from renewable resources.
- Challenges to Overcome: While promising, bioplastics face challenges such as cost competitiveness, scalability of production, and infrastructure for effective composting.
It is evident that the exploration of alternatives to petroleum-based polyethylene is not just an option but a necessity in striving for a sustainable future. The continuous research into bioplastics feeds the hope of creating materials that not only meet the demands of modern society but do so in a manner that respects our environment. This forward-thinking approach reflects the shifting paradigm in which industries operate, emphasizing a more responsibly sourced and utilized material landscape.
"The transition to bioplastics is not merely a trend; it represents a fundamental shift in tackling plastic waste and promoting environmental stewardship."
As we move forward, the interplay between innovations in polyethylene production and the emergence of alternatives will shape the landscape of materials science and sustainability.
Epilogue
In summarizing the insights shared throughout this article, the conclusion serves as a crucial component in consolidating knowledge on polyethylene plastics. This section not only brings together the key points covered but also elucidates the role that polyethylene plays in various domains, including industry, consumer products, and its environmental implications.
Recap of Key Points
The exploration of polyethylene has unveiled several essential findings:
- Definition and Characteristics: Understanding what polyethylene is and its unique properties sets a foundational perspective on its applications.
- Chemical Composition: The molecular structure and polymerization processes of polyethylene underline its versatility and adaptability.
- Types of Polyethylene: Recognizing the differences between LDPE, HDPE, LLDPE, and UHMWPE clarifies their specific uses and strengths in various contexts.
- Manufacturing Processes: The methods of extracting raw materials and producing polyethylene are pivotal for quality and sustainability considerations.
- Applications: Polyethylene's extensive utilization across industries such as packaging, consumer goods, and construction highlights its importance in daily life.
- Environmental Considerations: Addressing the pollution, waste management, and wildlife impact related to polyethylene stresses the need for responsible usage.
- Recycling Methods: Identifying types and challenges of recycling polyethylene illustrates the complexity of mitigating its environmental effects.
- Future Prospects: Innovations and sustainable alternatives present opportunities for improving the life cycle of polyethylene products.
The Role of Polyethylene in Sustainability
Polyethylene can significantly influence sustainable practices in the modern world. Its recycling potential is a major factor in minimizing environmental harm. Transitioning to mechanical recycling and chemical recycling can not only reduce waste but also conserve resources necessary for new polyethylene production. Furthermore, innovations in bioplastics are propelling the industry toward more sustainable materials.
"The ability to recycle polyethylene effectively can lead to a substantial reduction in the ecological footprint of plastic products."
Sustainable practices involving polyethylene enhance public awareness of environmental issues related to plastic usage. As research continues, the focus on achieving a circular economy in plastics could further strengthen polyethylene's role as a responsible and adaptable option in multiple sectors.
Ultimately, understanding polyethylene’s multifaceted nature and addressing its challenges are imperative for both commercial success and environmental stewardship. This conclusion synthesizes not just knowledge but the need for conscientious action toward a more sustainable future.