Understanding Steel Charpy Impact Test Results
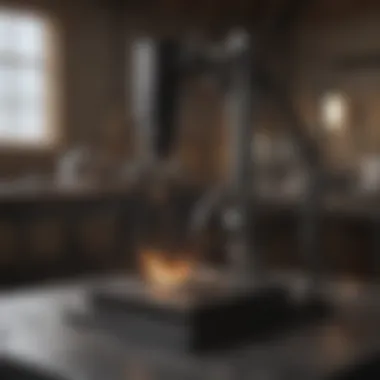
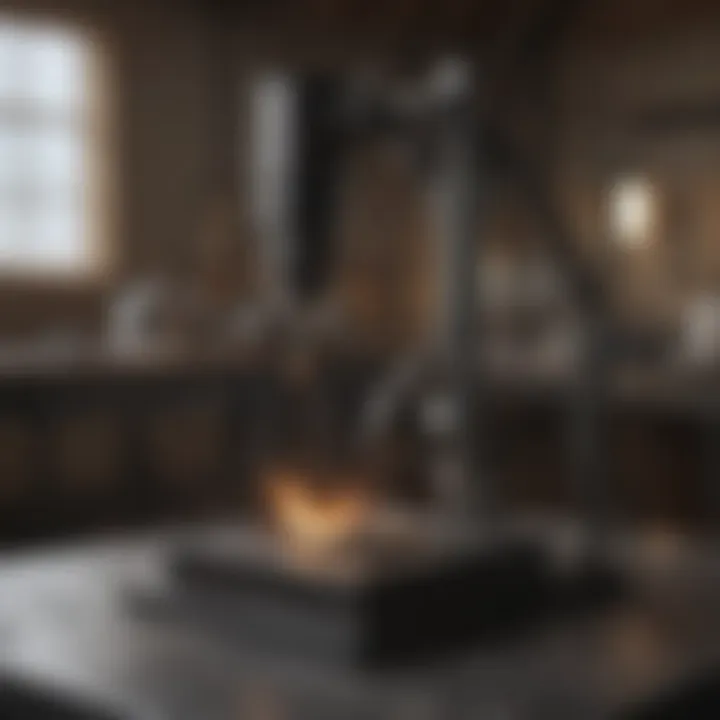
Intro
The Charpy impact test is a pivotal method employed in assessing the ductility and toughness of steel. It is essential in materials science and engineering fields, where understanding a material's response to sudden forces can dictate its efficacy in various applications. The results from this test can provide insights into the behavior of steel under stress, particularly in scenarios where impact resistance is crucial.
This article aims to thoroughly explore the Charpy impact test results associated with steel. It will cover the key findings, delve into the methodology of the test, and evaluate the significance of the outcomes in real-world applications.
Understanding how the Charpy test functions and interpreting its results can help students, researchers, and professionals alike in making informed decisions regarding material selection and performance. As industries evolve, so do the standards and research developments pertaining to steel testing, making this examination timely and pertinent.
In closing this introduction, we underscore the importance of the Charpy impact test in modern engineering and manufacturing, and how its outcomes influence not just theoretical research but practical applications as well.
Key Findings
Major Results
The Charpy impact test quantifies the ability of steel to absorb energy during fracture. Notable findings from recent analyses reveal:
- Energy Absorption: The test measures the energy absorbed by a material when a standardized striker hits it. This value is expressed in joules and indicates the toughness of the steel.
- Temperature Dependency: Results are significantly influenced by temperature, affirming that toughness typically increases at higher temperatures.
- Notch Sensitivity: Different steel compositions respond variably to notches, highlighting how certain grades exhibit superior toughness, which is crucial for specific engineering applications.
Discussion of Findings
The implications of these findings resonate profoundly in industries where steel is integral, such as construction and automotive. When choosing materials subjected to sudden forces, understanding the energy absorption characteristics is vital.
"Steel that possesses high impact energy values reveals its potential to prevent catastrophic failures in critical components."
These insights show how adhering to current industry standards can enhance safety and performance. The evolution of steel grades, marked by targeted enhancements in ductility, enables engineers to tailor material choices to project requirements effectively.
Methodology
Research Design
The Charpy impact test methodology involves specific procedures to ensure consistency and reliability of results. A standardized specimen of steel, often featuring a V-notch, is subjected to a controlled impact via a swinging pendulum.
Data Collection Methods
Data collection involves:
- Standardized Testing Conditions: Each test is performed under the same environmental conditions, accounting for factors such as temperature and humidity.
- Replicates: Multiple samples of the same steel grade are tested to obtain an average energy absorption value. This enhances the robustness of the findings.
- Statistical Analyses: Employing statistical techniques allows researchers to discern patterns and significance within the collected data, ensuring that the conclusions drawn are not based on isolated tests but reflective of broader trends.
Through this rigorous methodology, the Charpy impact test continues to be a reliable indicator of steel performance, vital for assessing safety and durability in engineering applications.
Foreword to the Charpy Impact Test
The Charpy impact test is a critical method used to evaluate the toughness of materials, particularly steel. This test measures the energy absorbed by a material during fracture when subjected to a sudden impact load. Understanding the results of this test is essential for engineers and researchers, as it provides valuable insights into material performance under dynamic loading conditions. By selecting appropriate materials based on their toughness, professionals can enhance safety and reliability in structures and components.
Defining the Charpy Impact Test
The Charpy impact test involves striking a notched specimen with a swinging hammer and measuring the energy absorbed during fracture. The test is conducted on a standardized sample, typically in the shape of a beam with a specific notch configuration. The absorbed energy is quantified in joules, and this value serves as a vital indicator of the material's ability to withstand impact stresses.
In practical terms, a higher energy absorption value implies better toughness. This makes the Charpy test a crucial factor in material selection for applications that require resilience, such as in construction, automotive, and aerospace industries. The test's simplicity and effectiveness have made it a widely accepted standard across various sectors.
Historical Context and Development
The Charpy impact test was developed by French engineer Georges Charpy in the early 20th century. Initially designed to improve assessment methods for rail and bridge materials, it became essential for understanding steel's behavior under low-temperature conditions and other critical environments. Over the years, as industries evolved, the test adapted to include variations that account for diverse material characteristics.
As steel usage expanded, so did the relevance of understanding its ductility and toughness. The Charpy test emerged as a pivotal evaluation method that could assist in preventing catastrophic failures. Through advancements in testing techniques and a growing understanding of material science, the Charpy impact test has continually refined its role within engineering. The historical development of this test underlines its significance in promoting safety and efficiency in various applications.
Fundamentals of Steel Material Properties
Understanding the fundamental properties of steel is essential for interpreting Charpy impact test results. These properties not only guide engineers and researchers but also influence the choices made during material selection for various applications. The relationship between the mechanical properties of steel and their performance in real-world situations cannot be overstated. Grasping these concepts helps in ensuring that the steel components can withstand operational stresses while exhibiting desired toughness and ductility in various environments.
Mechanical Properties of Steel
The mechanical properties of steel encompass a variety of factors, such as strength, ductility, hardness, and toughness. Each of these elements plays a critical role in determining how steel will behave under different mechanical stresses.
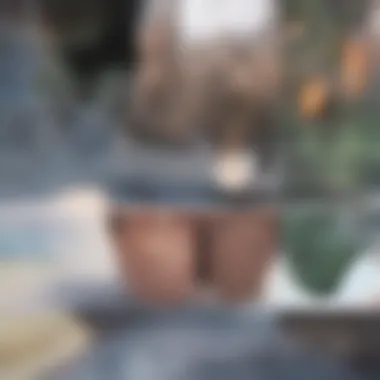
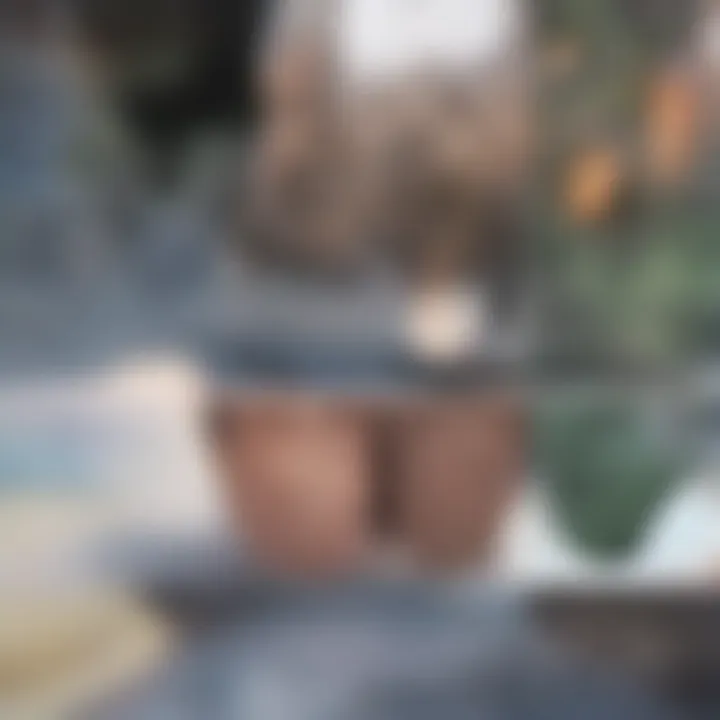
- Strength refers to the material’s ability to withstand forces without failing. It can be categorized into yield strength and tensile strength.
- Ductility indicates how much deformation the steel can undergo before fracture. This property is vital for applications where materials must be formed or shaped.
- Hardness measures the resistance to surface deformation and is indicative of wear resistance.
- Toughness is particularly critical in the context of the Charpy impact test. It denotes the material’s ability to absorb energy without fracturing, an essential characteristic for components subjected to dynamic loading.
When steel has desirable mechanical properties, it performs better in applications ranging from construction to automotive and aerospace engineering. Understanding these mechanical properties is therefore crucial for predicting how steel will respond under specific conditions.
Importance of Toughness in Steel
Toughness is an indispensable property in determining the reliability of steel components in applications where impacts or shocks can occur. In context with the Charpy impact test, toughness indicates how well a material can endure sudden forces. The test itself measures the energy absorbed by a steel specimen during fracture when struck by a hammer-like pendulum. Therefore, higher toughness levels often correlate with better performance in demanding environments.
Some key aspects regarding toughness include:
- Temperature Sensitivity: Toughness can greatly vary with temperature changes. Steel may become brittle at low temperatures, showing a significant drop in toughness.
- Microstructure Influence: The microstructural arrangements of steel, including phases such as martensite, ferrite, and pearlite, impact toughness significantly. Optimizing these structures leads to improved energy absorption in the face of dynamic loads.
- Implications for Safety: In structural applications, selecting steel with adequate toughness ensures the safety and longevity of structures. Understanding this property is paramount for preventing catastrophic failures.
Given the challenges in modern engineering, the emphasis on toughness has led to innovative processing techniques and alloy developments that can enhance this property. Thus, the significance of toughness in steel cannot be overlooked, especially regarding its role in the overall performance and reliability of materials in high-stress environments.
The Methodology of the Charpy Impact Test
The methodology of the Charpy impact test is fundamental to understanding the toughness and ductility of steel. It serves as a critical framework within material science and engineering. The results derived from this test provide vital information about how steel performs under sudden stress or impact. This section discusses essential elements of the methodology, emphasizing its benefits and considerations to ensure reliable outcomes in testing.
Setup and Equipment Required
To conduct the Charpy impact test, specific equipment and setup are necessary. The test typically requires:
- Charpy testing machine: This machine contains a pendulum that swings to strike a notched specimen.
- Specimen preparation tools: Tools for cutting, notching, and polishing the steel samples are vital for accurate results.
- Temperature control apparatus: Since the impact resistance of steel varies with temperature, it's important to regulate the test environment. This can include ovens or cooling systems.
The proper calibration of the machine is equally important. Any deviation might give erroneous results, so accuracy in setup cannot be overstated.
Testing Procedure Outline
The testing procedure involves several crucial steps:
- Specimen Selection: Ensure the samples are representative of the batch being tested.
- Notching: Each steel specimen must have a standardized notch. The notch's location and dimensions can significantly influence the results.
- Temperature Conditioning: The specimens should be conditioned at the specified temperature to observe how different temperatures affect the impact energy.
- Impact Testing: The pendulum swings and strikes the specimen. The energy absorbed by the sample is measured. This energy is a key indicator of toughness.
- Data Recording: Record the energy values for analysis. It's advisable to conduct multiple tests for statistical reliability.
Sample Preparation Techniques
Sample preparation is critical for ensuring valid results. Techniques include:
- Cutting: Ensure uniformity in specimen size, adhering to testing standards.
- Notching: This must be done precisely to avoid introducing variables. The notch should be deep enough to create a stress concentration without weakening the overall integrity of the specimen.
- Polishing: Proper polishing eliminates surface imperfections which could affect fracture behavior. While this step can be overlooked, it is crucial for precise testing.
Attention to detail in these techniques reflects significantly on the reproducibility and reliability of the test results.
"Reliable Charpy impact test results rely on thorough preparation and methodical execution of established procedures."
Interpreting Charpy Impact Test Results
Interpreting the results of the Charpy impact test is crucial for material assessment, especially in engineering contexts. Understanding these results allows researchers and professionals to determine the ductility and toughness of steel under impact conditions. This knowledge can guide material selection for various applications, influencing safety and performance standards. A thorough interpretation considers not only the numerical values but also the physical implications of the observed results.
Understanding Impact Energy Values
The impact energy value derived from the Charpy test serves as a key indicator of material performance. This value, measured in Joules, reflects the amount of energy absorbed by the material before fracture occurs. High impact energy values typically indicate greater toughness, suggesting that the material can withstand sudden forces or impacts without failing. Conversely, low energy values may signal brittleness, which can lead to catastrophic failures in critical applications.
- Materials with high toughness often show good ductility, meaning they can deform significantly before rupturing.
- Low toughness materials might exhibit little deformation under stress, risking fracture without warning.
Using these energy values effectively involves comparing them to standardized benchmarks. This comparison can aid in predicting how a specific steel might perform in real-world scenarios. Additionally, it can facilitate decisions regarding material treatment processes and design adjustments.
Fracture Surfaces Analysis
Analyzing the fracture surfaces resulted from Charpy tests provides valuable insight into the failure mechanisms of materials. The appearance of the fracture surface can tell whether the material failed by ductile or brittle means.
- Ductile fractures typically show characteristics like a fibrous appearance and dimples, indicating absorbing considerable energy before breaking.
- Brittle fractures, on the other hand, present smooth, shiny surfaces, revealing minimal energy absorption.
Understanding these fracture patterns is essential. They can inform whether further altering of material composition or processing might be necessary to enhance toughness and prevent unexpected failures in service. The adoption of high-resolution imaging techniques, such as scanning electron microscopy, can significantly enhance the analysis of fracture surfaces.
Impact of Temperature on Results
Temperature is a critical factor affecting the results of the Charpy impact test. Changes in temperature can significantly influence material properties, particularly for steels. Lower temperatures often lead to increased brittleness, reducing impact energy values. This trend is especially relevant when considering applications in cold environments.

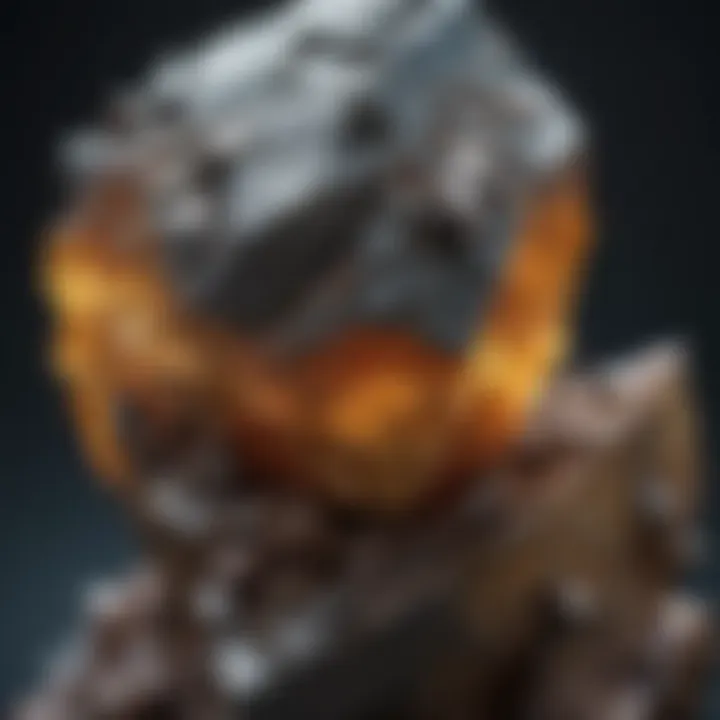
At elevated temperatures, materials may display improved toughness, leading to higher energy absorption values. However, it is important to find an optimal temperature range for specific applications to maximize safety and performance.
- Cold regions: Materials must be tested at real operating temperatures to ensure performance.
- Hot environments: Increased temperatures may alter mechanical properties, so testing under expected conditions is necessary.
Understanding the influence of temperature on Charpy test results helps engineers tailor materials for specific environments, ensuring reliability and safety in engineering applications.
Factors Influencing Charpy Impact Test Outcomes
The Charpy impact test evaluates a material's toughness, which directly relates to its performance in practical applications. Several factors greatly influence the outcomes of this test. Understanding these factors can help engineers and researchers select the right materials for specific tasks and understand the implications for structural integrity.
Influence of Chemical Composition
The chemical composition of steel plays a critical role in its toughness and ductility. Different elements can modify the properties of steel significantly. For example:
- Carbon content: Increased levels usually enhance hardness but can decrease toughness. This balance must be managed effectively for applications requiring both strength and flexibility.
- Alloying elements: Elements such as manganese, nickel, and chromium can improve toughness at low temperatures. These alloying elements influence how steel behaves under stress, especially when sudden forces are applied.
Moreover, trace elements present during melting and refining processes can also affect test results. Impurities such as sulfur and phosphorous can embrittle steel, which will lead to lower impact energy readings. Understanding the chemical makeup, therefore, is essential for interpreting Charpy test results effectively.
Impact of Microstructure
Steel's microstructure is pivotal in determining toughness as well. The arrangements and phases present at the microscopic level significantly affect material behavior. Here are key aspects to consider:
- Grain size: Finer grains typically promote better toughness. Smaller grains mean more surfaces for dislocations to travel through, which can absorb more energy during impact.
- Phase transformations: The transformation between phases like austenite, martensite, and pearlite can change the mechanical properties. For instance, martensite provides high strength but often lacks toughness unless tempered properly.
- Presence of inclusions: Non-metallic inclusions can act as stress concentrators, influencing how a material fractures. The distribution and type of these inclusions can directly affect the results from the Charpy test.
Evaluating microstructure influences the expected outcomes. Analyzing samples using techniques such as scanning electron microscopy can uncover insights related to toughness.
Role of Processing Techniques
The processes used to manufacture steel also greatly impact its performance in the Charpy test. Here are some crucial techniques and their implications:
- Heat treatment: Processes like quenching and tempering can alter steel’s microstructure effectively, improving toughness. Properly executed heat treatments can yield materials with optimal performance under dynamic loading
- Welding and fabrication methods: Different welding techniques affect the heat-affected zones of steel components. Incomplete fusion or improper cooling can introduce defects that impact mechanical properties. Understanding these variables is critical for ensuring that welded structures meet safety and performance standards
- Cold working: This process can increase the strength of steel, but it often reduces ductility. Engineering applications requiring both properties must find a balance through controlled processing.
Understanding how these processing techniques affect steel's response allows for better predictions of safety and reliability in engineering applications.
Applications of Charpy Impact Test Results
The applications of the Charpy impact test results extend well beyond mere academic interest; they play a significant role in practical engineering and construction scenarios. This section analyses how these insights influence material selection and safety considerations, underscoring their importance in the overall design and assessment of structures and components.
Material Selection in Engineering
Charpy impact test results guide engineers in selecting materials for various applications. Understanding a material’s toughness helps in identifying its suitability for specific conditions. For instance, in sectors where impact loading is common, such as aerospace and automotive industries, steel with high impact energy absorption is preferable.
Engineers often compare Charpy test results with the operational environment of a material. A material that performs well at room temperature might not be suitable for low-temperature applications. This leads to careful consideration of the chemical composition. Steels with controlled microstructures often demonstrate better toughness, as seen in normalized high-strength steels.
- Benefits of Using Charpy Results for Material Selection:
- Ensures reliability in high-stress applications.
- Reduces risk of catastrophic failure in structural components.
- Facilitates adherence to regulatory standards in various industries.
Safety Assessments in Structures
The importance of Charpy impact test results cannot be overstated when it comes to safety assessments in structures. These tests can reveal not just how a material reacts under extreme conditions but also assist in predicting performance during various stages of its lifecycle. By understanding the toughness characteristics, engineers can make informed decisions regarding the design and material usage in critical structures.
- Considerations for Safety Assessments:
- Identifying potential failure modes under impact conditions.
- Evaluating the correlation between toughness and expected service life.
- Justifying necessary safety margins in structural designs.
The Charpy impact test serves as a fundamental predictive tool for assessing material behavior in real-world conditions.
In summary, the application of Charpy impact test results is paramount in informing material selection and enhancing safety in engineering practice. These results have become integral to maintaining structural integrity and performance in various fields, making their understanding essential for students, researchers, and professionals alike.
Challenges in Charpy Impact Testing
The Charpy impact test is a vital procedure for assessing the durability of steel and its ability to withstand sudden impacts. However, this process is not free of challenges. Engaging with these challenges offers significant insights into the reliability of test results and the subsequent interpretation of data. With a clearer understanding of these issues, professionals can make informed decisions regarding material selection and application.
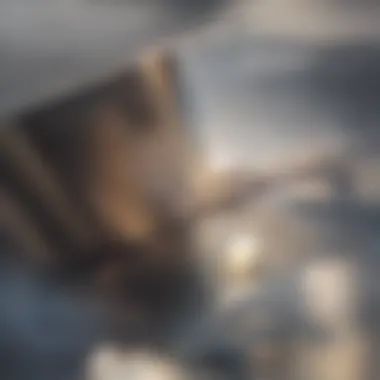
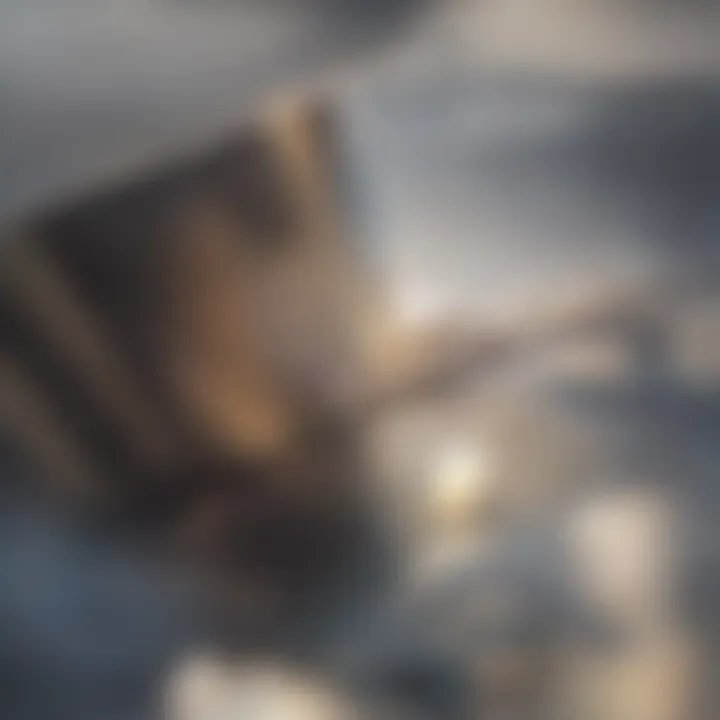
Variability in Test Results
One primary challenge encountered in Charpy impact testing is the variability in test results. This variability can arise from various factors, including environmental conditions, sample preparation, and variations in steel composition.
Environmental conditions such as temperature and humidity can significantly affect the test outcomes. Specifically, the temperature at which the test is conducted plays a critical role. Steel may exhibit different toughness characteristics at various temperatures, potentially leading to inconsistent impact energy values.
Moreover, sample preparation techniques can introduce additional variability. For instance, the notched samples must be prepared with precision, as even minor irregularities can affect the fracture behavior under impact. Small differences in notch depth, angle, or surface finish can result in substantial fluctuations in measured impact energy.
Finally, differences in steel composition, such as variations in alloying elements, can also impact results. Alloys can produce different microstructures, which influence both the toughness and the mechanical properties of the material. This complexity highlights the need for strict controls in both sample preparation and testing conditions to minimize variability and enhance the reliability of test outcomes.
Limitations of the Charpy Test
While the Charpy impact test is widely utilized, it has inherent limitations that must be considered. One significant limitation is that it evaluates only a small volume of material, which may not necessarily represent the bulk properties of a larger structure. Therefore, the test may overlook potential defects that exist outside the tested region.
Additionally, the Charpy test is primarily designed for quantifying the ductile-brittle transition in materials at a particular temperature. For materials that exhibit non-linear behavior or complicated fracture processes, the results may not provide a comprehensive picture of material performance under real-world conditions.
Another limitation lies in its inability to assess the impact of stress conditions that materials may face during service. Real-world applications can involve complex stress states, including multi-axial loading and the presence of pre-existing defects. These factors are not replicated in the Charpy test, thus necessitating complementary testing methods to evaluate material performance accurately.
In summary, while the Charpy impact test remains a cornerstone for evaluating steel toughness, addressing its inherent challenges and limitations is crucial. Understanding variability in results and recognizing the constraints of the test can inform further research and enhance future testing methodologies.
Future Directions in Impact Testing
As the field of materials science continues to evolve, understanding the future directions in impact testing is crucial for advancing the practical applications of the Charpy impact test. These advancements are significant not only for improving testing accuracy but also for optimizing material selection in engineering applications. Recognizing the importance of modernizing testing techniques is essential for aligning with industry standards and pushing the boundaries of current knowledge.
Advancements in Testing Techniques
Recent years have seen notable improvements in Charpy testing methodologies. Traditional techniques, while effective, can provide limited data. Innovations such as digital imaging and automated testing equipment have emerged. These methods allow for more precise measurements of impact energy and fracture characteristics.
The use of high-speed cameras enhances the ability to analyze fracture behavior in real-time. This technology provides insights into the dynamic response of materials under impact, offering a deeper understanding of how different steels perform. Furthermore, advancements in materials technology allow for the development of specialized test specimens, which can yield more representative results when evaluating steel performance under real-world conditions.
Importantly, the movement toward standardization in testing protocols ensures consistency across different laboratories and settings. This change not only harmonizes results but also elevates reliability in industrial applications.
Integration with Computational Models
The integration of Charpy impact testing with computational models represents a paradigm shift in material testing. Computational models allow researchers and engineers to simulate material responses before physical tests. This approach helps in predicting how specific steel grades will behave under varying conditions.
Using finite element analysis and other simulation techniques, it is now possible to visualize stress distributions and predict failure points in materials. Such models can be validated against experimental data obtained from Charpy tests, creating a feedback loop that enhances both the predictive capability of the models and the understanding of material behavior.
Additionally, machine learning and artificial intelligence are entering the field. These technologies can analyze vast amounts of testing data quickly, identifying trends and correlations that were previously undetectable. By leveraging these advancements, researchers can refine their testing strategies and material selection processes, leading to significantly improved designs in engineering projects.
The move toward advanced testing techniques and computational integration marks a critical evolution in impact testing, ensuring a more comprehensive understanding of material properties.
In summary, the future of impact testing lies in embracing technological advancements and integrating sophisticated computational models. This shift not only enhances the precision of Charpy impact results but also informs better decision-making in the selection and application of materials across various industries.
Ending: Implications of Charpy Impact Test Results
The evaluation of Charpy impact test results has essential implications for material science, especially when dealing with steel. Understanding these results begins with recognizing their role in assessing the toughness and ductility of steel. The outcomes of such tests inform engineers and researchers about the material performance under scenarios involving sudden impacts or shocks. This knowledge is paramount, enabling informed decisions that ensure safety and reliability in engineering applications.
In practical contexts, the results can directly influence material selection for various structural components. Components subjected to dynamic loads, such as bridges and buildings, must possess adequate toughness to withstand unexpected stresses. Thus, the reliable interpretation of Charpy results aids in predicting the behavior of materials in real-world conditions, contributing to enhanced safety margins in structural design.
Additionally, understanding the implications of these test results fosters advancements in the metallurgical field. By analyzing how different factors—like chemical composition, microstructure, and processing techniques—affect impact energy measurements, researchers can develop new alloys and treatments that optimize performance. This iterative learning process is essential for the evolution of materials used in critical infrastructures.
"Charpy impact test results are not merely numbers; they are indicators of the steel's ability to perform under stress. This makes them significant for engineers and designers alike."
Final Thoughts on Interpretation
Interpreting Charpy impact test results requires both technical knowledge and practical experience. The raw data derived from these tests must be placed in context before any conclusions can be drawn. Factors such as temperature conditions during testing, sample geometry, and previous treatments of the steel can substantially affect the results. Therefore, it is vital to analyze results in tandem with these parameters to form a robust interpretation.
Furthermore, fractured surface analysis offers critical insight. Distinct patterns can reveal the mechanism of fracture, whether it is ductile, brittle, or a mixture of both. This understanding can indicate whether the material will behave as expected in application scenarios.
To promote accurate understanding and interpretation of results, educators and professionals should advocate for a continuous learning approach. This includes staying updated with the latest testing techniques and subsequent research findings in material sciences.
Recommendations for Future Research
Future research on Charpy impact testing should concentrate on several pivotal aspects. One recommendation is the exploration of advanced testing methodologies that can provide more nuanced insights into material toughness. New techniques, perhaps using digital imaging or machine learning algorithms, could improve upon traditional interpretations significantly.
Additionally, investigations into how recent developments in steel alloys influence impact toughness will be beneficial. Materials scientists should explore the interaction of different alloying elements and their distinct contributions to toughness, with a focus on optimizing these combinations for superior performance.
Lastly, naturally, interdisciplinary collaboration is essential. Bridging the gap between theoretical research and practical applications can lead to innovations in both testing methodologies and material improvements. Partnerships among academicians, manufacturers, and regulatory bodies can foster environments for more extensive data sharing and research collaboration.
Adopting these recommendations will not only improve the understanding of Charpy impact test results but also contribute to the overall progress of material sciences, ensuring developments keep pace with industry demands.