Understanding Photosensitive Diodes and Their Innovations
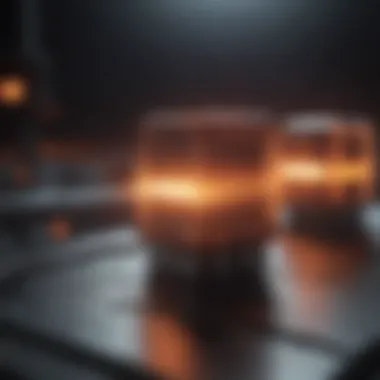
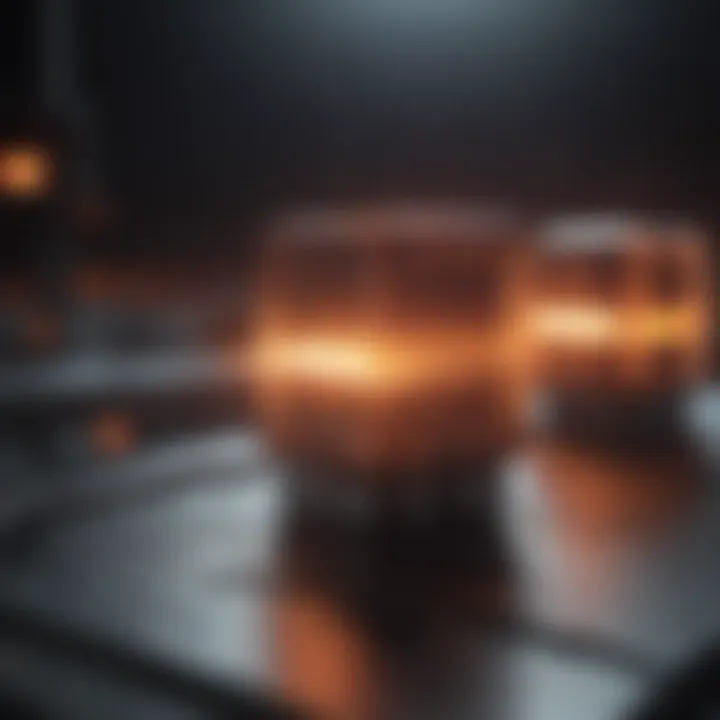
Intro
Photosensitive diodes are critical components in modern electronic systems. Their ability to convert light signals into electrical currents positions them as key players in a variety of applications. Understanding how these devices work, their design variations, and their role in technological advancements is essential for students, researchers, educators, and professionals alike.
This article will analyze the principles behind photosensitive diodes, focusing on their operational mechanisms and how they respond to light. We will also explore their significant applications in fields such as communication, sensing technologies, and medicine. In addition, the discussion will highlight recent innovations that are shaping the future of photosensitive diode technology.
To grasp the importance of photosensitive diodes, one must first examine their core operating principles. This foundation will grant insight into their applications and further innovations, making it central to a comprehensive understanding of this technology.
Foreword to Photosensitive Diodes
Photosensitive diodes play a critical role in the contemporary electronic landscape. They are devices that convert light into electrical signals, making them essential in various industries. Their significance extends to communication, sensing technologies, and medical applications. A solid understanding of their principles and functionalities enables engineers and researchers to innovate and improve existing systems. In this article, we will explore the fundamental aspects, historical context, and broader implications of photosensitive diodes.
Definition and Functionality
A photosensitive diode, often referred to as a photodiode, is a semiconductor device designed to detect light. It operates by converting incoming photons into an electrical current. When light strikes the diode, it excites electrons, generating pairs of electrons and holes. This excitation leads to a measurable current. The functionality of these devices can vary based on their construction and intended application.
There are several methods used to enhance the performance of photodiodes. Factors like material choice, impurity concentration, and geometry all influence their efficiency. Some common types include silicon photodiodes, which are widely used due to their suitable properties, and gallium arsenide photodiodes, known for their sensitivity to infrared light. This variety allows for applications across different fields.
Historical Development
The development of photosensitive diodes can be traced back to the mid-20th century. Early devices emerged from advancements in semiconductor technology. Initially, these diodes were sourced from materials with limited efficiency. Over time, scientists explored various semiconductor compounds, like germanium and silicon, leading to significant improvements.
The introduction of silicon as a primary material marked a turning point. Siliconβs abundance and desirable electronic properties allowed for mass production. Innovations continued through the 1980s and 1990s, with advances in the understanding of photoconductivity and the integration of technologies like photonic devices.
Today, research into new materials, such as organic semiconductors and new alloys, continues. This exploration aims to enhance efficiency and broaden the applications of photosensitive diodes. Importantly, the cycling between theory and practical implementation has led to breakthroughs that underpin many modern technologies.
"Understanding the evolution of photosensitive diodes is essential for grasping their current applications and future potential."
Fundamental Principles of Operation
Understanding the fundamental principles of operation is critical for grasping how photosensitive diodes work. This section outlines the basic mechanisms that drive the functionality of these devices. Comprehension here leads to innovation and application in various fields such as telecommunications, medical technology, and environmental monitoring. A deep dive into these principles reveals insights about their design, performance, and potential improvements.
Photoconductivity Explained
Photoconductivity refers to the increase in electrical conductivity of a material when exposed to light. In photosensitive diodes, this phenomenon is crucial. When photons strike the diode, they can energize electrons, allowing them to move freely and create an electrical current. Each type of photosensitive diode, whether it is silicon, cadmium sulfide, or gallium arsenide, utilizes this principle differently, though the core concept remains the same.
This characteristic is particularly valuable in applications requiring light detection, such as in light sensors or cameras. The efficiency and responsiveness of a diode are significantly influenced by its photoconductivity. When designing or selecting a photosensitive diode, considerations about its photoconductivity characteristics can greatly affect the overall performance in a system.
Energy Band Theory
Energy band theory provides insights into how materials behave at the atomic level. In semiconductors, energy bands represent the range of energies that electrons can possess. The conduction band contains free electrons, while the valence band contains bound electrons. The gap between these bands is known as the band gap.
When light hits the semiconductor, photons can supply sufficient energy to bridge this gap, promoting electrons from the valence band to the conduction band. This transition allows for the flow of current that the device will produce in response to light. The bandgap material type determines the wavelength of light the diode reacts to, influencing its spectral sensitivity.
Photovoltaic Effect
The photovoltaic effect is essential for converting light into electrical energy. This effect occurs in photodiodes when light photons knock electrons out of their atomic bonds within the semiconductor material. The result is an electric charge that can be captured and utilized. This process underpins solar cells but is also vital in photodiodes used in various applications.
In terms of efficiency, the photovoltaic effect is influenced by numerous factors, including material purity and layer thickness. Devices utilizing the photovoltaic effect can generate voltages even in low light. Thus, understanding this effect improves designs aimed at harvesting energy from ambient light sources.
In summary, the fundamental principles of operation in photosensitive diodes encompass critical concepts like photoconductivity, energy band theory, and the photovoltaic effect. These principles are essential for comprehending how these devices function in practical applications.
The integration of these principles not only aids in further innovations but also enhances the understanding of their current capabilities in technology.
Types of Photosensitive Diodes
Understanding the different types of photosensitive diodes is essential in grasping how these devices function in various applications. Each type comes with unique characteristics and advantages, making them suitable for specific contexts. The selection of a particular type can significantly influence the performance and efficiency in applications ranging from consumer electronics to industrial sensors and communication systems.
Silicon Photodiodes
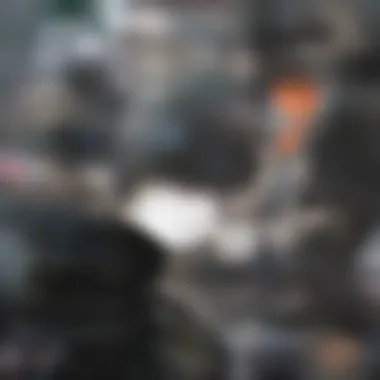
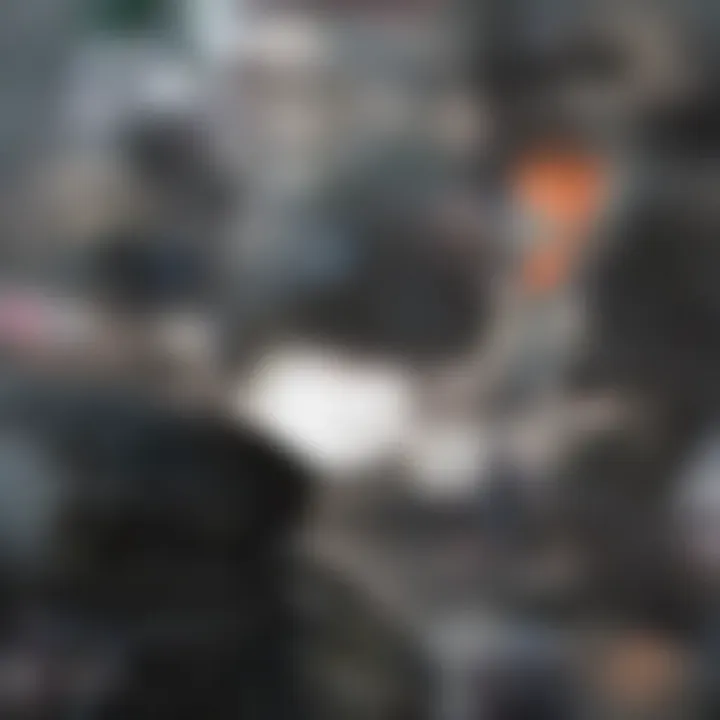
Silicon photodiodes are among the most common types of photosensitive diodes. They utilize silicon, a semiconductor material, which offers excellent photoconductivity. These diodes operate effectively in the visible to near-infrared spectrum, which allows them to respond well to various light sources.
Benefits of Silicon Photodiodes:
- Cost-Effective: Silicon is widely available and relatively inexpensive compared to other semiconductor materials.
- Stability: They provide stable performance under a range of environmental conditions, making them a reliable choice in many applications.
- Versatile Applications: Commonly used in optical communication, imaging systems, and consumer electronics.
Cadmium Sulfide Sensors
Cadmium sulfide sensors, or CdS cells, are another type of photosensitive diode. These sensors are particularly sensitive to light and primarily operate in the visible spectrum. They are known for their ability to detect low light levels, which makes them useful in various sensing applications.
Key Features of Cadmium Sulfide Sensors:
- High Sensitivity: They can respond to very low levels of illumination, making them excellent for light-sensing applications.
- Inexpensive Manufacturing: The production process of CdS sensors is generally less complex and more cost-effective than some alternatives.
- Applications: Often used in photometers, light meters, and automatic lighting systems.
Gallium Arsenide Photodiodes
Gallium arsenide photodiodes, made from the compound semiconductor gallium arsenide, exhibit superior performance in certain conditions. They are particularly efficient in converting light to electrical signals and are used in high-frequency applications.
Advantages of Gallium Arsenide Photodiodes:
- Higher Efficiency: They can achieve higher efficiencies compared to silicon photodiodes, especially in the infrared region.
- Fast Response Time: Their construction allows for a quicker response to light changes, which is crucial in optical communication.
- Robustness: Proven to perform well in high-temperature environments, adding to their versatility in demanding applications.
Understanding these distinct types of photosensitive diodes helps in identifying their appropriate use cases effectively.
Key Characteristics of Photosensitive Diodes
Understanding the key characteristics of photosensitive diodes is crucial for grasping their effectiveness and capabilities. These characteristics not only determine how well a diode performs under varying conditions but also influence its application across different technological fields. In this section, we will cover three main characteristics: spectral sensitivity, response time, and noise characteristics. Each factor plays a vital role in optimizing the technology for specific uses, impacting sectors from communications to medical devices.
Spectral Sensitivity
Spectral sensitivity refers to the responsiveness of a photosensitive diode to different wavelengths of light. Each specific diode is designed to have a particular range of sensitivity, which is vital for applications requiring precise light detection. For instance, silicon photodiodes typically exhibit good sensitivity in the visible spectrum, while gallium arsenide photodiodes can be designed to detect near-infrared light.
The significance of spectral sensitivity cannot be overstated. It affects not only the efficiency of light detection but also dictates which applications a specific type of diode is suited for. Consequently, engineers must consider the spectral sensitivity of a diode when developing systems for optical communications or imaging applications, ensuring the device captures light effectively for intended processes.
Response Time
Response time is another vital characteristic which defines how quickly a photosensitive diode can react to changes in light intensity. Fast response times are particularly important in applications that require real-time data processing, such as in digital cameras or high-speed communication systems. In general, materials and structural designs contribute greatly to the response time.
For example, photodiodes made from gallium arsenide tend to have faster response times compared to their silicon counterparts. This finite responsiveness influences the overall performance of devices utilizing these diodes, especially in environments with rapidly fluctuating light conditions. Engineers must evaluate the required speed based on the application to select the appropriate photodiode.
Noise Characteristics
Noise characteristics encompass the unwanted signals that interfere with the true responses of photosensitive diodes. Noise can originate from a variety of sources, including thermal fluctuations, electronic interference, and even the inherent properties of the materials used. Understanding the noise characteristics is essential to improve the signal-to-noise ratio in practical applications.
Effective noise reduction techniques can enhance a diode's performance significantly. Designers often implement various strategies like cooling methods or shielding to minimize these undesirable effects. High-performance systems, like those used in medical diagnostics or environmental monitoring, demand careful attention to noise characteristics to ensure they provide accurate readings.
The interplay of spectral sensitivity, response time, and noise characteristics fundamentally dictates the effectiveness of photosensitive diodes across various fields. Being aware of these attributes enables better optimization of the devices for specific tasks while paving advancements in technology.
Applications of Photosensitive Diodes
Photosensitive diodes bear significant importance across diverse applications, shaping how devices interact with light. Their ability to convert light into electrical signals makes them invaluable in communication and monitoring systems. This section will explore key applications in various fields, highlighting their individual contributions and relevance.
Optical Communication Systems
Photosensitive diodes play a critical role in optical communication systems. The primary function is to detect light pulses and convert them into electrical signals. This process is essential for transmitting information over long distances, such as in fiber optic networks.
The use of silicon photodiodes allows for high-speed data transmission with minimal signal loss. These diodes are sensitive to various wavelengths, enhancing their applicability across different communication technologies. Additionally, the integration of advanced materials, like indium gallium arsenide, increases efficiency significantly. This ensures that communications remain clear and uninterrupted.
In the realm of optical communications, one aspect to consider is the response time of these diodes. Faster response times mean quicker data relay, which is crucial for high-bandwidth applications, including internet services and telecommunication.
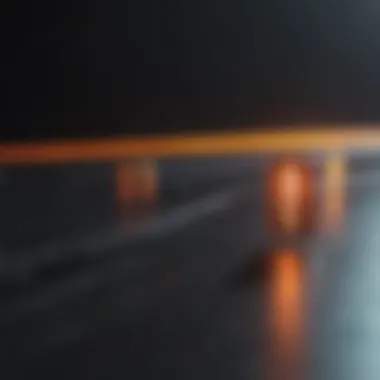
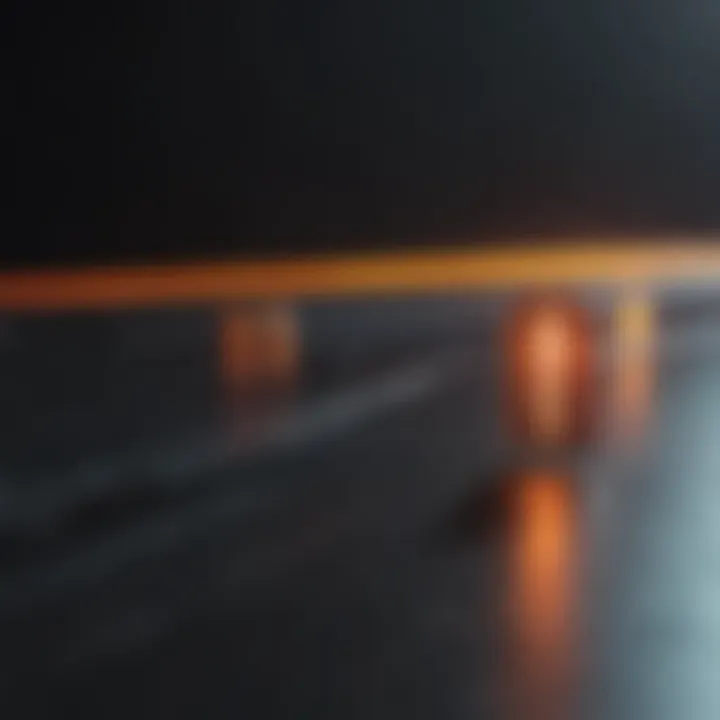
Sensing Technologies
In sensing technologies, photosensitive diodes are widely used to detect light levels and variations. This application spans diverse industries, including automotive, consumer electronics, and security. For instance, in automotive systems, photodiodes detect ambient light, allowing for automatic adjustments in headlights.
Particularly, cadmium sulfide sensors are known for their efficiency in light sensing applications like light meters and security alarms. These sensors respond to changes in luminosity, making them useful in providing feedback in automated systems. Their ability to operate efficiently in varying conditions fortifies their relevance in modern sensing strategies.
The consideration of noise characteristics also plays a vital role in this application. Effective noise reduction techniques enhance the accuracy of signal detection, thus improving overall sensor reliability.
Medical Devices
Photosensitive diodes find extensive use in medical devices, significantly enhancing diagnostic capabilities. They are used in numerous devices, such as pulse oximeters and phototherapy equipment. In pulse oximeters, for instance, they detect the amount of oxygen in blood by measuring the light absorption at specific wavelengths.
Gallium arsenide photodiodes are favored in certain applications due to their higher efficiency and sensitivity compared to silicon alternatives. This higher sensitivity allows for more precise readings, which can be vital in patient monitoring.
As the medical field continually evolves, the need for innovative solutions rises. Integrating photosensitive diodes into devices paves the way for better health outcomes through accurate diagnostics and treatment options.
Environmental Monitoring
Environmental monitoring is another key application of photosensitive diodes. These devices are instrumental in analyzing air quality and detecting pollutants. By converting light levels into measurable electronic signals, they facilitate an understanding of environmental conditions. This can involve monitoring light absorption by atmospheric components, enabling the assessment of air quality indices.
In this context, emerging materials are gaining traction. Novel materials can improve sensitivity to a wider range of light, enhancing the capability of sensors in various environmental conditions. Furthermore, the data collected can lead to informed decisions regarding public health and safety measures.
In summary, the versatility of photosensitive diodes across applications like optical communication systems, sensing technologies, medical devices, and environmental monitoring highlights their essential role in modern technology. Each application underscores the unique characteristics and innovations contributing to advancements in several fields.
Innovations in Photosensitive Diode Technology
Innovations in photosensitive diode technology are driving significant advancements in various fields. These emerging developments are not only enhancing the performance of existing devices but also redefining their applications. As the demand for more efficient and effective sensors grows, understanding these trends becomes paramount for students, researchers, educators, and professionals.
Emerging Materials
The exploration of new materials in the development of photosensitive diodes is crucial. Traditional materials like silicon and germanium have dominated the market for years. However, researchers are now investigating alternative materials that offer better sensitivity and efficiency. These include organic semiconductors, perovskites, and 2D materials like graphene.
Emerging materials provide several benefits:
- Improved Efficiency: Many new materials can absorb a wider spectrum of light, leading to higher conversion efficiencies.
- Cost-effectiveness: Materials like organic semiconductors can be produced at a lower cost than silicon-based diodes.
- Flexibility: New materials often allow for flexible designs, opening doors to applications in wearables and other portable devices.
Miniaturization Trends
The trend towards miniaturization in technology is evident in photosensitive diodes as well. As devices become smaller, the need for compact and efficient sensors grows. This trend supports easier integration into varied systems without compromising on performance.
Miniaturization presents some key considerations:
- Size vs. Performance: Engineers are challenged to maintain or even enhance performance while reducing size.
- Thermal Management: Smaller components can lead to overheating, so effective heat dissipation methods are necessary.
- Manufacturing Complexity: Smaller designs could complicate the manufacturing process, requiring new precision techniques.
Integration with Other Technologies
Integrating photosensitive diodes with other technologies offers transformative potential. This integration allows for the creation of more sophisticated systems that leverage the capabilities of multiple technologies.
Integration aspects include:
- Smart Systems: Photosensitive diodes can be combined with AI algorithms to create smart sensors that adapt based on environmental conditions.
- IoT Applications: Their integration within Internet of Things devices enhances functionality, enabling remote monitoring and control.
- Energy Harvesting: When combined with photovoltaic technologies, they can help create energy-efficient systems that capture and utilize light energy effectively.
"The seamless integration of advanced materials and technologies is reshaping the landscape of photosensitive diode applications."
Challenges in Photosensitive Diode Development
The technology behind photosensitive diodes continues to evolve, yet several challenges persist. These challenges simply reflect the intricate nature of these devices and their importance in both fundamental research and real-world applications. Addressing these challenges is crucial to enhance performance, reliability, and efficiency of photosensitive diodes. This section dives into three significant challenges: noise reduction techniques, temperature effects, and material limitations.
Noise Reduction Techniques
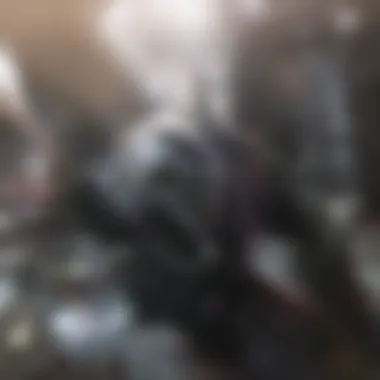
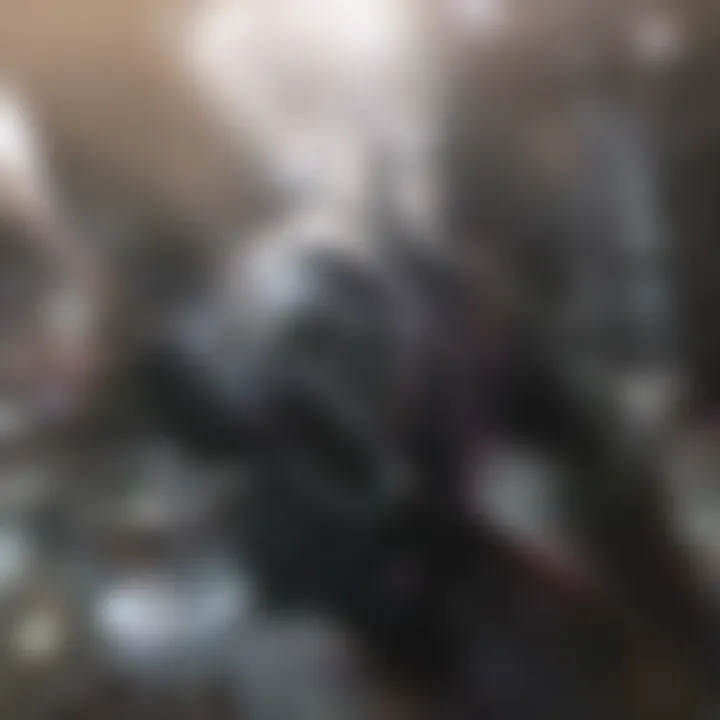
Noise is a perennial challenge in the domain of photosensitive diodes. High levels of noise can overshadow the weak signals that these devices often detect. Therefore, effective noise reduction techniques become essential to improve device performance. Techniques like temperature stabilization, circuit design improvements, and filtering methods serve to minimize this unwanted interference.
- Temperature Stabilization: As temperatures fluctuate, so does the signal-to-noise ratio. Maintaining a consistent operating temperature aids in reducing thermal noise, which is vital for sensitive applications.
- Circuit Design: Optimizing circuit layouts is a practical approach to enhance noise performance. By utilizing differential amplifiers or employing shielding, designers can substantially reduce noise interference.
- Filtering Methods: Implementing both analog and digital filtering methods assists in ignoring background noise. Techniques such as low-pass filtering are common to ensure that only desired frequencies are amplified.
By focusing on these noise reduction strategies, engineers can enhance the accuracy and reliability of photosensitive diodes.
Temperature Effects
Temperature is a critical factor impacting the operation of photosensitive diodes. Variations in temperature can lead to significant changes in device behavior and output signal. For example, increased temperature can enhance the dark current, which can obscure the detection of actual signals.
Engineers often rely on temperature compensation methods to counteract these effects. For instance, designing circuits that respond to temperature variations ensures stable performance. Moreover, using better insulating materials can help manage thermal fluctuations.
"Understanding how temperature influences a device is crucial to its effective operation."
Material Limitations
Material selection considerably affects the efficiency and performance of photosensitive diodes. Each material type, whether it be silicon, gallium arsenide, or cadmium sulfide, possesses inherent limitations that influence its application.
For instance, while silicon photodiodes provide excellent efficiency in the visible spectrum, they may not perform as well in infrared applications. Similarly, gallium arsenide is useful for high-frequency applications, yet it can be cost-prohibitive. The need for innovative materials continues to grow, driving research into alternatives that could offer better thermal stability, broader spectral sensitivity, and other performance metrics.
Future Prospects of Photosensitive Diodes
In recent years, photosensitive diodes have gained attention within the electronics community for their vital role in advancing various technologies. Understanding their future prospects is essential not only for researchers and developers but also for industries seeking to leverage these devices in real-world applications. The development of photosensitive diodes is closely linked to evolving market trends and potential breakthroughs of new materials and technologies. As the demand for efficient light detection continues to grow, the ability to innovate and adapt in this field becomes increasingly important.
Market Trends
The market for photosensitive diodes is expected to expand significantly in the coming years. Various sectors, such as telecommunications, healthcare, and environmental monitoring, are driving this growth. In telecommunications, for instance, the shift towards fiber optics has significantly increased the demand for high-performance photodiodes. Emerging technologies like Li-Fi, which utilize visible light for data transmission, further bolster this trend.
Another notable trend is the integration of photosensitive diodes with other sensors and technologies. This combination enhances their functionality, making them more versatile across applications. For example, combining photodiodes with temperature sensors allows for precise measurement of environmental conditions. Additionally, there is a demand for improved energy efficiency and miniaturization within electronic devices, which encourages innovation in photosensitive diode technology.
Potential Breakthroughs
As we look towards the future, potential breakthroughs in photosensitive diode technology may revolutionize their applications. Research in new materials, such as organic semiconductors and nanostructured photodetectors, holds great promise. These materials can lead to higher sensitivity and improved response times, which are critical in various applications.
Furthermore, advancements in manufacturing techniques, including 3D printing, could facilitate the creation of more efficient and complex designs. This flexibility may allow for tailored solutions that meet specific industry needs.
Emerging technologies like quantum dot photodiodes could redefine performance standards. With their ability to manipulate light at the nanoscale, these devices may offer new functionalities that surpass current models.
"The potential for innovation in photosensitive diode technology is immense, with broad implications across various industries."
In summary, the future of photosensitive diodes is bright, marked by both growing market demand and promising technological advances. Staying attuned to these trends and innovations will be essential for professionals in the field as they navigate the evolving landscape of this crucial technology.
Ending
The conclusion is a critical element of this article as it encapsulates the entire discussion surrounding photosensitive diodes. It serves to distill the main insights and highlight the overarching importance of these devices in modern technology. Through an exploration of their operational principles, various types, and real-world applications, this article has aimed to provide a thorough understanding of photosensitive diodes.
Summary of Key Insights
In summary, photosensitive diodes are pivotal in multiple technological domains. They utilize the principles of photoconductivity and the photovoltaic effect to convert light into electrical signals. The different types of diodes, such as silicon photodiodes and gallium arsenide photodiodes, come with distinct characteristics that make them suitable for specific applications.
Key insights from the article include:
- Diverse Applications: Photosensitive diodes are vital in optical communication, sensing applications, medical devices, and environmental monitoring. Their ability to convert photons into readable electrical signals ensures their relevance.
- Technological Innovations: Ongoing advancements in materials science and miniaturization enhance the performance and applications of these devices. Emerging materials present new possibilities for energy efficiency and sensitivity.
- Challenges and Opportunities: Despite the many benefits, challenges such as noise reduction and temperature sensitivity persist. Addressing these issues will be crucial to optimizing performance and expanding use cases.
The collective understanding achieved in this article positions readers to appreciate both the historical context and the future potential of photosensitive diodes.
Final Thoughts
Conclusively, a robust understanding of photosensitive diodes cannot be overlooked in an age where light-based technologies are increasingly important. As innovations continue to evolve, the demand for efficient, precise, and miniaturized sensors will only increase.
The future prospects of photosensitive diodes suggest that developments in field applications, from telecommunications to healthcare, have only scratched the surface. As researchers and engineers confront existing challenges, new solutions may emerge, allowing for further integration of these devices into advanced technologies. Therefore, staying informed about trends and innovations is essential for professionals, students, and researchers within relevant fields.
"Understanding the principles and applications of photosensitive diodes will pave the way for future technological advancements that rely on precise light detection and conversion."
In closing, the synthesis of insights from this article serves both as a foundation for further study and a springboard into discussions about innovation in light detection and energy conversion systems.