Understanding Heat Transfer: An In-Depth Exploration
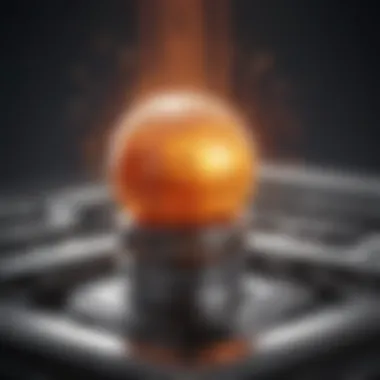
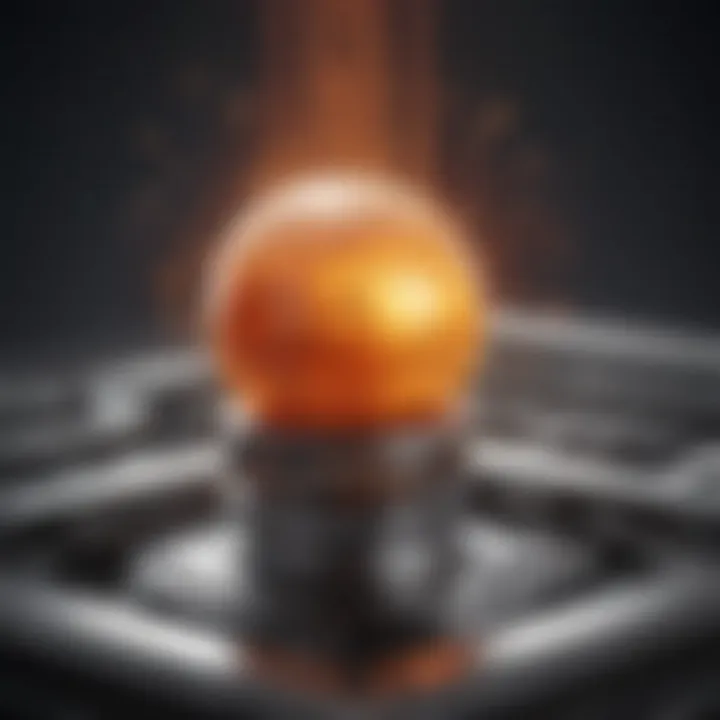
Intro
Heat transfer is a essential phenomena that shapes our understanding of a variety of systems, both natural and engineered. It plays a vital role in fields such as engineering, environmental science, and everyday technology. By grasping the fundamentals of heat transfer, professionals and students alike can apply principles that enhance innovation and efficiency in diverse applications.
This article embarks on a thorough examination of heat transfer, as it encompasses three primary mechanisms: conduction, convection, and radiation. Each mechanism operates under distinct principles and is relevant to specific contexts. Moreover, comprehending thermodynamics is crucial in these scenarios, as it provides a foundational understanding of energy exchanges and transformations. Through detailed examples from various industries and practical cases, we will unveil the complexities of heat transfer processes.
In a world where energy conservation and efficiency are paramount, the importance of understanding heat transfer cannot be overstated. Whether designing more effective cooling systems in electronics or improving insulation in buildings, the knowledge gained here is applicable across many disciplines.
The content presented in this article will empower the reader to not only understand the mechanisms involved but also to appreciate the implications these processes have on real-world applications.
Preface to Heat Transfer
Understanding heat transfer delivers critical insights into a fundamental aspect of physics and engineering. This concept directly affects various fields, including engineering, environmental science, and energy management. Grasping the essentials of heat transfer helps professionals and students tackle complex problems ranging from building design to thermal management in electronic devices. Given that heat transfer is central to thermodynamics, its implications extend to the efficiency and functionality of countless technical systems.
Definition of Heat Transfer
Heat transfer refers to the movement of thermal energy from one object or system to another. This movement occurs due to the temperature difference between two areas. In essence, heat transfers spontaneously from a warmer object to a cooler one. The methods of heat transfer can be categorized primarily into three types: conduction, convection, and radiation. Each method describes how thermal energy travels, either through direct contact, the motion of fluids, or electromagnetic waves, respectively. Understanding these methods forms the basis for analyzing heat-related phenomena in various applications.
Importance in Science and Engineering
The significance of heat transfer in science and engineering cannot be overstated. Without a firm understanding of heat transfer principles, it would be impossible to design effective thermal systems. Considerations like energy efficiency, safety standards, and performance outcomes depend on heat management strategies. In engineering disciplines, such as mechanical and civil engineering, knowledge of heat transfer aids in creating more robust structures and systems.
Furthermore, scientists utilize heat transfer principles to develop new technologies and optimize existing ones. For example, in the context of climate change, heat transfer analysis can assist in creating models that predict temperature changes in various environments. Without proper heat management, energy transformation processes may result in wasted resources or catastrophic failures.
"The study of heat transfer acts as a bridge between theory and practical application."
Through the lens of heat transfer, we emerge better equipped to handle challenges faced in research and real-world applications. This understanding serves as a foundation for further discussion in this article, elucidating the complexities and techniques necessary for tackling heat transfer in diverse contexts.
The Laws of Thermodynamics
The laws of thermodynamics form the foundation of heat transfer principles. Understanding these laws is essential for both theoretical and practical applications in physics and engineering. Thermodynamics explains how energy is transferred, converted, and conserved. Without this framework, many of the processes that govern heat transfer would remain obscure.
These laws not only outline energy conservation but also define limits on energy transformation efficiency, giving insight into how systems behave under various conditions.
First Law of Thermodynamics
The first law of thermodynamics, often referred to as the law of energy conservation, states that energy cannot be created or destroyed in an isolated system. It can only change from one form to another. For example, when heat is supplied to a system, some of it may be converted into work or stored as internal energy. The formulation is generally expressed as:
[ \Delta U = Q - W ]
where ( \Delta U ) is the change in internal energy, ( Q ) is the heat added to the system, and ( W ) is the work done by the system. This principle is crucial for various engineering applications. Understanding how energy transfers and transforms enables engineers to design better thermal systems and equipment.
Second Law of Thermodynamics
The second law of thermodynamics addresses the direction of energy transfers and the concept of entropy. This law asserts that energy transformations are not 100% efficient and that some energy will always disperse as waste heat. This implies that natural processes tend to move toward a state of greater disorder or randomness, increasing the universe's overall entropy.
A common expression of this law is that heat cannot spontaneously flow from a colder body to a hotter body. It underlines the limitations in converting heat energy into work. For example, in an engine, a portion of the generated heat escapes into the environment instead of being entirely converted into mechanical work. This principle has profound implications for the efficiency of thermal systems.
Applications of the Laws
Thermodynamics plays a critical role in multiple fields. Here are several areas where these laws are particularly influential:
- Engineering: The first and second laws guide the design of engines, refrigerators, and heat exchangers, ensuring they operate within thermodynamic limits while maximizing efficiency.
- Climate science: Understanding energy transfers helps explain phenomena like the greenhouse effect and climate systems.
- Material science: The laws inform how materials react under heat stress, guiding the development of new materials that can withstand extreme temperatures.
The laws of thermodynamics not only provide theoretical insights but also have extensive practical applications, fundamentally shaping engineering and environmental science.
Mechanisms of Heat Transfer
Mechanisms of heat transfer are fundamental concepts that underpin many processes in engineering and natural sciences. These mechanisms dictate how heat energy moves between different systems and are crucial for designing efficient thermal systems. Understanding heat transfer mechanisms helps in optimizing energy use across various applications, from industrial manufacturing to environmental science. In this section, we will explore conduction, convection, and radiation in detail.
Conduction
Definition
Conduction refers to the transfer of heat through a material without the movement of the material itself. This process occurs at the microscopic level as fast-moving particles collide with slower-moving particles, transferring energy in the process. The key characteristic of conduction is its reliance on molecular interactions. It is often the most common mechanism of heat transfer in solids.
One beneficial aspect of conduction is its predictability in steady-state applications, where temperature gradients are consistent. However, it is less effective over large distances compared to other methods, making its efficiency somewhat limited in certain applications, such as thermal insulation.
Fourier's Law
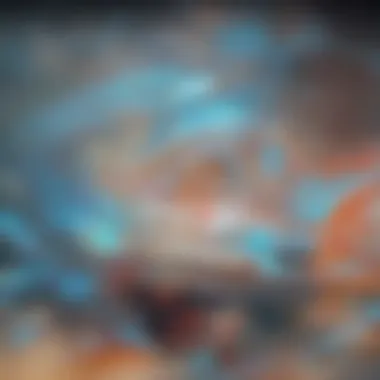
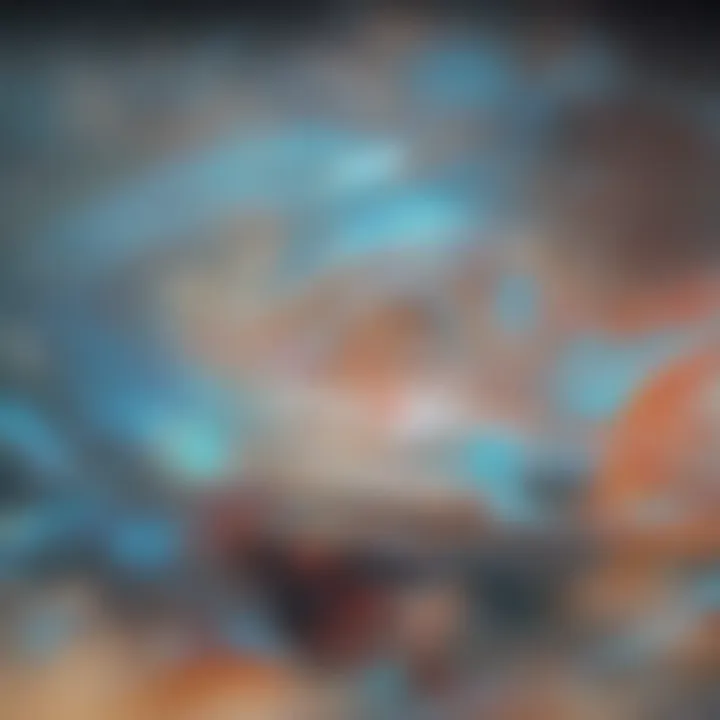
Fourier's Law describes the relationship between the heat transfer rate through a material and the temperature gradient across it. Mathematically, it can be expressed as:
[ q = -k \fracdTdx ]
where ( q ) is the heat transfer rate, ( k ) is the material's thermal conductivity, and ( \fracdTdx ) is the temperature gradient. A key characteristic of Fourier's Law is that it illustrates how heat flows from high to low temperature regions.
This law is a fundamental tool in thermal analysis, providing a framework for calculating heat conduction in various materials. However, it assumes a linear relationship that may not hold in all scenarios, particularly in non-homogeneous materials.
Materials and Thermal Conductivity
Thermal conductivity is a material property that quantifies how effectively heat can pass through a substance. Metals like copper and aluminum exhibit high thermal conductivity, while insulators like rubber and glass have low values. Understanding these properties is essential for selecting appropriate materials in heat transfer applications.
The key characteristic of materials concerning thermal conductivity is that it greatly influences the efficiency of heat transfer processes. High conductivity materials are favorable for heat sinks and exchangers, while low conductivity materials are crucial in insulation technologies. However, a disadvantage can arise from over-relied heat conductive materials in applications where heat retention is necessary.
Convection
Definition
Convection involves the transfer of heat by the physical movement of fluid, which includes both gases and liquids. As a fluid is heated, it becomes less dense and rises, allowing cooler fluid to take its place, this cycle continues as heat is distributed. The key characteristic of convection is its dependence on fluid motion, making it a dynamic form of heat transfer.
One advantage of convection is that it can transfer heat more efficiently than conduction, especially over larger distances. However, it requires some form of fluid motion, which might not be present in certain applications, rendering it less effective.
Natural vs. Forced Convection
Natural convection occurs due to buoyancy-driven flow, such as warm air rising in a room heated by a radiator. Forced convection, on the other hand, is induced by external forces, often fans or pumps, meaning more control over the heat transfer rate. A key characteristic of forced convection is its ability to enhance heat transfer rates, making it popular in many engineering applications.
Advantages of forced convection include consistency and efficiency in heating or cooling, making it suitable for modern HVAC systems. Yet, it often requires more energy input compared to natural convection, raising operational costs.
Heat Transfer Coefficients
Heat transfer coefficients are parameters that describe the efficiency of heat transfer through convection. It is a measure of the convective heat transfer between a surface and a fluid. The higher the coefficient, the more effective the heat transfer. This aspect is crucial when calculating heat exchanges in various systems.
The significant characteristic of heat transfer coefficients is their variability based on factors such as fluid velocity and surface geometry. While high coefficients are desirable for rapid heat transfer, they can imply a complex system which may require careful maintenance and design considerations to function efficiently.
Radiation
Definition
Radiation is the transfer of heat through electromagnetic waves and does not require a medium for transfer. All objects emit radiation based on their temperature, with hotter objects emitting more energy. The key characteristic of radiation is that it travels in straight lines and can occur even in a vacuum.
An advantage of radiation is its ability to transfer heat over distances without a medium, which can be essential in applications like solar energy harvesting. However, it often requires a direct line of sight between objects to be effective, meaning that obstacles can greatly impede heat transfer.
Stefan-Boltzmann Law
The Stefan-Boltzmann Law describes the power radiated from a black body in terms of its temperature, defined by the formula:
[ P = \sigma A T^4 ]
where ( P ) is the power, ( \sigma ) is the Stefan-Boltzmann constant, ( A ) is the area, and ( T ) is the absolute temperature. A notable characteristic of this law is that it shows radiation increases rapidly with temperature.
This law is quite beneficial for understanding energy exchanges in thermal processes, particularly in solar panel design. However, it can become complex when not dealing with ideal black bodies, requiring adjustments for real-world applications.
Real-World Examples
Real-world applications of radiation are ubiquitous, including solar energy utilization, thermal imaging, and radiative cooling of buildings. A key advantage of recognizing these applications lies in developing more effective systems for energy conservation.
However, it’s essential to note that radiation is less effective when involved in high humid environments where moist conditions can absorb significant radiant heat, impacting system efficiencies.
Heat Transfer Equations
Heat transfer equations serve as the foundation for understanding how heat moves through different materials and substances. They are critical for professionals in various fields including engineering, environmental science, and energy management. Understanding these equations aids in the design of more efficient thermal systems, informs research on climate change, and enhances everyday applications, such as in heating and cooling technologies. The analysis of these equations helps decipher the complex interactions between heat transfer mechanisms and their practical implications.
Heat Conduction Equation
The heat conduction equation, often referred to as Fourier's law of heat conduction, describes how heat flows through materials due to a temperature gradient. It can be expressed mathematically as:
[ q = -k \fracdTdx ]\
Where:
- ( q ) = Heat transfer rate (W)
- ( k ) = Thermal conductivity of the material (W/m·K)
- ( \fracdTdx ) = Temperature gradient (K/m)
This equation is essential for predicting temperature distributions within solid objects. It also serves as the basis for many engineering calculations regarding heat sinks, insulation materials, and building designs. Accurate modeling of heat conduction enables engineers to optimize materials for specific applications, ensuring both safety and efficiency in thermal management systems.
Convection Heat Transfer Equation
The convection heat transfer equation quantifies the heat transferred between a surface and a fluid in motion. It incorporates factors such as the type of fluid flow, the surface area, and the temperature difference between the surface and the fluid. The equation can be represented as:
[ q = hA(T_s - T_f) ]\
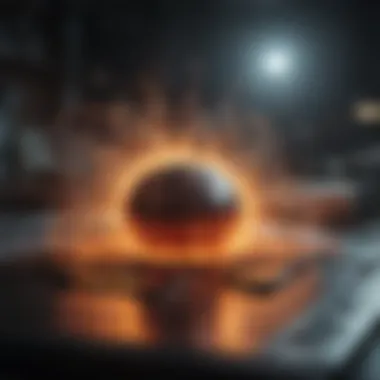
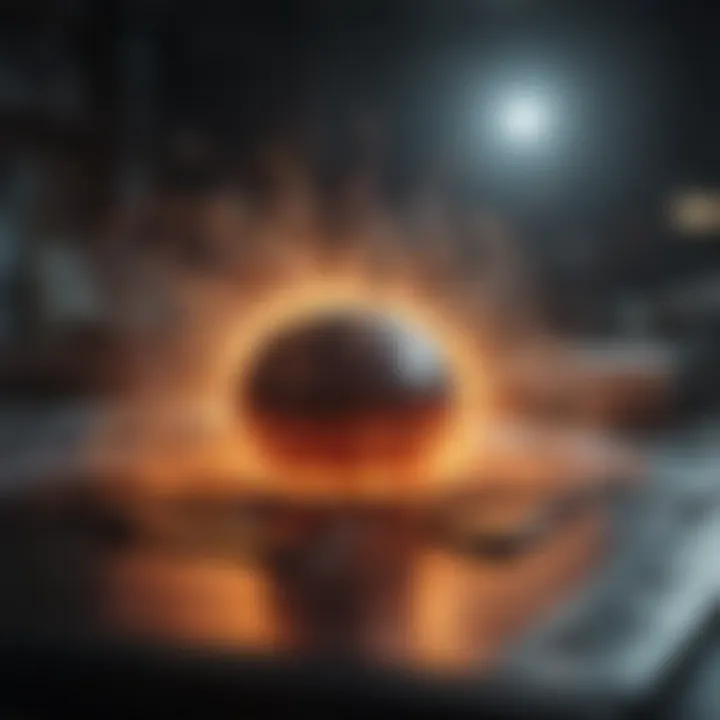
Where:
- ( q ) = Heat transfer rate (W)
- ( h ) = Convective heat transfer coefficient (W/m²·K)
- ( A ) = Surface area (m²)
- ( T_s ) = Surface temperature (K)
- ( T_f ) = Fluid temperature (K)
In this context, the convective heat transfer coefficient, ( h ), can vary significantly based on whether the fluid flow is natural or forced, along with the surface characteristics and temperature differences. This understanding is crucial in fields such as HVAC (heating, ventilation, and air conditioning), where optimizing heat transfer is vital for energy efficiency.
Radiation Heat Transfer Equation
The radiation heat transfer equation accounts for heat transfer through electromagnetic waves. It is characterized by the Stefan-Boltzmann law, which states:
[ q = \varepsilon \sigma A (T^4 - T_sur^4) ]\
Where:
- ( q ) = Heat transfer rate (W)
- ( \varepsilon ) = Emissivity of the surface (dimensionless)
- ( \sigma ) = Stefan-Boltzmann constant (5.67 x 10^(-8) W/m²·K⁴)
- ( A ) = Surface area (m²)
- ( T ) = Absolute temperature of the emitting surface (K)
- ( T_sur ) = Absolute temperature of the surrounding surface (K)
Radiation heat transfer plays a pivotal role in scenarios such as thermal insulation, space heating, and solar energy applications. By understanding how to manipulate these factors, engineers can better design systems that either maximize or minimize heat exchange, based on specific requirements.
"A solid grasp of heat transfer equations is necessary for making informed decisions in energy systems and ensuring effective thermal management in various applications."
In summary, heat transfer equations illustrate the fundamental principles governing heat movement across different mediums. These equations are indispensable tools for professionals and researchers, making them pivotal to advancements in thermal management technologies.
Applications of Heat Transfer
The applications of heat transfer are critical across numerous fields. Understanding how heat moves and behaves helps in improving energy efficiency, enhancing product performance, and developing technology. Whether in engineering, environmental science, or daily tasks, the principles of heat transfer are integral to achieving desired outcomes and optimizing processes.
In engineering, the practical application of heat transfer is evident in many systems. The design of thermal systems, heat exchangers, and refrigeration systems demonstrates how these principles operate in real-world scenarios. Effective heat management contributes to better performance and operational stability.
Environmental science also relyies heavily on heat transfer concepts. This is seen in climate systems, heat islands, and natural ecosystems, all of which showcase the influence of thermal dynamics on our environment. Understanding these can help in addressing climate change and improving urban planning.
In everyday life, heat transfer is present in cooking, building climate control, and electronics. Recognizing these applications can lead to innovations and practical solutions that improve our quality of life.
In Engineering
Thermal Systems
Thermal systems are crucial in the transfer and management of heat. They encompass everything from heating and cooling devices to power generation systems. A key characteristic of thermal systems is their ability to efficiently manage energy flow, which is vital for optimal performance. They are beneficial for improving energy conversion and usage.
The unique feature of thermal systems is their integration of various components like boilers and heat pumps, allowing for enhanced energy efficiency. However, their complexity can lead to challenges in maintenance and design, necessitating skilled professionals for effective operation.
Heat Exchangers
Heat exchangers play a pivotal role in facilitating heat transfer between two or more fluids. They are essential in industries like power generation and chemical processing. One of their key characteristics is the ability to transfer heat without mixing the fluids, which ensures energy efficiency.
Due to this capability, heat exchangers are a popular choice in applications requiring temperature regulation. The unique feature of these devices is their design flexibility, accommodating diverse operational needs. A disadvantage is that, if not properly maintained, they can lead to efficiency drops and operational issues.
Refrigeration Systems
Refrigeration systems are integral to food preservation and industrial processes. Their core characteristic is the removal of heat from one area to maintain lower temperatures in another. This application helps in increasing safety and extending the shelf life of perishable goods.
Refrigeration systems are popular due to their proven effectiveness in various applications. The unique feature is their ability to operate efficiently with proper design and maintenance. However, they can have high energy consumption, which leads to increased operational costs unless efficient technologies are used.
In Environmental Science
Climate Systems
Climate systems demonstrate the complex interplay of heat transfer mechanisms. They influence weather patterns and seasonal changes. A key characteristic of climate systems is their dynamic nature, responding to both natural factors and human activities.
Studying these systems is important for understanding climate change and its impacts. The unique feature is the large-scale interactions between the atmosphere, oceans, and land that govern global temperatures. The challenge is that their behavior can be unpredictable, complicating climate models.
Heat Islands
Heat islands arise when urban areas become significantly warmer than their rural counterparts. This phenomenon is mainly due to human activities and infrastructure. A key characteristic is the concentration of heat-absorbing materials, which can increase local temperatures.
Heat islands are important to consider in urban planning and environmental health. Their unique feature is the ability to exacerbate air conditioning needs, leading to higher energy consumption. Addressing these can mitigate negative effects, but efforts require comprehensive urban design strategies.
Ecosystems
Ecosystems rely on heat transfer for energy flow. The balance of energy within these systems is essential for sustaining life. A key characteristic is the diverse interactions between species, which can alter heat dynamics.
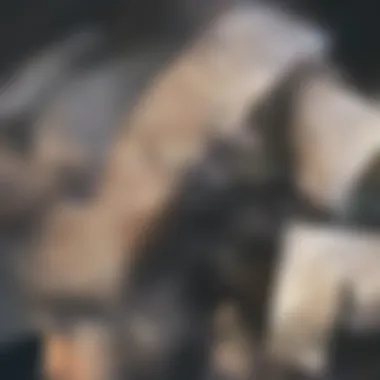
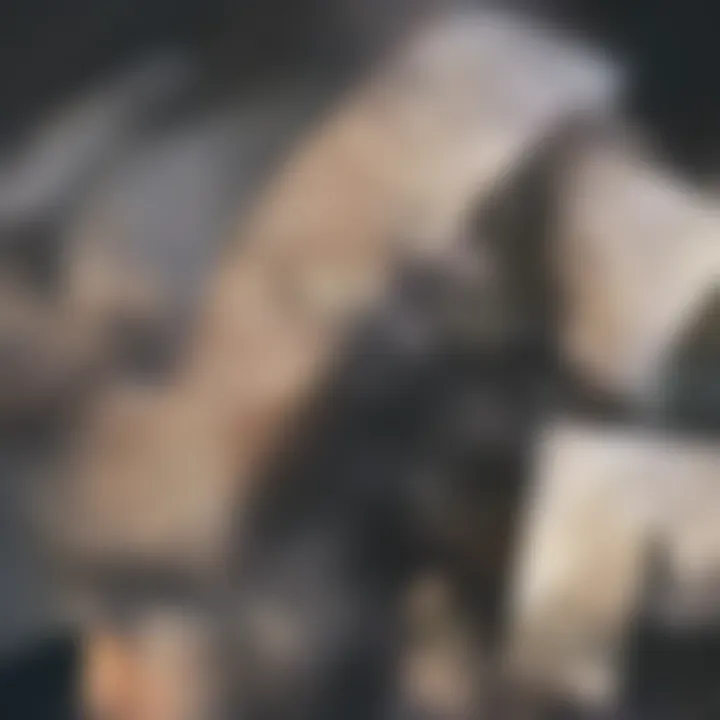
Understanding ecosystems is valuable for conservation efforts and promoting biodiversity. The unique feature is the sensitivity of ecosystems to changes in temperature and climate. Disruptions can lead to loss of species and habitat, emphasizing the need for sustainable practices.
In Everyday Life
Cooking
Cooking is a daily application of heat transfer. It involves using various methods such as boiling, frying, or baking, where temperature control is fundamental. A key characteristic is the transformation of ingredients through heat application, enhancing flavors and textures.
Cooking methods are popular due to their impact on food safety and palatability. A unique feature is the ability to modify heat intensity and duration, allowing for a range of cooking styles. However, improper techniques can lead to uneven cooking and waste.
Heating and Cooling of Buildings
Heating and cooling systems are vital in maintaining comfort levels in homes and offices. A key characteristic is their reliance on heat transfer principles to regulate indoor climates.
These systems are beneficial for energy efficiency when designed correctly. The unique feature is their adaptability to different building types and climate conditions. However, high installation and maintenance costs can be barriers for some homeowners.
Heat Sinks in Electronics
Heat sinks are essential components in electronic devices. They help manage heat generated by components like processors and prevent thermal overload. A key characteristic of heat sinks is their design, maximizing surface area for heat dissipation.
Heat sinks are popular because they ensure operational stability and prolong device lifespan. Their unique feature is the diversity of designs crafted for different applications. However, they can add bulk or weight to devices, requiring careful consideration in product design.
Advanced Topics in Heat Transfer
The exploration of Advanced Topics in Heat Transfer holds considerable significance in understanding modern applications and innovations in the field. As technology progresses, the intricacies involved in heat transfer become increasingly vital in various domains such as engineering, electronics, and environmental science. These advanced topics enhance our comprehension of heat transfer mechanisms and present new possibilities to optimize thermal management. This section delves into three prominent areas: nanofluids, phase change heat transfer, and heat transfer in microdevices, highlighting their relevance and potential impact on future technologies and research.
Nanofluids
Nanofluids represent a significant advancement in thermal fluids technology. These are colloidal dispersions of nanoparticles in a base fluid, such as water or oil. The inclusion of nanoparticles, typically made of metals or metal oxides, enhances the thermal conductivity of the base fluid. This results in improved heat transfer performance, essential in applications ranging from cooling systems to heat exchangers.
Some benefits of using nanofluids include:
- Increased Thermal Conductivity: Nanofluids can achieve thermal conductivities much higher than conventional fluids.
- Enhanced Heat Transfer Rate: The improved thermal properties often lead to a reduced size of heat exchangers or cooling systems.
- Potential for Energy Savings: Improved efficiency allows for less energy consumption in thermal management systems.
However, there are considerations. The stability of nanofluids can be challenging. Preventing particle agglomeration is crucial for maintaining their effectiveness. Research continues to explore various nanoparticles and base fluids to optimize these fluids for practical applications.
Phase Change Heat Transfer
Phase Change Heat Transfer involves the transfer of heat during the transition between solid, liquid, and gas states. This process is crucial in power generation, refrigeration, and even climate control systems. Common instances include boiling and condensation, both of which demonstrate significant heat transfer rates.
Key aspects of phase change heat transfer include:
- Latent Heat: This is the energy absorbed or released during a phase transition, which does not change the temperature.
- Heat Exchangers: These devices often utilize phase change processes to improve efficiency in energy systems.
- Thermal Management: Understanding these principles is essential for developing efficient HVAC systems.
The applications remain expansive. For example, in refrigeration, the vapor-compression cycle relies heavily on phase change heat transfer. By enhancing the understanding of this topic, researchers can develop better materials and systems that manage heat transfer more effectively.
Heat Transfer in Microdevices
Heat Transfer in Microdevices is an emerging field addressing the cooling needs of small-scale electronic components. As devices become smaller and more powerful, efficient thermal management is a key challenge. Microdevices often experience high heat fluxes, which require advanced methods of heat transfer to ensure functionality and longevity.
Considerations in this area include:
- Microchannel Cooling: Microchannel heat sinks offer an effective solution for managing heat in compact devices.
- Heat Transfer Coefficients: Understanding the coefficients that govern heat transfer in these small dimensions is critical.
- Innovative Materials: The study of new materials and designs can lead to better thermal performance.
The continuous development in heat transfer for microdevices is vital in sectors like consumer electronics, aerospace, and medical devices. Scientists and engineers are pushing boundaries to create more efficient and reliable thermal management solutions, significantly impacting technology development.
In summary, Advanced Topics in Heat Transfer encapsulate dynamic research and developments that influence modern applications. Emphasizing areas like nanofluids, phase change heat transfer, and heat transfer in microdevices provides a holistic view of the future trajectory of thermal management and efficiency.
Culmination
In this article, the exploration of heat transfer serves as a fundamental pillar for both scientific understanding and practical applications. The thorough examination of conduction, convection, and radiation not only highlights how energy moves within various systems but also demonstrates its essential role in real-world scenarios.
Summary of Key Points
Through our discussion, several key points have emerged:
- Mechanisms of Heat Transfer: Understanding the three primary modes—conduction, convection, and radiation—enables one to analyze thermal behavior in diverse contexts.
- Laws of Thermodynamics: The integration of these laws is crucial as they govern how energy is conserved and transformed.
- Real-World Applications: From engineering designs to environmental considerations and daily life, heat transfer plays a significant role in optimizing performance and efficiency.
Future Directions in Heat Transfer Research
Looking ahead, the field of heat transfer continues to evolve, with emerging trends pointing toward several avenues for future exploration:
- Nanotechnology: Investigating the properties of nanofluids can lead to breakthroughs in thermal management in various industries, including electronics and energy production.
- Phase Change Materials: Research into these materials may enhance thermal storage solutions, addressing challenges in energy efficiency.
- Sustainable Practices: As global focus shifts toward renewable energy, the application of heat transfer principles in solar energy systems and building design remains a significant area of study.
"The future of heat transfer research is vital for sustainable innovation and solving modern energy challenges."
Overall, the advancement of heat transfer knowledge will significantly impact technology, environmental science, and energy efficiency in various fields.