Sustainability Trends in Mechanical Engineering

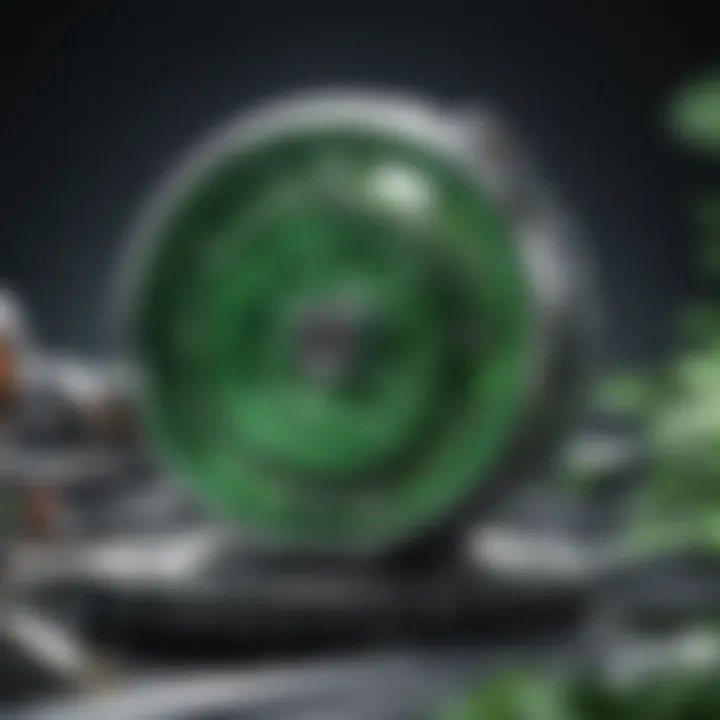
Intro
Sustainability is becoming a central theme in many fields today, especially in mechanical engineering. The implications of environmental impact are pushing professionals to rethink traditional methods. Mechanical engineering, as a discipline, encompasses a vast array of applications—from machine design to manufacturing processes. Recognizing the urgency of ecological preservation, integration of sustainability principles into mechanical engineering is no longer optional but rather essential.
This article aims to elucidate how sustainability permeates mechanical engineering practices. It focuses on current trends and innovations that encourage reduced waste and efficient resource use. In addition, it will explore how renewable energy sources are reshaping the design of mechanical systems. Accompanying this discussion are insights into regulatory frameworks that guide sustainable practices, and how educational efforts can ensure future engineers are equipped to face these challenges.
By delving into these areas, we seek to outline a path for mechanical engineers committed to fostering a more sustainable future. This exploration will greatly benefit students, researchers, educators, and professionals who are keen to enhance their understanding of sustainability's role in their field.
Preamble to Sustainability in Engineering
Sustainability in engineering has emerged as a critical framework guiding the practices of various engineering disciplines, notably mechanical engineering. This shift signifies a growing awareness among professionals about the environmental impact of engineering solutions. The importance of sustainability cannot be overstated, as it fundamentally influences the way engineers approach design, materials selection, and manufacturing processes.
Focusing on sustainability helps to address pressing global challenges, such as climate change and resource depletion. By prioritizing sustainable methods, engineers can minimize the negative environmental consequences of their work. For mechanical engineers, this means designing systems that use energy more efficiently, selecting materials with a lower carbon footprint, and implementing practices that conserve resources.
Several key elements exemplify the importance of sustainability in engineering:
- Resource Efficiency: Sustainable practices promote the efficient use of natural resources, ensuring that materials are used judiciously and recycled or reused wherever possible.
- Economic Viability: Sustainability is not just beneficial for the environment; it can also lead to cost savings. By investing in energy-efficient technologies and sustainable materials, companies can reduce operational costs over time.
- Social Responsibility: Engineers have a responsibility to society. By integrating sustainable practices into their work, they contribute positively to community well-being and help to create a resilient economy.
- Regulatory Compliance: With increasing governmental regulations addressing environmental issues, engineers must align their projects with these frameworks to avoid legal and financial penalties.
The benefits of adopting sustainability in engineering practices are manifold. It encourages innovation by pushing engineers to develop new technologies and approaches. Moreover, it fosters a culture of accountability and integrity within organizations, as stakeholders increasingly demand transparency regarding environmental impacts.
When considering sustainable engineering, it is also essential to evaluate the life cycle of products. This encompasses everything from raw material extraction to manufacturing, use, and eventual disposal. By understanding and improving these phases, engineers can significantly mitigate adverse effects on the environment.
"The transition to sustainable engineering requires not just innovative technologies, but also a fundamental change in how we think about our work and its impact on future generations."
Thus, the introduction of sustainability into engineering practices marks a paradigm shift towards a more ethical and responsible approach. This foundation sets the stage for discussions on various aspects specific to mechanical engineering, leading into more detailed examinations of energy efficiency, materials, manufacturing practices, and more. Understanding these principles forms the backbone of sustainable mechanical engineering.
Defining Sustainability
Understanding sustainability is crucial to integrating it into mechanical engineering. Sustainability is the ability to meet present needs without compromising the ability of future generations to meet their own needs. This concept encompasses environmental, economic, and social dimensions. In mechanical engineering, it calls for practices that minimize negative impacts on the planet while promoting innovation and design that respect resources.
Historical Context
The idea of sustainability has evolved over time. Originally, environmental concerns began gaining traction in the 1960s and 1970s. Books like Silent Spring by Rachel Carson raised awareness about the effects of pesticides on nature. By the late 1980s, the Brundtland Commission popularized the term “sustainable development” in its report, which emphasized looking at how economic growth and environmental preservation can coexist. This history reflects an increasing recognition of our changing relationship with the environment and the need for mindful engineering approaches.
As mechanical engineering has advanced, so too has the understanding of sustainability. For instance, earlier engineering practices often prioritized efficiency in productivity without considering environmental consequences. Today, the focus shifts toward holistic methodologies that include life cycle assessments and resource management strategies. Companies now recognize that sustainable practices not only benefit the environment but can also reduce costs and improve public perception.
Key Principles of Sustainability
- Resource Efficiency: Optimizing resource use helps reduce waste and pollution. This can include energy-efficient designs and manufacturing processes.
- Eco-Design: Products should be designed with their entire life cycle in mind, from material extraction to disposal. This approach considers the environmental impact and the sustainability of materials.
- Social Responsibility: Engineering practices should consider societal implications. This involves engaging with communities, respecting labor rights, and enhancing quality of life.
- Economic Viability: Sustainable solutions need to be economically attractive. If they are not cost-effective, they will not be adopted widely.
By following these principles, mechanical engineers contribute to sustainable solutions that are socially equitable and environmentally responsible while ensuring economic viability. In this way, sustainability becomes a pathway to innovation rather than an obstacle.
The Role of Mechanical Engineering in Sustainable Development
Mechanical engineering is pivotal in shaping sustainable development across various industries. This discipline integrates principles of design, analysis, and manufacturing with a focus on minimizing environmental impacts and enhancing operational efficiency. By leveraging advancements in technology, mechanical engineers can create systems that not only meet current demands but also anticipate future challenges. This foresight is crucial as society increasingly prioritizes sustainability in its development agenda.
Energy Efficiency in Mechanical Systems
Energy efficiency is a core aspect of mechanical systems. Mechanical engineers are tasked with designing systems that optimize energy use in processes ranging from manufacturing to transportation. Improved energy efficiency leads to reduced operational costs and decreases in greenhouse gas emissions. The implementation of energy-efficient technologies can significantly lower a facility's energy consumption.
One approach to enhance efficiency is through the use of advanced simulation tools. These tools help in analyzing thermal and fluid dynamics, enabling engineers to refine designs for machines and systems. For instance, HVAC (heating, ventilation, and air conditioning) systems, when optimized, can significantly reduce energy wastage.
Some effective strategies include:
- Employing high-efficiency motors and drives
- Implementing variable frequency drives (VFDs) for motors
- Utilizing energy recovery systems in manufacturing
According to recent studies, the adoption of highly efficient systems can lead to energy savings of up to 30% in certain applications. This not only conserves resources but also aligns with global initiatives aimed at reducing carbon emissions.
Reduction of Carbon Footprint
The mechanical engineering sector plays a critical role in reducing the carbon footprint of various industries. This is accomplished through the redesign of products and processes with sustainability in mind. Engineers are exploring lightweight materials and more efficient production methods to lower emissions.
The shift towards renewable energy sources is another significant factor in this reduction. Mechanical engineers are increasingly involved in designing equipment and systems that harness energy from wind, solar, and hydro sources. By doing so, they contribute directly to a decrease in reliance on fossil fuels, a major contributor to carbon emissions.
Key actions include:
- Integrating renewable energy sources into mechanical designs
- Innovating waste heat recovery systems
- Developing efficient combustion systems for engines
"The transition to a low-carbon economy is not just a challenge, but an opportunity for innovation in mechanical engineering."
Innovative Materials for Sustainable Engineering
Innovative materials play a crucial role in advancing sustainability within mechanical engineering. As the global focus shifts towards reducing environmental impacts, the demand for effective and sustainable materials continues to grow. These materials not only contribute to resource conservation but also enhance the overall efficiency of engineering applications.
Employing innovative materials like biodegradable and recycled materials can effectively minimize waste during production and end-of-life stages. Their integration into engineering processes showcases a clear commitment to sustainability. Additionally, utilizing these materials often leads to lighter, stronger products and systems, which can reduce energy consumption in various fields.
Focusing on innovative materials also aligns with market trends. As consumers become increasingly aware of environmental issues, products made from sustainable materials attract more attention. Therefore, manufacturing industries that prioritize these materials position themselves favorably in the competitive landscape.
Biodegradable Materials
Biodegradable materials have gained prominence in sustainable engineering due to their capacity to decompose naturally. This characteristic significantly reduces waste accumulation in landfills and other environments. Examples of biodegradable materials include polylactic acid (PLA) and polyhydroxyalkanoates (PHA). These are derived from renewable resources, making them preferable to conventional plastics.
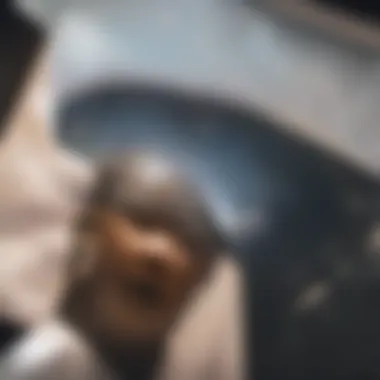
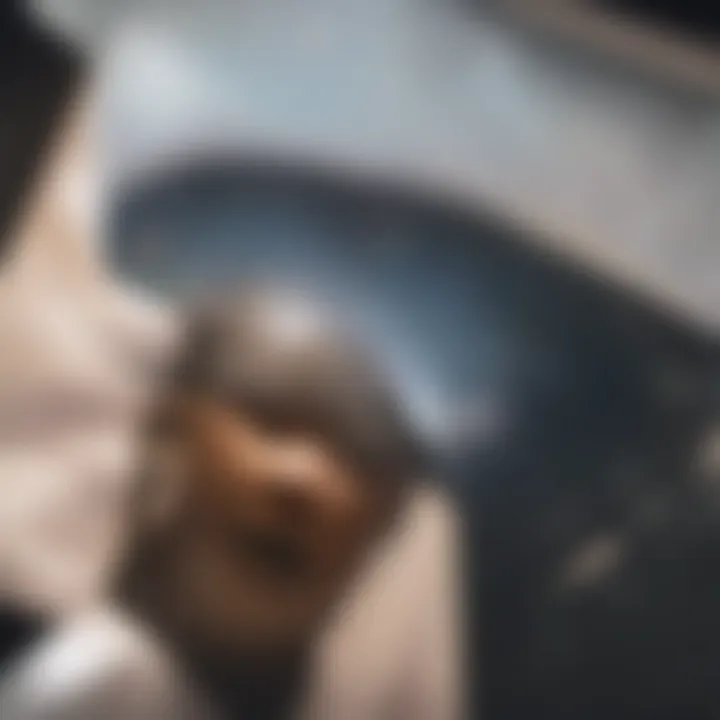
Benefits of incorporating biodegradable materials in mechanical engineering include:
- Reduced environmental footprint.
- Compliance with stringent regulations concerning environmental standards.
- Enhanced company reputation among eco-conscious consumers.
However, it is essential to consider aspects such as performance, cost, and processing methods when selecting biodegradable materials for specific applications. In some cases, performance may not match traditional materials, leading to restrictions on their use in critical components.
Recycled and Recyclable Materials
Recycled and recyclable materials represent another important area in sustainable mechanical engineering. By using materials that have been previously utilized and processed, industries can significantly decrease the extraction of virgin resources. Commonly recycled materials include metals, glass, and certain plastics. On the other hand, recyclable materials remain valuable after their initial use, allowing them to be processed and repurposed into new products.
The advantages of employing recycled and recyclable materials are:
- Lower production costs through reduced material acquisition expenses.
- Decreased environmental impact, as less waste is generated from manufacturing processes.
- Preservation of natural resources for future generations.
It is vital to ensure that the recycling processes these materials undergo do not compromise their strength and usability in engineering applications. Striking a balance between sustainability and functionality is a key undertaking in this domain.
"The choice of materials in engineering significantly impacts sustainability outcomes."
Green Manufacturing Practices
Green manufacturing practices represent a crucial approach within mechanical engineering, focusing on reducing environmental impacts while maintaining operational efficiency. This practice prioritizes sustainable methods throughout the manufacturing process. It encompasses a variety of techniques and innovations aimed at minimizing waste, conserving energy, and utilizing sustainable materials. The significance in this article is evident as it highlights a holistic view of how mechanical engineering can adapt to meet the demands of sustainability.
Adopting green manufacturing practices not only enhances the environmental performance of manufacturing facilities but also leads to economic benefits. Companies that implement these practices often experience reductions in operational costs due to lower waste disposal fees, reduced energy consumption, and the efficient use of materials. Moreover, there is increased consumer demand for products made with environmentally-friendly processes, making these practices attractive from a market perspective.
Lean Manufacturing
Lean manufacturing is a methodology that seeks to enhance productivity by minimizing waste without sacrificing quality. It aligns well with sustainability efforts, as both aim for efficient resource use. The principles of lean manufacturing, such as value stream mapping and continuous improvement, lead to a streamlined production process. For instance, by identifying and eliminating non-value-adding activities, manufacturers can reduce not just waste but also energy consumption.
Implementing lean principles can result in significant cost savings. For example, in a traditional manufacturing environment, the inefficiencies in operations might lead to excess material usage and energy waste. Yet, a lean approach can systematically reduce these factors. Additionally, lean manufacturing emphasizes quality at every stage, reducing defect rates and thus minimizing the need for rework, which can be resource-intensive.
Additive Manufacturing
Additive manufacturing, commonly known as 3D printing, is another significant innovation in the realm of green manufacturing practices. This technology builds objects layer by layer from digital models, which inherently reduces waste compared to subtractive manufacturing methods. Traditional manufacturing often involves removing material from a larger block, leading to a significant amount of scrap. In contrast, additive manufacturing only utilizes the material necessary to create the finished product.
Furthermore, 3D printing enables the use of sustainable and even biodegradable materials. This development aligns with the shift towards an environmentally responsible manufacturing ecosystem. Applications range from prototyping to large-scale production across various industries including automotive and aerospace. As further advancements occur, the potential for additive manufacturing to contribute to sustainable practices expands.
"The integration of lean and additive manufacturing can create powerful synergies, amplifying both efficiency and sustainability in production."
Renewable Energy Integration
The integration of renewable energy sources into mechanical engineering is crucial for advancing sustainable practices. This concept emphasizes the need for engineers to develop systems and products that utilize renewable resources, thereby minimizing reliance on fossil fuels. By embracing renewable energy integration, the field of mechanical engineering can contribute significantly to reducing greenhouse gas emissions and fostering a more sustainable future.
Benefits of Renewable Energy Integration:
- Environmental Impact: Utilizing renewable energy sources like wind and solar helps to significantly reduce pollution.
- Energy Security: By diversifying energy sources, nations can protect themselves from fluctuating fossil fuel prices.
- Economic Growth: Investment in renewable energy technologies can spur job creation and stimulate economic progress.
Incorporating renewable energy technologies into mechanical systems not only enhances energy efficiency but also reduces operating costs in the long run. As the demand for sustainable solutions continues to rise, the role of renewable energy in mechanical engineering cannot be overlooked.
Wind Energy Technologies
Wind energy technologies are among the most promising forms of renewable energy. They convert kinetic energy from wind into electricity using wind turbines. The efficiency of these turbines is continually improving through advancements in design and materials.
Factors to consider in wind energy include:
- Site Assessment: Locations with strong and consistent winds are essential for maximizing efficiency.
- Turbine Design: Modern turbines feature innovations like larger blades and improved aerodynamics, which increase energy capture.
- Grid Integration: Effective systems must be in place to integrate wind energy into existing electricity grids.
As wind energy becomes more viable economically, its implementation in mechanical engineering applications grows even more significant, leading to cleaner energy production.
Solar Energy Applications
Solar energy applications harness the power of the sun to generate electricity, either directly through photovoltaics or indirectly through solar thermal energy. This integration is increasingly relevant in mechanical engineering, where systems can be designed to utilize solar power effectively.
Considerations for solar energy applications include:
- Photovoltaic Systems: These systems convert sunlight directly into electricity and can be installed on various scales, from small residential systems to large solar farms.
- Solar Thermal Systems: These systems use sunlight to heat a fluid, which can then generate steam to drive turbines and produce electricity.
- Energy Storage: The reliance on energy storage solutions, such as batteries, is essential for managing periods when sunlight is not available.
Solar energy applications represent a large potential for innovation in mechanical engineering. By focusing on designs that optimize sunlight capture and energy efficiency, the field can significantly lower environmental impact while providing essential energy solutions.
Regulatory Frameworks and Policies
Global Environmental Policies
Global environmental policies provide a foundation for international cooperation in tackling sustainability issues. Agreements like the Paris Agreement set ambitious targets for reducing greenhouse gas emissions. Mechanical engineering plays a vital role in achieving these targets through improved energy efficiency and cleaner production techniques. Countries are encouraged to develop incentives and set emissions reductions plans. This affects mechanical engineering projects on various scales, driving the need for companies to embrace sustainable practices.
Furthermore, these policies promote research and development in sustainable technologies. Innovative approaches such as carbon capture and storage technology have emerged due to this global push. Such developments can significantly alter how mechanical systems operate, paving the way for more sustainable energy practices and reducing the overall carbon footprint on a global scale.
Local Initiatives and Standards
Local initiatives and standards represent the operational guidelines tailored to regional needs. They complement global policies by addressing specific challenges faced by local communities. For example, communities may implement regulations that require energy efficiency upgrades in older mechanical systems, ensuring ongoing compliance with sustainability goals. Local standards can also promote the utilization of renewable resources in mechanical engineering processes, enhancing energy independence and reducing reliance on fossil fuels.
Additionally, local initiatives often involve the collaboration of various stakeholders, including government bodies, industry representatives, and community organizations. This collaborative approach fosters a sense of ownership and accountability among local actors, encouraging them to innovate and strive for sustainability.
Regulatory frameworks guide the industry, ensuring that sustainability is not just an option but a necessity.
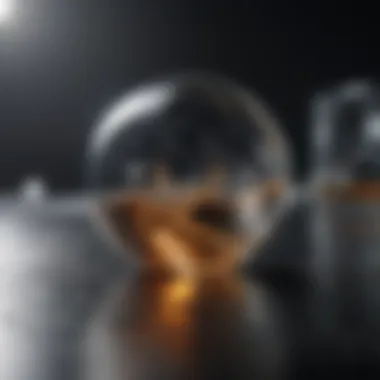
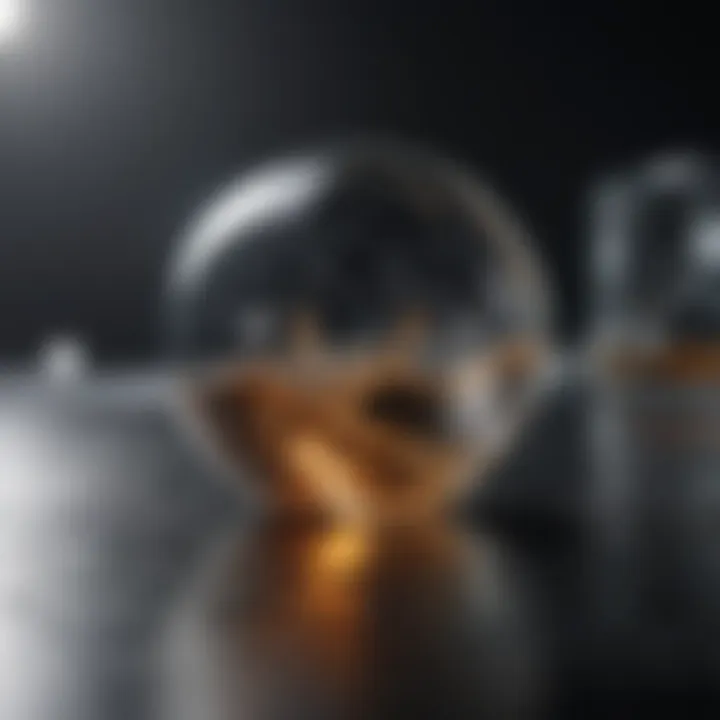
Aligning with these policies can lead to significant advancements in sustainable practices, highlighting the role of mechanical engineering in creating a greener future.
Challenges in Implementing Sustainable Practices
The journey toward integrating sustainability within mechanical engineering is often clouded by numerous challenges. These hurdles can slow progress and limit innovation.
Understanding these challenges is crucial. Addressing them leads to more effective strategies, enabling industries to overcome barriers. It is essential to focus on both economic and technological aspects. Doing so allows for comprehensive discussions. This examination highlights why addressing these challenges must be a priority.
Economic Barriers
Economic factors significantly affect the adoption of sustainable practices in mechanical engineering. The high initial costs associated with sustainable technologies often discourage companies from making investments. For instance, switching to advanced materials or energy-efficient systems can require substantial upfront capital. Many firms, particularly small and midsize enterprises, may struggle to justify these expenditures, especially when immediate returns are not visible.
Moreover, market competition often prioritizes cost reduction over sustainability. Companies may be reluctant to adopt costly measures if competitors offer cheaper alternatives. The result can be a race to the bottom, where concerns for the environment take a backseat.
Some key economic barriers include:
- Initial capital investment requirements
- Long payback periods for sustainable projects
- Existing infrastructure that is not sustainable
- Market pressures favoring short-term profits
To overcome these barriers, companies need support from governments and financial institutions. Subsidies or tax incentives can help lower the entry price. Collaboration with other companies to share costs and risks is also a viable strategy.
Technological Limitations
Some prevalent technological challenges include:
- Lack of mature technologies for scalability
- Insufficient training for engineers and workers
- Complexities in retrofitting old systems with new sustainable technologies
These limitations can inhibit the adoption rate of sustainable practices. Therefore, ongoing research and development are critical. Companies should invest in training programs to equip their workforce with the necessary skills.
Ultimately, tackling both economic and technological barriers is essential. This will pave the way for a future where sustainable practices are the norm, rather than the exception.
Case Studies: Success Stories in Sustainability
Industry-Leading Companies
Several companies have taken significant strides in integrating sustainability into their operations. For example, Siemens has established initiatives focused on energy efficiency and has committed to being carbon neutral by 2030. Their use of smart technology in manufacturing helps optimize energy consumption, thereby reducing the overall carbon footprint. Such a commitment allows Siemens to lead the way in green practices while maintaining competitive advantages in a rapidly evolving market.
Another noteworthy example is General Electric (GE). Their investment in renewable energy technologies, particularly in wind power, demonstrates the potential of large-scale sustainable solutions. GE's innovative wind turbines not only generate clean energy but also incorporate advanced materials and design techniques that enhance efficiency and durability.
These companies set benchmarks in sustainability that can inspire others within the engineering sector.
Innovative Projects and Research
Various research projects are pushing boundaries in sustainable mechanical engineering. One such initiative is the work done on 3D-printed structures using bio-based materials. Researchers have developed methods to create building materials that are not only strong but also environmentally friendly. This means less reliance on conventional materials, which often come with high carbon emissions during production.
Additionally, projects like the Solar Impulse Foundation showcase the integration of renewable energy in aviation. This aircraft, powered solely by solar energy, demonstrates the potential of sustainable engineering to revolutionize transportation.
These examples of innovative projects underline the importance of collaboration between academia and industry. They highlight how research not only contributes to our understanding of sustainable practices but also leads to practical applications that can be adopted on a larger scale.
The Future of Sustainable Mechanical Engineering
The future of sustainable mechanical engineering holds immense potential. It is not just about making designs more efficient; it's a thoughtful approach to harmonizing technology with environmental stewardship. As society shifts its focus to greener solutions, mechanical engineering professionals face the critical task of integrating sustainability into every project. Key factors like regulatory demands, consumer preferences, and innovations in technology all shape this future.
One of the main advantages of a sustainable future is the reduction of waste. By adopting principles of sustainable design, engineers can minimize the use of resources while maximizing utility. This can lead to cost savings, improved production timelines and a better public image for companies. Furthermore, in a world increasingly driven by climate change, adapting engineering practices to mitigate environmental impact becomes not just beneficial, but crucial.
"The importance of sustainability transcends mere compliance; it is about envisioning a world where engineering practices contribute positively to our planet."
To ensure a relevant and comprehensive approach, we must also consider education and workforce development as future cornerstones of sustainable mechanical engineering. Cross-disciplinary knowledge involving economics, environmental science, and engineering design will become essential.
Emerging Technologies
Emerging technologies are set to revolutionize mechanical engineering's sustainability practices. Innovations like artificial intelligence and machine learning allow for data-driven decision-making, resulting in more efficient processes and reduced waste. They enable predictive maintenance in machinery, thus extending equipment life and cutting down on replacements.
Additive manufacturing, also known as 3D printing, is changing the production landscape. This technology allows engineers to create complex shapes and structures with minimal waste. Furthermore, it supports the use of novel materials that may offer better performance and reduced environmental impact.
In addition, advancements in energy storage technologies significantly contribute to sustainability. Improved battery technologies such as lithium-sulfur or solid-state batteries may enhance the efficiency of renewable energy systems, supporting their widespread adoption across industries.
Potential Growth Areas
The potential for growth in sustainable mechanical engineering is broad and multifaceted. Significant areas include:
- Renewable Energy Systems: The push towards wind and solar power is creating demand for engineers who can design and optimize these systems. There's an ongoing need for innovation in energy capture and storage technologies.
- Sustainable Transportation: Electric and hybrid vehicles are gaining traction. Engineers in this sector will focus on lighter materials and more efficient powertrains to enhance performance and sustainability.
- Circular Economy Practices: Companies are increasingly striving to design products for longevity, reuse, and recyclability. This broadens the role of mechanical engineers in advocating for and implementing circular systems within industries.
- Smart Manufacturing: By combining Industry 4.0 with sustainability goals, manufacturers can optimize processes through real-time data analytics, ultimately reducing waste and energy consumption.
Integrating Sustainability into Engineering Education
The integration of sustainability into engineering education represents a fundamental shift in how future engineers are trained. As the field of mechanical engineering evolves, educators must adapt their curricula to include sustainable practices, ensuring that students not only understand engineering principles but also the environmental impact of their decisions. This transition is important for several reasons.
First, it prepares graduates to tackle complex global challenges, such as climate change and resource depletion. By embedding sustainability into the educational framework, students become equipped with the tools and knowledge to design systems and products that minimize negative environmental effects. Moreover, this approach aligns educational outcomes with the increasing demand for sustainable practices across industries, making graduates more employable and relevant in the modern workforce.
Second, engineering curricula that emphasize sustainability foster critical thinking and innovation. Students learn to question traditional methods of design and manufacturing. This mindset encourages them to seek alternative solutions that prioritize ecological balance. As they engage in projects that focus on sustainability, they start to develop a sense of responsibility toward the planet. This change in perspective is essential for future leaders in engineering.
Finally, integrating sustainability into engineering education fosters interdisciplinary collaboration. Mechanical engineers frequently work with professionals from other fields, such as environmental science and policy. By exposing students to concepts from various disciplines, educational programs create a more holistic understanding of sustainability challenges.
Curriculum Development
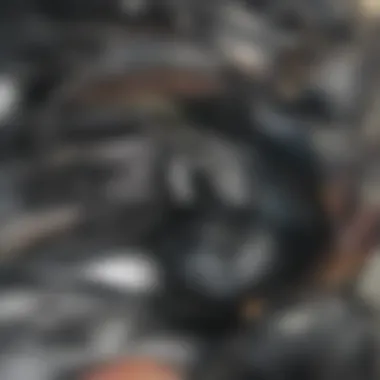
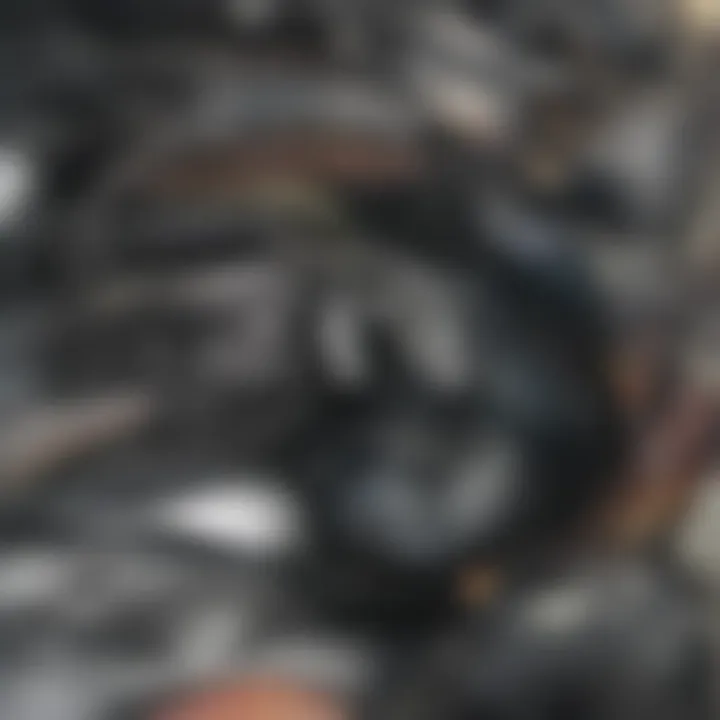
Curriculum development in the realm of sustainability must consider various aspects to become truly effective. It is not merely about adding a few courses to existing programs; it involves a comprehensive redesign that embeds sustainability throughout the entire educational experience.
Key elements of curriculum development include:
- Core Courses: Traditional subjects like thermodynamics and fluid mechanics should incorporate sustainability topics. For instance, discussions on energy efficiency in mechanical systems can be included.
- Project-Based Learning: Hands-on projects that address real-world sustainability challenges can stimulate students' critical thinking and problem-solving skills. Examples include designing a solar-powered device or developing strategies to reduce waste in manufacturing processes.
- Interdisciplinary Projects: Collaborating with other departments can create a more enriched learning experience. Engineering students could work with environmental scientists or social scientists to develop comprehensive solutions that consider multiple viewpoints.
Industry Partnerships
Partnerships with industry play a crucial role in enriching sustainability education. These collaborations provide students with real-world context and opportunities to apply their learning in practical settings. Industry partners can bring valuable insights into current practices and emerging trends.
Benefits of industry partnerships include:
- Internships and Co-op Programs: These experiential opportunities allow students to gain insights into sustainable practices already implemented in the industry. They can see firsthand how theoretical knowledge translates into practical application.
- Guest Lectures and Workshops: Professionals from the industry can share their experiences in sustainable engineering projects. Inviting experts can deepen students' understanding and provide networking opportunities.
- Joint Research Initiatives: Collaborative research projects between universities and companies can yield innovative solutions and place students at the forefront of sustainability advancements in mechanical engineering.
As educators, connecting students with industry helps create a bridge between academia and practice. This linkage not only enhances student learning but also fosters a workforce ready to innovate and lead in sustainable engineering.
Collaboration in Sustainability Initiatives
Collaboration in sustainability initiatives plays a vital role in the advancement of sustainable practices within mechanical engineering. By uniting various stakeholders, including private companies, governmental agencies, universities, and sustainability organizations, the field can leverage diverse expertise and resources. This cooperative approach enables the sharing of knowledge and innovation, driving better outcomes in sustainability.
One of the key benefits of collaboration is the pooling of resources. For example, companies may not have the full spectrum of tools or technologies necessary to implement green practices independently. Collaborative initiatives allow for resource-sharing, which might include technologies, financial investments, and manpower. This not only reduces costs but also enhances project efficiency.
Moreover, collaboration fosters a culture of innovation. When different entities come together, they bring unique perspectives, approaches, and problem-solving capabilities. This can lead to unexpected and innovative solutions that might not emerge in isolation. Networking through collaborative efforts can also accelerate the pace at which sustainable technologies are developed and adopted.
It is also important to note that collective efforts can amplify the impact of sustainability goals. For example, a private company might partner with a public institution to develop renewable energy systems that benefit both the environment and the local economy. This synergy creates a multiplier effect – the efforts of two entities working together can have a greater impact than acting alone.
Nonetheless, challenges do exist in fostering these collaborations. Communication and alignment of goals between different stakeholders can be complex. It is crucial to develop clear frameworks and mutual contracts that define responsibilities, expectations, and outcomes. Effective leadership is needed to navigate potential conflicts and ensure that all parties are committing to genuine and reciprocal partnership.
Collectively, these considerations demonstrate that collaboration in sustainability initiatives is not just beneficial; it is essential for driving meaningful change in mechanical engineering, paving the way for a more sustainable future.
Public-Private Partnerships
Public-private partnerships (PPPs) have emerged as key players in advancing sustainability. These collaborations harness the strengths of both sectors to drive projects that serve the public interest while providing private entities with opportunities for innovation and profit.
In the context of mechanical engineering, PPPs often target infrastructural projects focused on energy efficiency, waste reduction, and resource optimization. For example, a city might collaborate with a private engineering firm to retrofit existing public transport systems with energy-efficient technologies. This kind of project not only benefits the environment but can also reduce operational costs for the city in the long run.
The unique structure of PPPs allows for shared risk. By combining public funding with private sector efficiency, projects often achieve better financial health and resource management. Additionally, private firms may benefit from public sector credibility and access to lucrative contracts, creating a win-win scenario for both sides.
However, transparency remains critical in these partnerships. Ensuring that both public and private interests are accounted for can prevent potential pitfalls. Stakeholders should engage in ongoing dialogue to address any issues that arise and maintain public trust.
Academic Collaborations
These partnerships often involve joint research initiatives aimed at exploring new technologies, materials, and processes that support sustainable engineering. For instance, university-led research on advanced materials can be pivotal in developing lighter, stronger composites that reduce energy consumption during production and use.
Moreover, academic collaborations also play a significant role in shaping future engineers. By working with industry partners, students gain hands-on experience with real-world sustainability challenges. This not only enhances their educational journey but also prepares them to be better problem solvers in their careers.
Additionally, bridging the gap between academia and industry fosters continuous learning and adaptation within mechanical engineering as both sectors benefit from each other's insights and advancements. When academic institutions and industry players collaborate effectively, they create a robust framework for innovation that is aligned with sustainability goals.
Key Metrics for Assessing Sustainability
Key metrics for assessing sustainability are essential tools that provide frameworks for measuring and improving sustainability efforts within mechanical engineering. These metrics allow engineers, companies, and researchers to quantify their environmental impact and track progress over time. By defining clear, quantifiable indicators of sustainability, stakeholders can make informed decisions that lead to improved environmental and economic outcomes.
Life Cycle Assessment
Life Cycle Assessment (LCA) is a crucial method in evaluating the environmental impacts associated with all the stages of a product's life from raw material extraction through to disposal. This systematic approach helps in understanding the cumulative environmental effects, including resource consumption, energy use, and greenhouse gas emissions. The following points elucidate the importance of LCA:
- Holistic Perspective: LCA considers the entire lifecycle of products, providing a comprehensive view rather than focusing solely on single aspects.
- Decision-Making: Engineers utilize LCA to inform their design choices, promoting greener alternatives before products reach the market.
- Benchmarking: This assessment allows for the comparison of similar products in terms of their environmental performance, facilitating better choices by consumers and industries.
This process often involves several steps including goal definition, inventory analysis, impact assessment, and interpretation of results. Implementing LCA encourages industry leaders to prioritize sustainability in product design, thus minimizing overall ecological footprints.
Sustainability Audits
Sustainability audits are assessments conducted to evaluate how well an organization’s practices meet sustainable goals. These audits focus on resource usage, waste management, and compliance with regulatory standards. The benefits are manifold:
- Identifying Key Areas for Improvement: Audits highlight areas with significant potential for reducing waste or increasing efficiency.
- Regulatory Compliance: Regular audits ensure that organizations adhere to local and global environmental regulations, minimizing risk of penalties.
- Stakeholder Engagement: Sharing audit findings can enhance transparency with stakeholders, leading to improved trust and collaboration.
An effective sustainability audit typically involves a thorough examination of operational practices, including energy consumption, water use, and recycling efforts. Often, these audits lead to actionable recommendations that enable firms to enhance their sustainability credentials.
The capacity to measure and assess sustainability not only enhances compliance but also fosters innovation and cost-savings in mechanical engineering.
Ending
The conclusion of this article encapsulates the significance of integrating sustainability within mechanical engineering. It brings together various threads discussed throughout, highlighting the essential role that this field plays in fostering a greener future. Understanding sustainability is no longer optional for engineers; it is pivotal in redefining how we approach designing and manufacturing products.
One key element is the economic benefits achieved through sustainable practices. Companies adopting these principles often experience increased efficiency, reduced waste, and lower operational costs. Furthermore, there is a growing market demand for sustainable products, creating a competitive advantage. As such, businesses are incentivized to innovate while being eco-conscious.
Another crucial aspect is the societal impact. By prioritizing sustainable engineering, we contribute to public health and environmental well-being. This creates a ripple effect where communities thrive, leading to a more balanced ecosystem.
In considering education and the future, integrating sustainability concepts into engineering curricula is critical. Preparing the next generation of engineers to think critically about the environment can drive change. Institutions must foster a culture of collaboration between academia and industry to facilitate this.
Overall, the pathway to sustainable mechanical engineering is multi-faceted. It involves technological advancement, regulatory support, and a commitment to continuous improvement. Engineers must embrace these challenges, pushing the boundaries of innovation while staying grounded in the principles of sustainability. By doing so, they can not only meet today's demands but also ensure a viable planet for future generations.
Key Elements of Effective References
- Credibility: Citing reputable sources enhances the overall trustworthiness of the content. Peer-reviewed journals, academic publications, and recognized institutions should be prioritized.
- Diversity of Sources: A mix of sources, including books, articles, and research papers, broadens the perspective on a given issue. This is particularly relevant in a dynamic field like sustainable mechanical engineering, where knowledge is continually evolving.
- Current Information: Sustainability is a rapidly changing domain. Thus, up-to-date references are crucial to reflect the latest advancements and trends. Using sources from the last few years is ideal.
- Clear Citation Style: Consistency in citation style helps in maintaining clarity and organization. Whether using APA, MLA, or another format, clear indications help the reader follow the reference trail easily.
Benefits of Incorporating References
- Enhances Learning: For students and researchers, references create pathways for further exploration and knowledge acquisition.
- Supports Best Practices: In professional settings, references guide engineers and decision-makers in identifying best practices and emerging trends in sustainability.
- Fosters Collaboration: A well-referenced article can encourage engagement with others in the field, leading to potential partnerships or collaborative research efforts.