Understanding the Complexity of Oxidized Metal
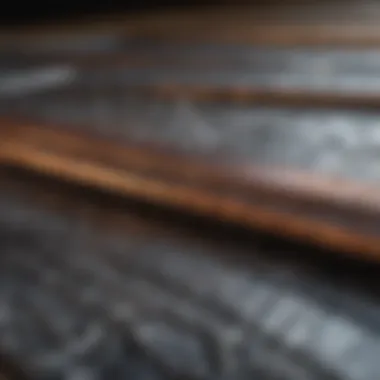
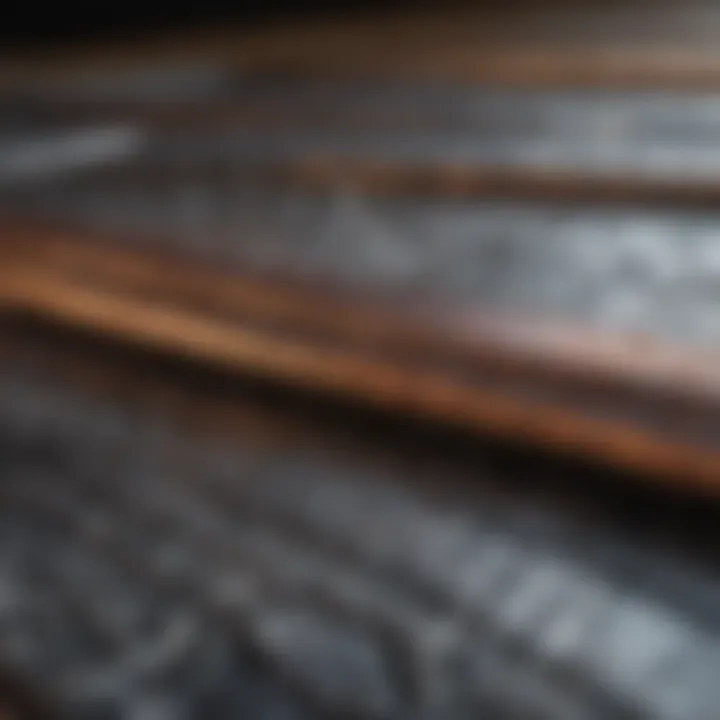
Intro
The realm of oxidized metal is a fascinating one, a blend of chemistry and artistry that captures a world where materials transform, adapt, and find new purpose. Understanding this subject not only requires a dive into chemistry but also an appreciation for its applications in everyday life and specialized domains. From architectural adornments to technological innovations, oxidized metals can be found gracing our environment in unexpected yet vital ways.
Oxidation, at its core, refers to the reaction of a metal with oxygen, leading to a range of transformations that are both physical and chemical. This process may result in protective layers, alterations in appearance, or even changes in functionality. As materials scientists and enthusiasts, our job is to dissect the nuances of this fascinating phenomenon—what makes a metal susceptible to oxidation, how these reactions unfold, and what implications they hold across various sectors.
As we journey through this exploration, readers will encounter essential findings that reflect the significant role oxidized metals play. This article aims to bridge the gap between complex scientific discussions and practical applications, illuminating the complexities of oxidized metal through relatable examples and detailed explanations. From the artwork of ancient civilizations to the tech advancements of our time, the implications of oxidation are more profound than one might assume.
Prelims to Oxidized Metal
Metal oxidation is a captivating and complex phenomenon that pervades various sectors, from art preservation to industrial manufacturing. Understanding this topic not only paints a clearer picture of the metal's lifespan but also illuminates practical applications and solutions to counteract its downsides. The study of oxidized metal helps individuals appreciate the balance between nature's processes and human ingenuity. It can guide engineers, artists, and conservationists alike in their respective fields, making it a universally relevant subject for a multitude of audiences.
Definition and Overview
Oxidation is fundamentally a chemical process where metal reacts with oxygen, resulting in the formation of an oxide. This process is not merely a nuisance; rather, it is part and parcel of the life cycle of metals. Unlike what popular belief suggests, oxidized metal can take many forms, each with a unique structure and properties. For instance, rust, which is iron oxide, signifies metal degradation but can also serve as a striking feature in art.
Chemical Basis of Oxidation
Understanding the chemical basis of oxidation is fundamental to grasping the broader implications of oxidized metals in both natural and industrial contexts. Oxidation is not a mere chemical curiosity; it’s a process that governs the durability, functionality, and aesthetic value of metals across various applications. Throughout this section, we will explore the core mechanisms of oxidation, emphasizing its significance in oxidation-reduction reactions as well as the electrochemical processes that drive these reactions.
Oxidation-Reduction Reactions
At the heart of oxidation is the oxidation-reduction reaction, commonly referred to as the redox reaction. This involves a transfer of electrons between two substances, comprising two critical processes:
- Oxidation: This is where a substance loses electrons, leading to a rise in its oxidation state. For example, when iron rusts, it reacts with oxygen in the presence of water, forming iron oxide.
- Reduction: In contrast, this occurs when a substance gains electrons, resulting in a decrease in its oxidation state.
Redox reactions are vital across different fields. In the context of environmental chemistry, these reactions can dictate the fate of contaminants in soil and water. The outcomes of such reactions may lead to the degradation of essential materials or, on the flip side, the recovery of valuable resources. For instance, during waste water treatment, the reduction of harmful substances can lead to the formation of less toxic materials, showcasing the dual nature of oxidation processes.
Additionally, oxidation-reduction reactions also play a role in energy production. Take batteries as an example. They work on the principle of redox reactions, where chemical energy is converted into electrical energy. This not only highlights the practical applications of oxidation but also shows its influence on technology and daily life.
Electrochemical Processes
Delving deeper into oxidation, electrochemical processes emerge as a critical aspect of how oxidation occurs. These processes can be observed in various scenarios, from simple batteries to complex corrosion phenomena. The essence of electrochemical processes lies in the interaction between an electrode and electrolyte, leading to electron transfer and subsequently, oxidation or reduction reactions.
In a corrosion scenario, such as the oxidation of iron, we see the potential for electrochemical activity:
- Anode Reaction: At the anode, iron undergoes oxidation, losing electrons and forming iron ions.
- Cathode Reaction: At the cathode, the reduction of available species occurs, often involving oxygen and water to form hydroxide ions.
Through these pathways, one can understand how protecting metals from oxidation is not just a surface-level endeavor but a multifaceted dance of chemical reactions.
Proper management of metal exposure to oxidizing agents, like moisture and pollutants, is key in preventing corrosion.
In summary, the chemical basis of oxidation extends beyond mere theoretical significance; it influences practical applications in technology, art, and conservation. Recognizing the nuances of oxidation-reduction reactions and electrochemical processes can lead to innovative approaches in material preservation and exploitation. When researchers and professionals consider these factors, they gain essential insights that pave the way for sustainable practices in myriad fields.
Forms of Oxidized Metal
Understanding the various forms of oxidized metal is crucial for several reasons. Firstly, it sheds light on how these transformations impact material properties, which can be particularly important in industrial applications. Secondly, recognizing the different oxidative products enables better strategies for preservation and conservation in art and historical artifacts. Thirdly, each form of oxidized metal carries unique aesthetic and functional attributes, affecting how they are used in everyday applications. This section delves into three main forms of oxidized metal: rust, patina, and aluminum oxides.
Rust: Iron Oxide
Rust, often encountered as the reddish-brown flaking on iron surfaces, is primarily iron oxide, formed when iron reacts with oxygen in the presence of water. The chemical reaction that produces rust can be simplified as:
[ 4Fe + 3O_2 + 6H_2O ightarrow 4Fe(OH)_3 ]
This reaction reveals that, for rust to form, moisture is a key factor, making it an ever-present concern in various locales, especially those with high humidity.
"Rust is like a slow thief, gradually stealing away the integrity of structures we often take for granted."
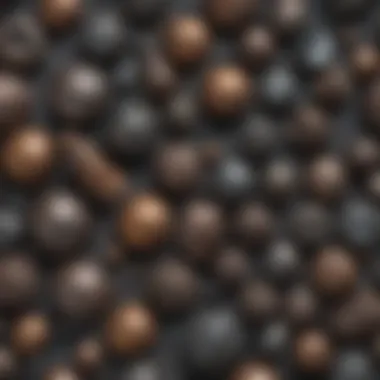
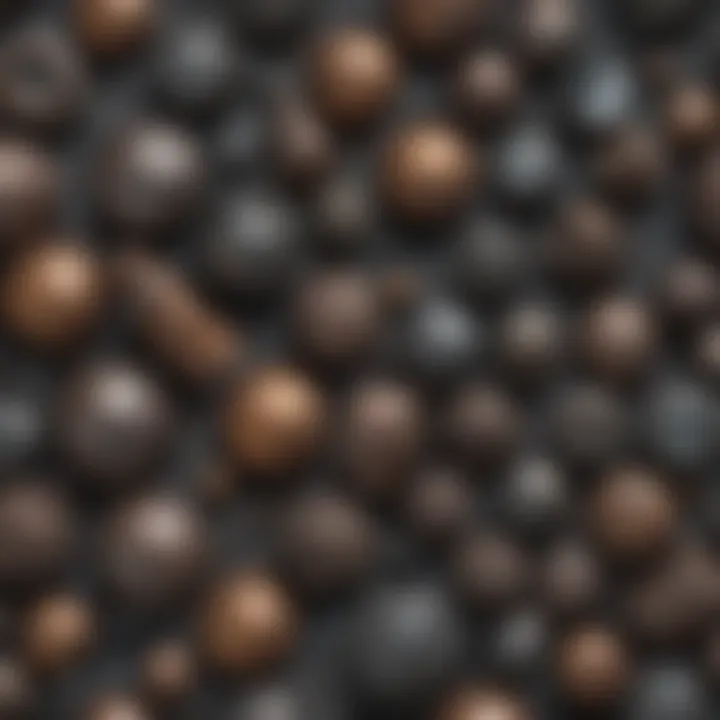
The presence of rust not only signifies material degradation but also serves as a strong indicator of environmental conditions. It connects the state of the metal with its surroundings. Understanding rust leads to better prevention methods. For instance, applying protective coatings or using alloys can mitigate the rusting process.
Patina: Copper Corrosion Product
Patina is a fascinating phenomenon that emerges when copper is exposed to atmospheric elements. Over time, this ancient metal evolves to acquire a protective layer, primarily composed of various copper compounds like copper carbonate and copper sulfate. This layer, often seen as a greenish film, serves a dual purpose: aesthetic enhancement and protective coating.
The patina process is not merely about turning green; it symbolizes the material’s adaptability and longevity. This transformation can take years to achieve, ensuring that the underlying metal remains safe from further corrosion. Artists and architects often celebrate patina for its unique beauty, making it a sought-after feature in buildings and sculptures.
Much of the charm of historical artifacts rests on their patina. The color variations—ranging from blues to vibrant greens—tell a story of the environment and time elapsed since the material's creation. Despite its appealing aspect, one must be cautious; excessive patina can signal deterioration if it leads to structural weaknesses.
Aluminum Oxides
Aluminum oxide represents a crucial form of oxidized metal that plays an essential role across various industries. When aluminum is exposed to air, it rapidly forms a thin layer of aluminum oxide, resulting in a protective barrier that stops further oxidation. This quality not only enhances durability but also renders aluminum suitable for applications where strength and resilience are paramount.
The process of anodizing aluminum emphasizes this oxidization, allowing for thicker aluminum oxide layers to be formed artificially. This treatment enhances corrosion resistance, wear resistance, and can even allow for dyeing in vibrant colors. The surface finish provided by anodizing is prevalent in areas such as
- Aerospace components
- Architectural features
- Consumer electronics
Overall, aluminum oxides are vital due to their beneficial properties that contribute significantly to maintaining both functional integrity and aesthetic appeal in numerous applications. Recognizing this form alongside rust and patina allows a better grasp of metal oxidation's broader implications in material science.
By understanding the distinct characteristics and situations of these three forms, readers can appreciate the complexity involved in the oxidation processes. Keeping in mind the impact of environmental factors, such as humidity and temperature, also allows us to predict metal longevity and devise better conservation strategies.
Environmental Influences on Metal Oxidation
Understanding the environmental influences on metal oxidation sheds light on a variety of factors that ultimately shape the durability and lifespan of metal materials. This exploration is crucial, as it dives into how different conditions can accelerate or inhibit the oxidation process. The interaction between environmental elements and metals is not merely a scientific curiosity; it influences industries ranging from construction to art preservation. The examination of such virtues clarifies our comprehension of material science and facilitates the development of improved protective measures against corrosion.
Humidity and Moisture
Humidity and moisture are arguably among the leading contributors to the oxidation of metals. When metals are exposed to humid air, especially at elevated temperatures, the rate of oxidation can shoot up. Water acts as a facilitator in the electrochemical reactions that lead to rust formation. For instance, iron reacts with oxygen and water, forming iron oxides, or rust, which can weaken the structure over time.
The importance of moisture cannot be overstated. For example, in coastal regions, where saltwater and air collaborate to create harsh conditions, metals can corrode far more quickly compared to dry inland areas. This makes it essential for industries, particularly marine and construction, to recognize these intricate interactions. Some metals, like aluminum, develop a protective oxide layer when exposed to moisture, slowing the progression of corrosion, while others, like iron, are vulnerable to further deterioration.
"Understanding how various atmospheres interact with metals can aid in predictive maintenance, preventing costly repairs down the line."
Temperature Factors
Temperature variations also play a significant role in metal oxidation. High temperatures can enhance the rate of chemical reactions, thereby accelerating the oxidation process. In extreme heat, some metals may lose their protective layers more quickly than they would at cooler temperatures. For instance, steel exposed to high heat can undergo thermal oxidation, which changes its microstructure and can lead to unexpected failures.
Conversely, low temperatures can mitigate oxidation risks, but prolonged exposure to cold can also lead to moisture accumulation, which creates a different set of problems. It’s a delicate balance, as industries must monitor both extremes to ensure longevity of metal. In manufacturing processes, understanding the thermal behavior of different metals can guide decisions in material selection and processing.
Pollutants and Chemical Exposure
The presence of pollutants and chemicals in the environment remains another significant factor affecting metal oxidation rates. Industrial emissions, acid rain, and other environmental contaminants can interact with metals, creating aggressive corrosion pathways. For example, sulfur dioxide in the air can combine with water to form sulfuric acid, which can significantly speed up corrosion, leading to what is commonly referred to as "acid rain corrosion."
Moreover, certain chemicals, like chlorides and sulfides, can exacerbate the characteristic rusting process in materials like iron and steel. Understanding these elements is crucial for industries, as employing proper measures to mitigate exposure can save substantial costs in repairs and maintenance.
By grasping these environmental elements, from humidity to temperature to pollutants, professionals can develop more effective strategies for protecting metals against oxidation. Such considerations are vital not only for sustaining the integrity of materials but for advancing the collective knowledge within this essential field of study.
Applications of Oxidized Metal
The study of oxidized metal extends beyond mere scientific curiosity. It interfaces with various fields, offering practical solutions and artistic inspiration. Understanding these applications is vital because they reflect how society interacts with materials on multiple levels, illuminating their significance in art, industry, and conservation efforts.
In Art and Architecture
The aesthetic appeal of oxidized metal cannot be overstated. Artists and architects alike have harnessed the unique qualities of oxidized surfaces to enhance their creations. Take, for example, copper that has formed a beautiful green patina over time. This transformation does not merely signify decay; rather, it embodies a dialogue between nature and human creativity.
In modern architecture, oxidized metals such as Corten steel have become quite popular. These metals develop a rich, rusty appearance that blends beautifully with natural landscapes. The outer layer acts as a protective coat, minimizing further corrosion while providing an attractive finish. Artists like Richard Serra use such materials to juxtapose the solidity of metal with the fluidity of environmental elements, creating thought-provoking installations.
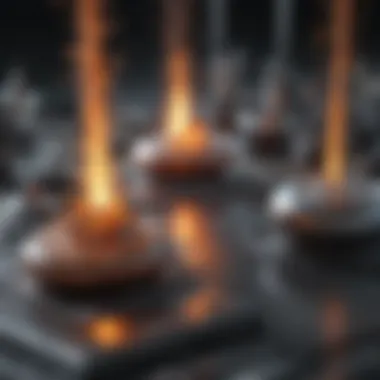
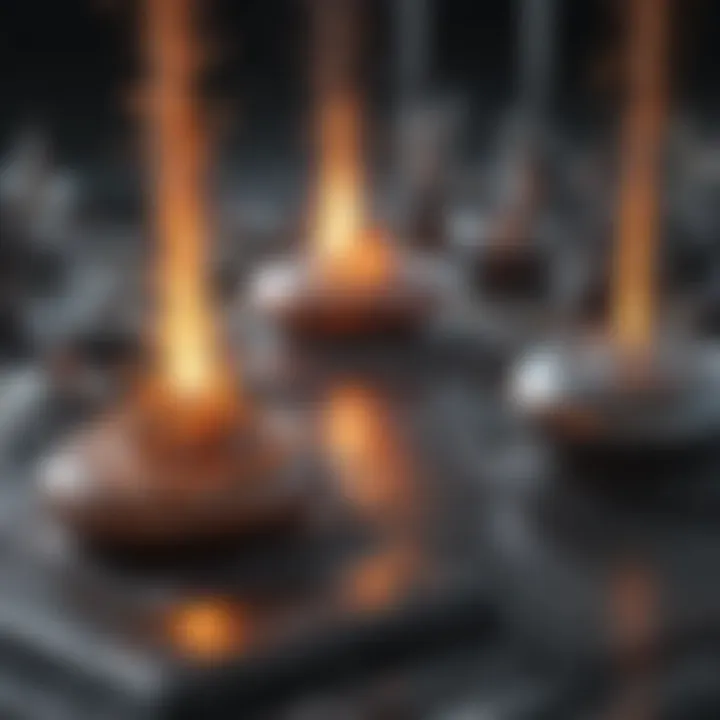
Industrial Uses
Beyond aesthetic appeal, oxidized metals play critical roles in various industries. Rust, often seen as a bane, can be surprisingly functional when understood and controlled. For instance, iron oxides are utilized in producing pigments for paints. The shades derived from these oxides are not only stable but also provide a classic look preferred for certain applications.
Furthermore, aluminum oxides, resulting from oxidization, are valuable in manufacturing. They possess an array of beneficial properties such as strength and durability. These traits make them ideal for aerospace applications and in creating lightweight components that can withstand harsh environments.
Important Considerations:
- Economic Factors: Oxidized materials can reduce costs in certain applications by enhancing longevity and reducing the frequency of replacements.
- Safety Concerns: It is crucial to manage and mitigate the risks of oxidation in certain industries to ensure compliance with safety regulations and to prevent structural failures.
In Heritage Conservation
Preserving historical and cultural landmarks often necessitates a nuanced understanding of oxidized metal. Many ancient structures feature metals that have oxidized over centuries, contributing to their character. Conservationists face the challenge of maintaining these structures without compromising their authenticity.
For instance, the Statue of Liberty has notably developed a thick layer of patina that not only signifies its historical journey but also serves a protective purpose. Understanding the science behind oxidation helps conservators decide whether to leave oxidized surfaces intact or restore them.
Moreover, modern conservation techniques often incorporate protective coatings. These can slow down oxidation while allowing the original material's character to shine through. Thus, a balance must be struck between preservation and ongoing deterioration, while respecting the historical significance of the materials involved.
The intersection of art, industry, and conservation in the context of oxidized metal exemplifies its multifaceted utility in contemporary society. Understanding these applications enhances our appreciation of both the materials we often take for granted and the craftsmanship that employs them.
In essence, the applications of oxidized metal offer a complex tapestry of interactions with our environment and culture, calling for continued exploration and innovation in its study and utilization.
Challenges of Metal Oxidation
Understanding the challenges of metal oxidation is fundamental in various disciplines, primarily where metal is a core component of the infrastructure or technology. The ramifications of oxidation can extend far and wide, affecting everything from architectural integrity to economic viability. Recognizing these challenges allows stakeholders, such as researchers, engineers, and conservationists, to formulate effective mitigation strategies.
Material Degradation
Material degradation due to oxidation poses significant issues for the longevity and functionality of metals. Over time, oxidization leads to a breakdown of metal structures, diminishing their strength and reliability. For instance, iron exposed to moisture will develop rust, which can compromise structural integrity in buildings or bridges.
Consider the case of old train tracks. If oxidation is allowed to progress unchecked, it leads to rail fractures that could cause derailments. In environments like marine settings, the conditions are even harsher. Saltwater accelerates oxidation, leading to rapid corrosion of metals such as steel. This not only necessity higher maintenance costs, but it also requires more frequent replacements, ultimately impacting the performance of transportation infrastructures.
Furthermore, the aesthetic appeal of metals can deteriorate due to oxidation. A beautiful, shiny bronze sculpture could lose its charm if it develops a green patina. In such cases, while oxidation may protect the metal from further degradation, the visual impact can be undesirable.
Economic Implications
Economic implications stemming from metal oxidation are multifaceted and often intertwine with various industries. The costs associated with corrosion management can be staggering. According to studies, the annual cost of corrosion around the globe runs into hundreds of billions of dollars. This covers not only the replacement of oxidized metal components but also the loss of productivity resulting from systems downtime.
Moreover, the economic burden transcends just repairs; it can ripple through supply chains. When automotive manufacturers face issues related to metal degradation in their supply materials, the consequences trickle down - impacting production schedules and profitability. Consumers, too, may bear the financial brunt, either through higher prices or through diminished product longevity.
"Inadequate attention to corrosion management can lead to risks that threaten not just metal structures, but entire economies."
Innovative Solutions and Advances
In the ever-evolving field of materials science, addressing the challenges associated with oxidized metal is paramount. The development of innovative solutions and advances in this area not only help mitigate the negative impacts of oxidation, but also open new avenues for enhanced performance in various applications. These solutions are crucial in preserving structural integrity and aesthetic appeal in construction, art, and technology.
Protective Coatings
Protective coatings are gaining traction as one of the primary methods to combat oxidation. Their main function is to serve as a barrier between the metal surface and the environmental factors that promote oxidation. These coatings can be engineered from various materials, including organic polymers and inorganic compounds.
For instance, polysiloxane coatings offer excellent resistance to both moisture and chemicals. Such coatings not only prevent the rusting of iron but also improve the lifespan of aluminum and copper surfaces. Furthermore, advanced technologies in coating application, like plasma spraying and electroplating, have made it possible to achieve thinner layers with more durability, reducing material waste.
The choice of coating must take into account several considerations, such as:
- Environmental Conditions: Different areas may require different coating materials, depending on humidity, temperature, and exposure to pollutants.
- Cost Efficiency: While some advanced coatings may come with higher upfront costs, they can save substantial amounts in terms of long-term maintenance and replacement.
- Sustainability: Eco-friendly coatings are increasingly being developed, encouraging industries to adopt solutions with minimal environmental impact.
The right protective coating can significantly extend the service life of metal components, making it an essential aspect of modern engineering practices.
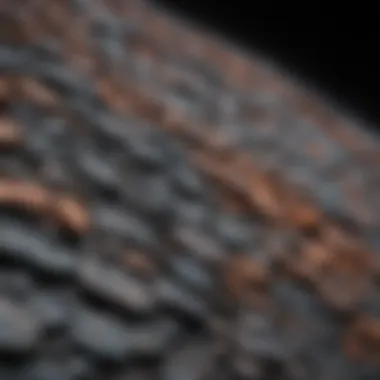
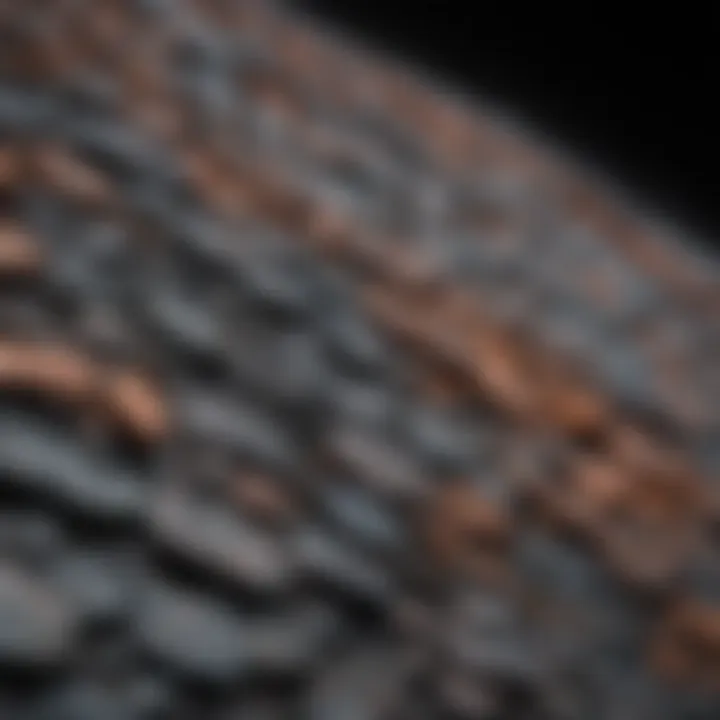
Corrosion Inhibitors
Corrosion inhibitors represent another innovative approach that has garnered attention in recent years. These substances are added to the environment surrounding metals to reduce the rate of corrosion. Their effectiveness lies in their ability to form a thin protective layer on the surface of the metal, thereby impeding the electrochemical reactions that lead to oxidation.
There are two primary categories of corrosion inhibitors:
- Anodic Inhibitors: These substances primarily work by shifting the electrochemical potential of the metal to minimize reactions that lead to corrosion.
- Cathodic Inhibitors: These prevent reduction reactions, which are equally crucial in the corrosion cycle.
An example of a commonly used corrosion inhibitor is zinc phosphate, often utilized in paints and coatings. This compound can help safeguard steel structures such as bridges and buildings from the effects of moisture and contaminants. Moreover, recent research has focused on green corrosion inhibitors derived from plant extracts, showing promising results in maintaining both efficacy and sustainability.
Future Directions in Oxidized Metal Research
The exploration of oxidized metal encompasses a vast array of topics, stretching from the origins of corrosion to its applications in various industries. As we look ahead, it becomes increasingly vital to consider the future directions of this research. This section delves into why understanding emerging technologies and sustainability considerations figures prominently in enriching our grasp of oxidized metals.
Emerging Technologies
One cannot ignore the revolutionary potential of emerging technologies in the realm of oxidized metals. Novel advancements enable us to not only enhance existing processes but also develop entirely new methodologies for studying and utilizing these materials. For instance, researchers are employing nanotechnology to create better protective coatings that minimize oxidation without significantly altering the metal's appearance or structure. These thin films can dramatically extend the lifespan of metals used in infrastructure, vehicles, and consumer goods.
Additionally, AI and machine learning are playing significant roles in metallurgical research. These technologies can analyze vast datasets more swiftly than ever before, uncovering patterns and predicting corrosion rates based on various environmental factors. In particular, the ability to assess real-time data can lead to smarter maintenance strategies that save resources and maximize the longevity of metal products.
The intersection of 3D printing and oxidized metals also holds promise. With the potential to customize metal components on demand, manufacturers can create pieces that are specifically designed to resist oxidation. For example, when producing unique parts for spacecraft or marine applications, engineers can apply coatings that are tailor-made to withstand harsh conditions. This is not only efficient but reduces waste, as it allows for precise machining rather than broad casting processes.
Overall, innovation in these areas can bring practical advantages, driving both efficiency and sustainability in industries that rely on oxidized metals.
Sustainability Considerations
The question of sustainability in metal research is not merely a buzzword; it is becoming increasingly crucial. As communities and industries confront climate change and resource depletion, strategies for sustainable metal usage are being prioritized.
One key aspect is the development of recycling techniques specifically tailored for oxidized metals. Traditionally, rusted or oxidized materials were considered waste or at best, a nuisance. However, technologies are emerging that can recycle and purify these metals, turning what was once termed garbage into useful materials. The process not only recovers valuable metals but also reduces energy consumption compared to primary metal extraction.
Additionally, utilizing eco-friendly corrosion inhibitors is gaining traction. Unlike conventional inhibitors that could be harmful, new organic compounds sourced from natural products are showing promise in reducing oxidation. This evolution towards greener chemistry is reshaping our approach to preventing corrosion.
Incorporating life cycle analysis in metal production processes can further reinforce sustainability. By evaluating environmental impacts from extraction through disposal, manufacturers can identify and implement practices that lessen their carbon footprint. Ultimately, the goal is to find a balance where economic feasibility meets environmental responsibility.
The future of oxidized metal research is not just about understanding corrosion but also about finding harmonious solutions that serve academic, industrial, and environmental interests.
In sum, the future directions in oxidized metal research hold the key to many pressing challenges we face today. From leveraging emergent technologies to prioritizing sustainability, the ongoing study of oxidized metal is essential not only for advancing materials science but also for forging a more sustainable future.
Finale
The world of oxidized metal is not without its intricacies, and our exploration unfolds a myriad of insights that shed light on both the scientific and practical aspects of this phenomenon. First and foremost, understanding the process of oxidation allows us to appreciate not just the degradation of metals but also their transformation into aesthetically and functionally valuable forms. For instance, patinas on copper sculptures do not merely symbolize decay but are often seen as enhancing the beauty of the artwork itself. This duality is one of the key takeaways from our article: oxidation can be both a foe and a friend.
Moreover, the applications discussed throughout, ranging from industrial uses to their role in conservation, underline the significance of oxidized metals in various domains. Recognizing that these materials can also carry socioeconomic implications is vital. Businesses, after all, must grapple with economic implications of metal corrosion, which can lead to substantial repair costs if left unchecked.
A well-rounded understanding of oxidized metals supports better decision-making in design and sustainability practices.
Additionally, given the environmental stressors that accelerate metal oxidation, continuing research is critical. As urban environments become increasingly polluted, the challenges associated with oxidized metals will only intensify. Tackling these issues through innovative solutions, as highlighted earlier, equips professionals with better tools for conservation and restoration—strategies that are becoming essential in our rapidly changing world.
In summary, the study of oxidized metals serves as a reminder of the delicate balance between deterioration and enhancement. By maintaining a focus on ongoing research and developments, we prepare ourselves to better manage the long-term effects of oxidation on metal. Recognizing the nuances of these materials opens the door to deeper appreciation and understanding, ensuring that both professionals and enthusiasts can contribute to a future where metal arts and technologies flourish in harmony with the natural world.
Summary of Key Insights
The examination of oxidized metal reveals myriad insights:
- Oxidation as Versatile Process: It can lead to deterioration or artistic enhancement, depending on context.
- Material Characteristics: Different metals show various behaviors when subjected to oxidizing agents, such as humidity and pollutants.
- Economic Impact: The costs associated with repairing corroded materials highlight the importance of effective management.
The Importance of Ongoing Study
Further study in this field is essential for several reasons:
- Adaptation to Changes: New technologies can offer improved methods for combating oxidation, which is crucial as environmental conditions change.
- Sustainable Practices: Ongoing research can help find ways to sustainably utilize oxidized metals in art and industry.
- Awareness of Consequences: Understanding the implications of oxidized metal can lead to better conservation efforts, protecting both artifacts and modern constructions.
Engaging with these themes not only enhances technical knowledge but also promotes an understanding of the broader environmental realm in which oxidized metals exist.