Exploring Microfluidic Mixing Devices: Insights and Innovations
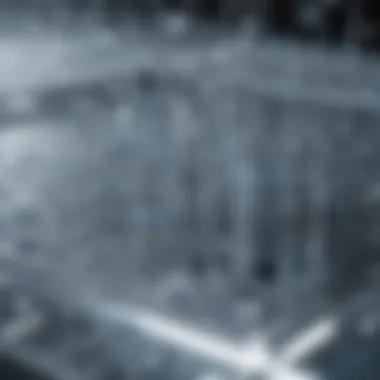
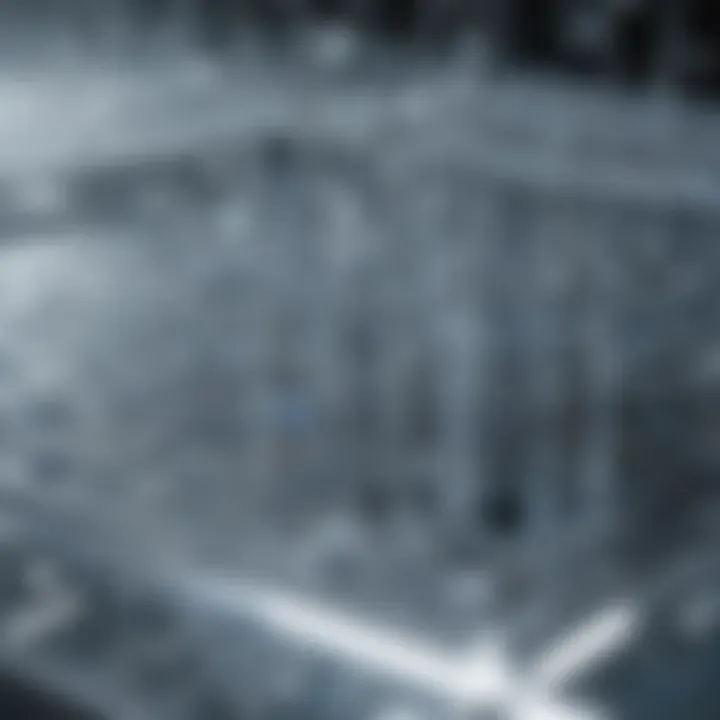
Intro
Microfluidic mixing devices represent a significant advancement in the merging of small volumes of fluids for various scientific and industrial applications. These devices leverage the principles of fluid dynamics at the microscale to create efficient, controlled mixing environments. Understanding their design, functionalities, and wide-ranging applications sheds light on their crucial role in diverse fields like biology, chemistry, and engineering.
Historically, the development of microfluidic technology derived from a need for precise control over minute quantities of liquids. This demand arises from advancements in areas such as drug delivery, diagnostics, and chemical synthesis. As we continue to push the boundaries of science, microfluidic mixing devices are becoming increasingly important due to their ability to enhance reaction rates and improve the reproducibility of experiments.
In this article, we will explore the key findings, including critical results and discussions regarding microfluidic mixing mechanisms, design principles, applications, and future prospects. Together, these elements will provide a rich narrative that not only informs but also engages students, researchers, educators, and professionals involved in the field.
Preamble to Microfluidics
The realm of microfluidics stands at the crossroads of multiple disciplines, merging principles from physics, chemistry, and engineering. This introduction to microfluidics lays the groundwork for understanding its crucial role in modern scientific inquiry and technological innovation. Microfluidics refers to the manipulation of fluids on a microscopic scale, often through channels that are only a few micrometers in width. This technology has transformed how experiments are conducted, enabling precise control over fluid environments.
Microfluidic systems hold immense potential by allowing researchers to conduct biochemical reactions with reduced volumes of reagents. This results in not only cost savings but also a decrease in waste production. Understanding microfluidics means grasping how these devices resemble miniature laboratories, capable of performing complex tasks such as mixing, heating, and biochemical analysis in a compact size. Such capabilities are particularly beneficial in fields ranging from biomedical research to environmental monitoring.
Defining Microfluidics
Microfluidics encompasses the study and application of fluid flow dynamics in systems where dimensions are on the micrometer scale. In this context, “micro” refers to the size (1 millionth of a meter), but the implications are vast. Typically, the flow rates observed in microfluidics are very low, leading to unique behaviors of fluids that differ from those observed in larger systems. This discipline allows for greater control over reaction conditions which, in turn, can lead to enhanced performance in various applications. The integration of microfluidic technology with lab-on-a-chip devices has enabled increased automation and miniaturization of experiments, streamlining workflows in ways that are feasible only at micro scales.
The Significance of Mixing in Microfluidic Systems
Mixing is a fundamental process in microfluidic systems. Effective mixing ensures that reactants combine evenly, which is crucial in applications such as chemical synthesis, diagnostics, and drug delivery. In microfluidic environments, traditional mixing methods often prove inefficient due to the low Reynolds numbers involved, which lead to laminar flow conditions. As a result, achieving homogenous mixing requires innovative strategies.
Mixing Mechanisms
- Passive Mixing: This mechanism relies on the geometry of the channel to promote mixing without external energy. The design often includes serpentine shapes or obstacles that induce chaotic advection, leading to enhanced contact between fluid streams.
- Active Mixing: This relies on external forces or devices, such as magnetic fields or ultrasound, to stir the fluids. Active mixing provides faster and more uniform results compared to passive techniques, particularly when rapid mixing is necessary.
Understanding the principles of mixing in microfluidics is not just an academic exercise; it has real-world implications, influencing everything from drug formulation to biochemical assays.
As microfluidic devices continue to evolve, optimizing mixing processes remains a critical focus area, driving advancements across various applications. This foundational knowledge sets the stage for deeper exploration into the key principles, design considerations, and applications of microfluidic mixing devices.
Key Principles of Microfluidic Mixing
Microfluidic mixing is fundamental for the operation of various systems that utilize small volumes of liquids. This section discusses the key principles that underpin how mixing occurs in these devices. Understanding these principles is crucial for optimizing device design and performance. The flow of fluids in microchannels often differs significantly from conventional mixing methods due to the scale involved. Key elements such as fluid dynamics and the mechanisms employed for mixing play a vital role in achieving the desired outcomes.
Fluid Dynamics in Microchannels
Fluid dynamics in microchannels is a critical concept in microfluidics. At the microscale, viscous forces dominate over inertial forces. This condition alters how fluids behave compared to larger systems, leading to unique flow patterns. Laminar flow is typically observed, where fluids flow in parallel layers without significant mixing between them. This behavior necessitates highly effective mixing techniques, as the strong laminar flow can prevent adequate combination of different fluids.
In microchannels, the Reynolds number is usually lower than one. Thus, even a small change in flow rate can cause substantial shifts in the flow regime. Understanding these dynamics aids in strategizing how to facilitate mixing through design variations, such as changes in channel width and height.
Mixing Mechanisms
Mixing in microfluidic devices generally falls into two categories: passive mixing and active mixing. Each type of mixing mechanism brings unique advantages and is suitable for differing applications.
Passive Mixing
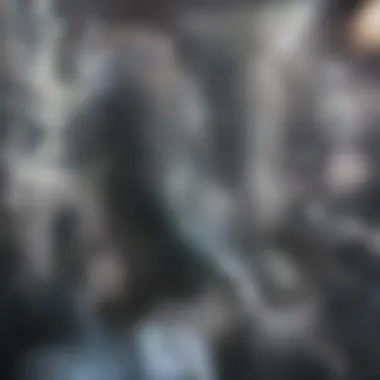
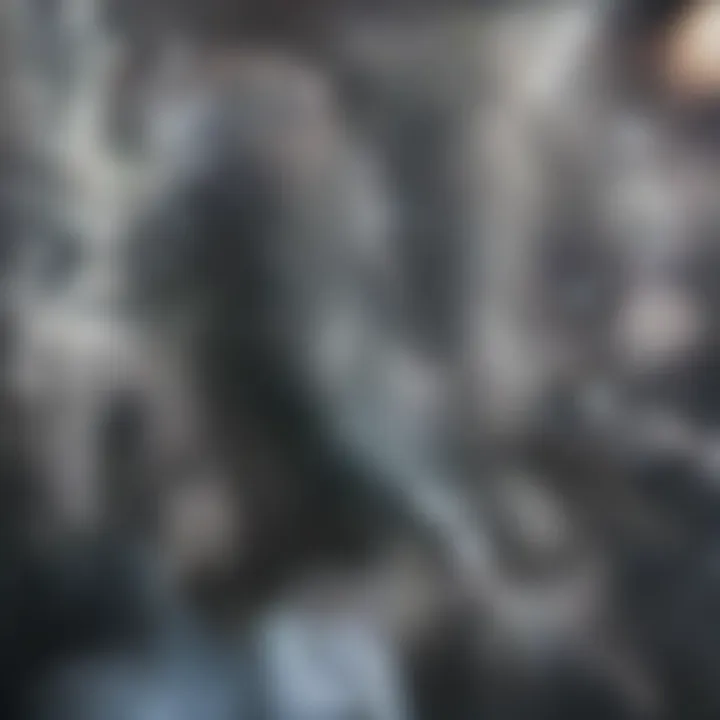
Passive mixing employs the inherent properties of fluid flow without the need for external energy input. This approach is characterized by its simplicity and low cost. One common aspect of passive mixing is the design of channel structures that promote chaotic advection. Features such as zigzag paths or constrictions increase interaction between fluids, encouraging more thorough mixing.
The key characteristic of passive mixing is the reliance on geometry rather than mechanical devices. As the fluids travel through constricted areas or along intricate geometries, they are forced to interact more. This method is beneficial for applications where a gentle mixing process is essential. However, the chief disadvantage is that passive mixing can be slower and may not achieve as thorough mixing as active methods.
Active Mixing
Active mixing employs external forces or mechanisms to enhance the mixing process. This can involve the use of electric fields, magnetic fields, or mechanical pumps. One significant aspect of active mixing is its ability to achieve rapid and homogenous mixing in a short period. In scenarios requiring precise control over mixing rates and outcomes, active methods are often the preferred choice.
The key advantage of active mixing is the efficiency with which it can integrate various reagents. It can also manage temperature and concentration gradients more effectively. However, these systems are often more complex and can be costlier due to additional components required. Active mixing may generate mechanical stress, which could impact delicate biological samples.
Design Considerations for Microfluidic Mixing Devices
Design considerations are critical to the success of microfluidic mixing devices. These devices play a key role in numerous applications, and their effectiveness can largely be attributed to their design. It is essential to understand the interaction between fluid dynamics, channel geometry, material selection, and flow control to create effective mixing solutions.
Channel Geometry
The geometry of the channels in microfluidic devices significantly affects mixing efficiency. Altering the shape and dimensions of the channels can create various flow patterns, influencing how fluids interact.
- Channel Width and Depth: Narrower channels can enhance shear flows and increase mixing rates as they force fluids together more effectively. However, if channels are too narrow, it may lead to pressure drop issues.
- Channel Design: Different geometrical designs, such as serpentine, zigzag, or branched channels, can generate unique flow patterns. This increases the turbulence and contact area between mixed fluids.
- Surface Roughness: Textured surfaces within channels can also enhance mixing by promoting chaotic advection, which helps to homogenize fluids at low Reynolds numbers.
Considering these factors can lead to improved mixing performance in multiple applications, from diagnostics to drug delivery.
Material Selection
Choosing the appropriate materials for constructing microfluidic devices is essential. Materials influence various factors, such as chemical compatibility, durability, and thermal properties.
- Polymeric Materials: Common choices like polydimethylsiloxane (PDMS) are popular due to their flexibility and optical transparency. However, PDMS can absorb small molecules, which can affect experimental results.
- Glass and Silicon: These materials are chosen for their chemical resistance and thermal stability, making them suitable for high-performance applications. However, the manufacturing process can be more complex and expensive.
- 3D Printing Materials: The advent of 3D printing introduces new possibilities using materials like acrylonitrile butadiene styrene (ABS) or thermoplastic elastomers (TPE). This allows for rapid prototyping and customization, but the longevity and bio-compatibility should be considered.
In summary, the choice of materials directly impacts the functionality and reliability of microfluidic devices and requires careful selection based on the intended application.
Flow Rate and Control
Managing the flow rate is another crucial aspect of microfluidic mixing design. Precise control can lead to enhanced mixing and reproducibility, which are vital for experimental accuracy.
- Pumping Techniques: Various methods such as syringe pumps, peristaltic pumps, and pressure-driven flow systems can be employed. Each method offers unique advantages depending on the setup and desired outcomes.
- Flow Rate Variability: Adjusting the flow rate alters the residence time of the reagents in the mixing zone. Optimal flow rates can enhance the mixing quality, while excessive speeds may lead to insufficient mixing.
- Microvalves: Incorporating microvalves allows for precise control of fluid flow and can be used to manipulate flow rates dynamically. This adaptability is especially important in complex experimental designs.
Careful attention to flow control is necessary to achieve robust mixing performance and increase the reliability of microfluidic devices in various research domains.
Applications of Microfluidic Mixing Devices
Microfluidic mixing devices are fundamental tools used across various scientific domains, enabling precise control over fluid flows at the microscale. These systems exhibit great potential due to their versatility and efficiency. Understanding their applications is crucial, as they directly impact advancements in multiple fields, including biomedical research, chemical synthesis, and environmental monitoring. Each application not only highlights the capabilities of microfluidic devices but also reveals their significance in solving complex scientific problems.
Biomedical Research
In biomedical research, microfluidic mixing devices are instrumental in the development of diagnostic tools and therapeutic techniques. The ability to manipulate small volumes of fluids makes these devices ideal for cell analysis, drug screening, and biomarker detection. For instance, researchers can use microfluidics to efficiently mix reagents and achieve rapid reactions that would otherwise take longer in conventional setups.
- Cell Analysis: Microfluidics allows for the study of single-cell behaviors, providing insights into cellular responses under various conditions.
- Drug Discovery: With enhanced mixing capabilities, researchers can swiftly evaluate the efficacy of a multitude of compounds against specific targets.
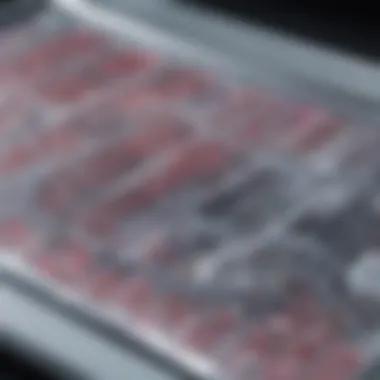
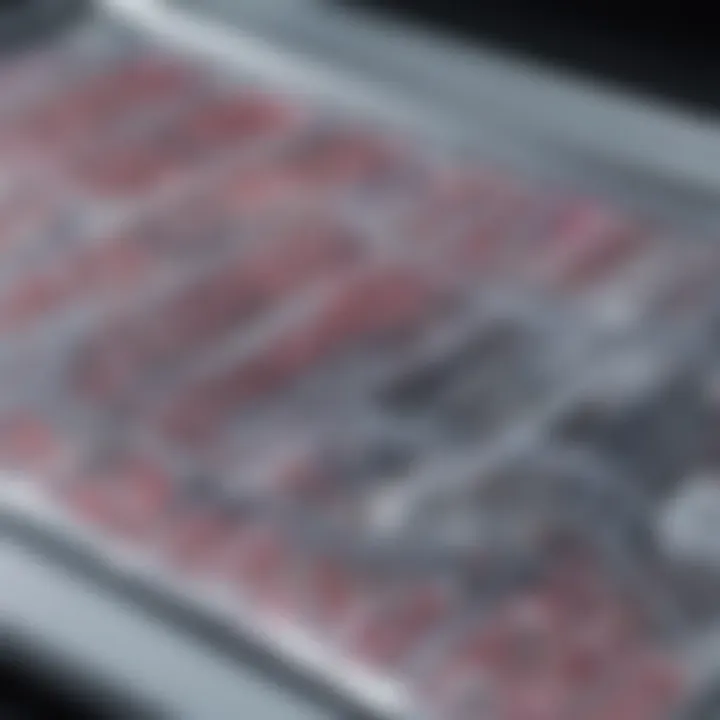
Importantly, microfluidic mixing devices reduce the need for larger volumes of reagents, which is both cost-effective and environmentally friendly. This capability streamlines processes and accelerates the pace of research.
Chemical Synthesis
Microfluidic mixing technologies are also transformative in chemical synthesis. Here, the precise control of reaction conditions leads to improved yields and enhanced selectivity of products. The integration of microfluidics allows chemists to conduct reactions that are difficult to manage on a larger scale.
- Continuous Flow Synthesis: Microfluidic systems enable continuous flow synthesis, which minimizes delays often seen in batch processing. This method can lead to faster production times.
- High-throughput Screening: The capability to conduct numerous synthesis reactions simultaneously increases efficiency and opens avenues for discovering novel compounds.
These advantages lead to a paradigm shift in how chemical synthesis is approached, promising not only increased productivity but also novel methods to create complex molecules.
Environmental Monitoring
Microfluidic mixing devices find their place in environmental monitoring by facilitating the analysis of water and air samples. These devices enable real-time detection of pollutants and toxins, which is essential for maintaining ecological balance and ensuring public safety.
- Pollutant Detection: The devices can detect trace levels of contaminants, providing invaluable data for environmental studies.
- Field Deployability: Microfluidic sensors can be deployed in remote locations, allowing continuous monitoring without the need for extensive laboratory setups.
Thus, their application in environmental monitoring is not only crucial for data collection but also empowers swift responses to environmental hazards.
In summary, the applications of microfluidic mixing devices highlight the intersection of technology and science, offering innovative solutions across various fields.
Recent Advancements in Microfluidic Mixing Technology
The evolution of microfluidic mixing technology is marked by significant innovations and enhancements that improve both functionality and efficiency. As microfluidic systems gain prominence in various scientific fields, understanding these recent advancements becomes essential. They offer novel strategies to optimize mixing processes, addressing challenges faced previously in the design and implementation of microfluidic devices.
Innovations in Design
Recent advancements in the design of microfluidic mixing devices have introduced novel geometries and layouts that enhance mixing efficiency. Multi-layered designs allow for more complex flow paths, improving the interaction between fluids. The implementation of sharp bends or obstacles, for example, has been shown to induce chaotic advection, resulting in rapid and thorough mixing of reactive substances.
Additionally, the shift towards 3D printing technology for fabricating microfluidic devices has opened new avenues for precision and customization in design. This technology allows for the creation of intricate structures that were previously difficult to achieve through traditional methods. Notably, designs now often incorporate features like valves and pumps that are also 3D printed, allowing greater control over fluid movement and enhancing overall system performance.
Moreover, recent innovations include the use of soft lithography techniques that facilitate robust device fabrication while maintaining the integrity of microscale features. The adjustment of material properties through these methods has enabled better control over wetting and adhesion, crucial for effective mixing. As a result, these designs not only become more effective, but also reduce manufacturing costs and time.
Integration with Other Technologies
The integration of microfluidic mixing devices with other technologies has emerged as a key trend in recent years. This synergy enhances their applicability across various fields. For example, coupling microfluidic systems with optical sensors can provide real-time monitoring of chemical reactions. The seamless data collection allows researchers to make more informed decisions and adjust experimental conditions dynamically.
Furthermore, incorporating machine learning algorithms offers a way to optimize mixing parameters. By analyzing mixing efficiency and outcomes, these algorithms can recommend adjustments in real time, leading to improved performance of microfluidic devices.
Moreover, the integration of microfluidics with lab-on-a-chip technology has revolutionized various applications, including biomedical diagnostics and drug discovery. Such systems can conduct multiple processes simultaneously, significantly reducing the time needed for analysis while enhancing automation.
In summary, the recent advancements in microfluidic mixing technology emphasize the ongoing evolution of device design and its successful integration with other scientific technologies. This not only addresses previous challenges but also enhances the functionality and versatility of microfluidic systems, paving the way for innovative applications in scientific research.
Challenges in Microfluidic Mixing
Microfluidic mixing devices have transformed many fields through precise manipulation of fluids at the microscale, yet they confront several challenges that hinder their widespread application. Addressing these challenges is vital for optimizing device performance and enhancing functionality across diverse domains like biomedical engineering and chemical synthesis. Understanding these obstacles can provide critical insights into the ongoing development of more efficient microfluidic systems.
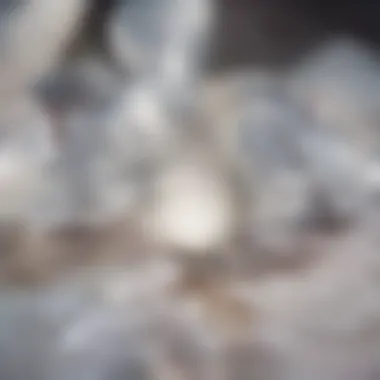
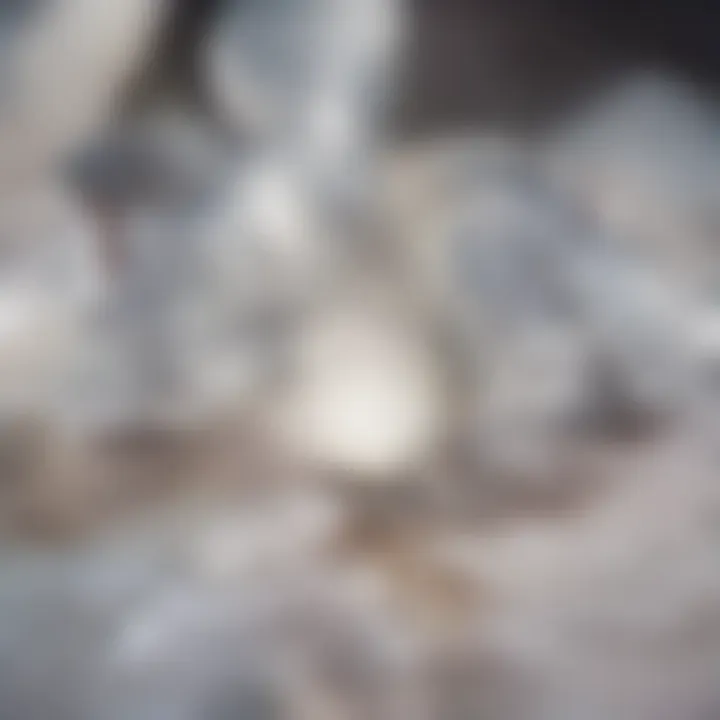
Scalability Issues
One of the major challenges with microfluidic mixing is scalability. As most microfluidic devices are designed for lab-scale experiments, transitioning them for industrial-scale applications remains a complex undertaking. Generally, the physical dimensions of microchannels are small, limiting their throughput. This limitation necessitates the redesign or replication of channels to increase flow rates while ensuring effective mixing.
Key factors affecting scalability include:
- Channel dimensions: Larger volumes can result in inefficient mixing, necessitating alterations to channel geometry.
- Flow conditions: Changes in flow rates must not compromise the mixing efficiency. Too low flow tends to lead to incomplete mixing, while too high may result in chaotic flow patterns.
- Device manufacturing: Current manufacturing techniques may not easily adapt to produce larger or multiple devices simultaneously.
These aspects complicate the process of scaling up designs efficiently without losing the benefits experienced at smaller scales.
Reproducibility of Results
Reproducibility is another significant challenge in microfluidic mixing. Achieving consistent results across different batches of microfluidic devices is crucial for both academic research and industrial applications. Variations in device fabrication and environmental conditions can lead to discrepancies in performance.
Factors contributing to reproducibility issues include:
- Fabrication variability: Minor differences in manufacturing processes can alter channel dimensions and surface properties, leading to varied flow dynamics.
- Environmental conditions: Factors such as temperature, humidity, and pressure may influence fluid properties and mixing behavior, complicating the prediction of outcomes.
- Material quality: The choice of materials affects not only mixing but also the device's longevity and reliability.
"To ensure the effectiveness of microfluidic devices, consistent reproducibility must be pursued particularly in critical applications such as drug development and diagnostic tools."
These challenges are critical as they directly impact the reliability and credibility of findings drawn from microfluidic mixing experiments. Therefore, addressing these problems must be a priority in ongoing research and development efforts within this field.
Future Directions for Microfluidic Mixing Devices
As microfluidic mixing devices evolve, understanding their future directions is crucial for both academic and industrial sectors. With ongoing research and advancements, the potential to revolutionize various fields becomes apparent. This section explores emerging research areas and potential market growth, highlighting the benefits and considerations for future developments in microfluidic mixing technologies.
Emerging Areas of Research
Innovative research avenues are continually arising in the realm of microfluidic mixing. These areas aim to enhance the efficiency and efficacy of mixing processes. Some notable fields of study include:
- Multiscale Mixing: This research explores mixing across different scales—ranging from molecular to macroscopic levels. The goal is to optimize mixing efficiency in complex applications.
- Integration with Biotechnology: Advancements in biotechnology often drive research in microfluidic devices. The integration of biological systems with microfluidics can lead to breakthroughs in drug delivery systems and personalized medicine.
- Smart Mixing Systems: The development of intelligent mixing systems involves incorporating sensors and automated controls. This can significantly improve the adaptability and reliability of microfluidic devices, leading to more precise mixing.
- Sustainable Practices: There is increasing interest in designing microfluidic devices that use less energy and resources. Researchers are exploring eco-friendly materials and minimizing waste while maximizing performance.
These emerging areas not only show promise for enhancing current technologies but also pave the way for groundbreaking applications previously thought impossible.
Potential Market Growth
The market for microfluidic mixing devices is poised for significant growth, driven by increased applications in various industries. Key factors influencing this growth include:
- Rise in Demand: The growing need for miniaturized and efficient mixing solutions in sectors like healthcare, pharmaceuticals, and environmental monitoring is driving the demand for microfluidic devices.
- Technological Advancements: Innovations continue to emerge, with new materials and manufacturing techniques redefining what is possible in microfluidics. These advancements increase reliability and expand the range of applications.
- Investment and Funding: Venture capital is increasingly flowing into startups focusing on microfluidic technologies. This influx of funding supports research and promotes commercial endeavors, further driving market expansion.
- Global Health Initiatives: There is a heightened awareness of health challenges, especially in the post-pandemic world. Microfluidic devices’ potential in rapid testing and diagnostics will be pivotal, enhancing their market relevance.
Closure
In the field of microfluidics, understanding the design, functions, and implications of microfluidic mixing devices holds significant importance. This conclusion synthesizes the core elements discussed throughout the article. Microfluidic mixing devices facilitate rapid and efficient mixing of fluids in a constrained environment, supporting innovations across multiple scientific realms.
Summary of Key Points
- Definition: Microfluidic mixing is essential for various applications, including biomedical research and chemical synthesis. The devices utilize small volumes of liquid to enhance efficiency and precision.
- Mechanisms: Both passive and active mixing mechanisms are employed, each having distinct advantages in different scenarios. Passive mixing relies on the intrinsic properties of fluid dynamics, while active mixing utilizes external forces to enhance mixing efficiency.
- Design Considerations: Factors such as channel geometry, material selection, and control of flow rates are crucial in the effective design of mixing devices. These parameters directly affect mixing efficiency, reproducibility, and device durability.
- Applications: The use of these devices spans from biomedical applications to environmental monitoring, where precise measurements and reactions are critical.
- Challenges: Issues related to scalability and reproducibility are significant impediments. Future research is necessary to address these challenges.
The Importance of Continued Research
The domain of microfluidics is continually evolving. Further investigation into the complexities of mixing technologies is essential for several reasons. Ongoing research may lead to the development of more efficient devices, ultimately enhancing precision in laboratory settings and industrial applications. Additionally, as new materials and methods come to the forefront, innovation in microfluidic mixing can drive breakthroughs in diagnostics, drug delivery, and even environmental sustainability.
Continued efforts in microfluidic research not only promise enhanced technologies but also the potential to solve pressing global challenges, particularly in health and environmental sectors.