Key Elements of a Wastewater Treatment Plant
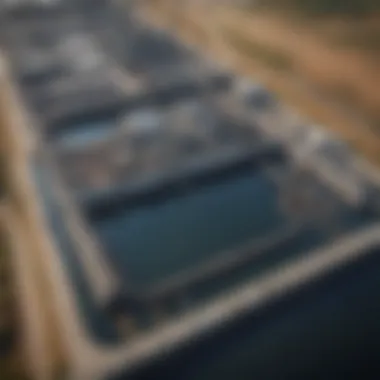
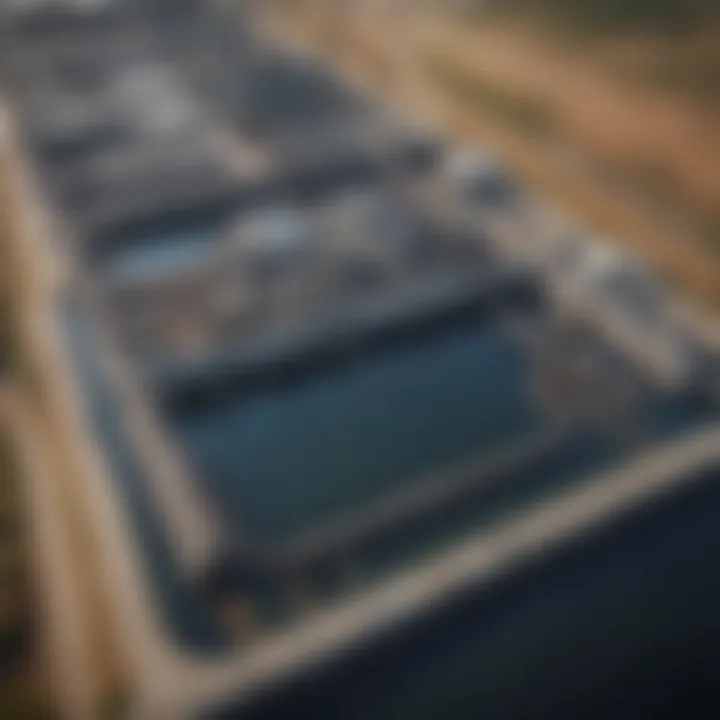
Intro
From the initial stages of treatment to the final, refined output, each section of the plant has a specific function that contributes to the overall effectiveness of the treatment process. In this article, we will embark on a detailed exploration of these components, including their significance, how they interconnect, and the methodologies employed in their operations.
Key Findings
Major Results
The investigation reveals common key sections integral to the functionality of wastewater treatment plants:
- Preliminary Treatment: Involves the removal of large debris and grit to protect the subsequent systems.
- Primary Treatment: Focuses on gravity separation to eliminate settleable materials from the wastewater.
- Secondary Treatment: Utilizes biological processes to break down organic matter.
- Tertiary Treatment: Advanced processes to filter and purify the remaining water, preparing it for discharge or reuse.
- Disinfection: Eliminates pathogens ensuring the water meets health standards before being released into the environment.
These components are symbiotic, contributing distinctively to the overall goal of protecting water quality and public health.
Discussion of Findings
In the world of wastewater treatment, the importance of each stage cannot be overstated. For instance, preliminary treatment acts as the first line of defense, filtering out items that could harm equipment downstream. The efficiency of subsequent stages hinges on the effectiveness of this initial phase. Moreover, technological advancements in secondary treatment processes, particularly in aeration techniques, have proven essential in optimizing the breakdown of organic pollutants.
"A robust understanding of water treatment processes provides communities the tools they need to monitor their own environmental impact."
Methodology
Research Design
The information presented arises from a systematic examination of wastewater treatment plants across various geographic locations. Observational studies, alongside existing literature reviews, provided the groundwork for understanding the componentsā functionality.
Data Collection Methods
Data were gathered through:
- Field Studies: Observations made during visits to wastewater treatment plants.
- Interviews: Discussions with plant operators and engineers to glean insights into practical challenges and solutions.
- Literature Review: Analysis of peer-reviewed articles and reports detailing advancements in treatment technologies.
This meticulous approach has paved the path toward greater insight into wastewater management, leading to a deeper appreciation of the technologies and practices that contribute to efficient and safe water treatment.
Prelims to Wastewater Treatment
Wastewater treatment is a cornerstone of modern sanitation and environmental health practices. As societies evolve, the quantity and complexity of wastewater generated have intensified. This complexity makes effective treatment processes not just beneficial but essential. \n\nWastewater is often laden with pollutants, toxins, and pathogens. The importance of its treatment transcends mere regulatory compliance; it is integral to protecting public health, maintaining ecological integrity, and supporting sustainable development. Neglecting this process can lead to dire consequences, including waterborne diseases and environmental degradation.\n\nWater is a finite resource, and the challenge lies in managing it wisely. Therefore, understanding the nuances of wastewater treatment is crucial for students, researchers, educators, and professionals alike.
Overview of Wastewater Characteristics
Wastewater can be broadly categorized into several types: domestic, industrial, and stormwater. Domestic wastewater, stemming from household activities, contains organic matter, pathogens, and nutrients, primarily nitrogen and phosphorus. Industrial wastewater, on the other hand, varies more significantly based on the nature of the industry āoften including heavy metals, hazardous chemicals, or manufacturing byproducts.
Stormwater is usually runoff from rain or snow that can carry debris, oil, and other pollutants into drainage systems. Each type of wastewater poses unique challenges and requires tailored treatment approaches.
Understanding these characteristics helps in designing effective treatment systems that can handle the varied and complex nature of incoming wastewater.
Importance of Treatment Processes
The treatment processes integral to wastewater management serve multiple objectives, such as:
- Public Health: Effective treatment reduces pathogens and harmful substances, mitigating potential health risks.
- Environmental Protection: Properly treated wastewater reduces pollution loads on water bodies, preventing ecosystem damage and protecting aquatic life.
- Resource Recovery: Advanced treatment methods can facilitate the recovery of water for reuse and valuable byproducts such as biogas and fertilizers.
Without a comprehensive treatment strategy, untreated or poorly managed wastewater can lead to irreversible consequences. For instance, freshwater sources can become compromised, leading to habitat losses and threats to biodiversity. A proactive approach to treatment is therefore not merely desirable; itās a necessary undertaking for ensuring future generations have access to clean water and a healthy environment.
"Sustainability today is tied to the effectiveness of our wastewater management practices tomorrow."
As we dig deeper into the components that make up a wastewater treatment plant, we will see how each element contributes to achieving these vital goals.
Preliminary Treatment Phases
In the delicate ecosystem of wastewater management, the preliminary treatment phases hold a vital role. This initial phase may often be overlooked, yet it is crucial for reducing the load on subsequent treatment stages. During this phase, large solids and grit are removed from the wastewater. This not only prevents damage to downstream equipment but also enhances the efficiency of the overall treatment process. If you think of the wastewater treatment plant as a well-oiled machine, preliminary treatment is akin to the first gear that keeps everything running smoothly.
Screening Components
Types of Screens
Various types of screens are utilized in preliminary treatment to ensure that significant debris is filtered out. The most common types include coarse screens and fine screens, each serving a distinct purpose. Coarse screens are typically the first line of defense. These larger screens capture big objects like sticks, leaves, and even plastic bottles. Fine screens, on the other hand, catch smaller particles that could otherwise evade the coarse screens.
The key characteristic of these screens is their mesh size; it affects what debris is kept out of the treatment overview. Coarse screens typically have larger openings, while fine screens possess smaller ones, catching finer materials. This two-tiered approach is a popular choice in effective treatment plants because it helps maintain the integrity of the overall system.
However, one mustn't overlook the unique feature of these screens: their ability to prevent human intervention in early stages of cleaning. Failing to manage debris could lead to costly repairs, thus confirming the screens' advantages in operational efficiency and cost-saving.
Function of Screening
The function of screening is not just about waste removal; it also significantly contributes to the functioning of the wastewater treatment plant. By removing large solids at the beginning, the screening process reduces the burden on subsequent treatment stages. This reduced load means that biochemical processes can proceed more smoothly, leading to more effective treatment down the line.
Moreover, advanced screening systems often come with automatic cleaning features. This leads to less operational involvement. However, one should consider that certain screenings do demand periodic maintenance to ensure optimal functioning. In the long run, the combination of performance and efficiency makes screening an essential part of the wastewater treatment narrative.
Impact of Screening on Treatment Efficiency
The impact of screening on treatment efficiency heightens with each passing stage of wastewater processing. Efficient screening results in fewer interruptions during treatment. By catching large solids upfront, it allows downstream processesālike sedimentation and biological treatmentāto function without interferences. Essentially, the screening phase acts like a gatekeeper, ensuring that only manageable wastewater enters subsequent systems.
A unique feature of the screening process lies in its ability to normalize flow rates. When received solids are minimized, the overall flow becomes steadier, allowing operators to predict and optimize treatment processes. This consistency plays a substantial role in improving the efficiency of the entire plant. In summary, effective screening sets the stage for a smoother, more efficient treatment journey.
Grit Removal Systems
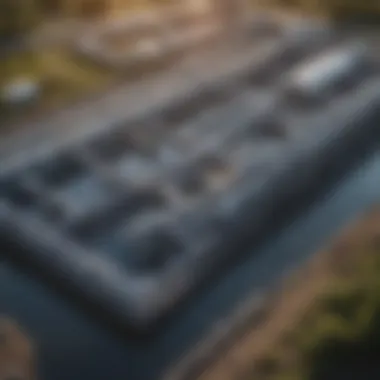
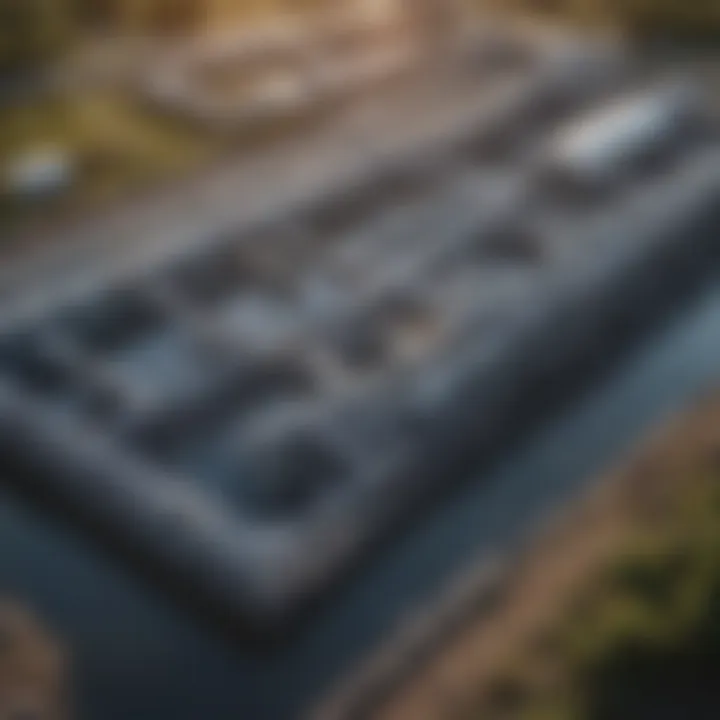
Types of Grit Chambers
Grit chambers are another vital aspect of preliminary treatment. Their main function is to remove gritty materials, like sand and small stones, which can cause significant wear on equipment if left unchecked. Various types of grit chambers can be found, including rectangular grit chambers and vortex grit chambers. These designs tend to vary based on flow conditions and space availability.
One of the key characteristics that makes these chambers indispensable is their design, which aims to facilitate sedimentation. Vortex grit chambers utilize the centrifugal force to separate grit from water quickly. This efficiency makes them a preferred option in many modern treatment facilities. However, operators must recognize that each type of chamber has unique maintenance requirements, making it crucial to choose wisely based on the specific wastewater characteristics.
Efficiency and Maintenance
When discussing efficiency and maintenance, grit removal systems play an essential role in the operational backbone of a treatment plant. Properly functioning grit chambers can make a world of difference in the upkeep of downstream equipment. They require routine maintenance efforts to ensure all grit is effectively removed, if neglected could result in equipment damage and costly repairs.
One vital aspect of efficiency in these systems is their ability to operate with minimal chemical additives. As grit removal largely relies on physical separation principles, this gives them an edge over more chemically intensive processes, helping maintain environmental sustainability.
Role in Preventing Wear and Tear
The role of grit removal systems focuses heavily on preventing wear and tear on mechanical components downstream. By removing harmful grit, the likelihood of damage to pumps and aerators can greatly reduce. This not only prolongs equipment lifespan but also lowers maintenance costs in the long run.
A noteworthy characteristic of grit systems is their capacity to facilitate more effective treatment operations. When equipment runs optimally, it allows for better resource management and savings in energy consumption. However, if improperly managed, grit could create operational inefficiencies that can escalate costs. It becomes clear that investment in effective grit removal systems pays dividends, both in ensuring machinery longevity and optimizing overall process efficiency.
"A stitch in time saves nine," reflects the essential nature of these preliminary phases in wastewater treatment. Without robust preliminary treatment, the entire system could face disruptive consequences.
Primary Treatment Elements
The primary treatment elements play an essential role in the wastewater treatment process, acting as the first line of defense against contaminants. This stage is crucial because it physically separates solids and greases from liquids, which significantly reduces the volume and load of pollutants entering the next treatment phases. By ensuring that the wastewater is a bit cleaner before it moves on, the primary treatment sets the tone for the entire treatment process. In this section, we will dive into the primary building blocks of this stage: sedimentation tanks and primary clarifiers.
Sedimentation Tanks
Mechanics of Sedimentation
Sedimentation tanks work on a fundamental principle: gravity. As wastewater settles in these tanks, heavier solids sink to the bottom, which creates sludge. An important aspect is the concentration gradientāparticles fall at different rates based on their size and density. Larger, denser particles settle quickly, which means that the design of these tanks must accommodate the characteristics of the incoming wastewater. A standout feature of sedimentation tanks is their ability to efficiently reduce the organic load by allowing enough time for solids to settle without excessive turbulence.
Advantages: This process can be tremendously efficient in reducing suspended solids, making greater amounts of waste manageable later. It's often seen as a low-energy solution compared to other treatment methods, lowering operational costs.
Disadvantages: However, it does require regular maintenance to manage sludge buildup and can be less effective if the inflow varies significantly in composition.
Design Variations
When it comes to design variations in sedimentation tanks, there are several options to consider. Circular and rectangular tanks are common, each having its pros and cons. For instance, circular tanks often allow for more efficient settling due to their shape, while rectangular tanks may fit better into urban spaces.
The critical attribute of these design variations lies in their effectiveness in optimizing space and operational performance. A unique feature of some modern tanks is the inclusion of mechanisms for enhanced mixing prior to sedimentation, noticeably improving the quality of the effluent.
Advantages: These variations can help to cater to specific needs based on the wastewater characteristics, thus enhancing the overall efficiency of the treatment process.
Disadvantages: On the flip side, the more complex designs may require advanced monitoring systems, increasing long-term costs.
Expected Outcomes
The expected outcomes from sedimentation tanks are multi-faceted, with a primary goal of reducing solids concentration. The percentage of solids removed can reach impressive levelsāoften over fifty percent, depending on the design and operation.
A crucial characteristic of this expectation is the consistency of effluent quality. The tanks effectively prepare the wastewater for the secondary treatment phase, which is vital in maintaining the overall treatment plant efficacy.
Advantages: This sets the foundation for improved performance in subsequent treatment steps, as well as ensuring compliance with environmental regulations.
Disadvantages: Nonetheless, inconsistency in sludge removal or frequent operational disturbances can affect the expected outcomes negatively.
Primary Clarifiers
Functionality of Clarifiers
Primary clarifiers operate similarly to sedimentation tanks but with a more focused role. Their core function is to further clarify the water post-sedimentation, allowing finer solids to settle out. An attractive feature of clarifiers is their ability to manage varying flow rates while maintaining treatment efficiency.
Advantages: They effectively achieve greater removal rates of suspended solids, greatly enhancing the quality of effluent sent to secondary treatment.
Disadvantages: Yet, the potential for operational challenges exists, especially if not properly maintained, leading to concerns over the clarity of the discharged water.
Operational Considerations
Consideration in operational factors for primary clarifiers is key. They require a careful balance and monitoring of flow rates to prevent short-circuiting, which could compromise treatment performance. The unique characteristic of clarifiers is that they can be adjusted to accommodate fluctuations in wastewater composition by modulating the detention times.
Advantages: This flexibility allows for more robust handling of storm events and variations in incoming load.
Disadvantages: However, frequent adjustments necessitate vigilant operations, which can strain resources.
Effects on Subsequent Treatment Stages
The effects of primary clarifiers on subsequent treatment stages cannot be overstated. By removing a significant amount of solids and organics, they lay a critical groundwork for the efficiency of secondary treatment processes. A key characteristic is their contribution to reducing operational load in secondary systems, leading to lower energy requirements.
Advantages: This results in a smoother and more responsive operation across the treatment plant.
Disadvantages: Yet, if the clarifiers underperform, the subsequent processes may face challengesācausing delays in treatment and a risk of non-compliance with discharge regulations.
Effective management of primary treatment elements is essential in maintaining not only the functionality of the treatment plant but also the environmental standards expected by modern society.
Secondary Treatment Components
Secondary treatment is crucial in the wastewater treatment process, primarily because it addresses organic matter and suspended solids that remain after primary treatment. The key aim here is to significantly reduce the concentration of biodegradable materials, making the effluent less harmful upon reintroduction into the environment. This stage not only serves health purposes but also plays a role in reclaiming resources that can be beneficial for various applications, such as water reuse and irrigation.
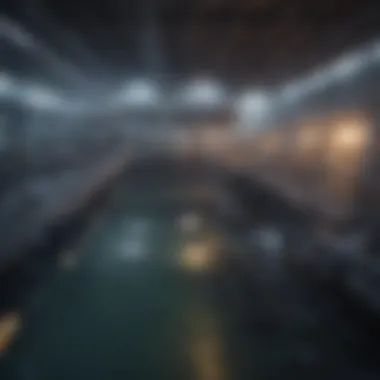
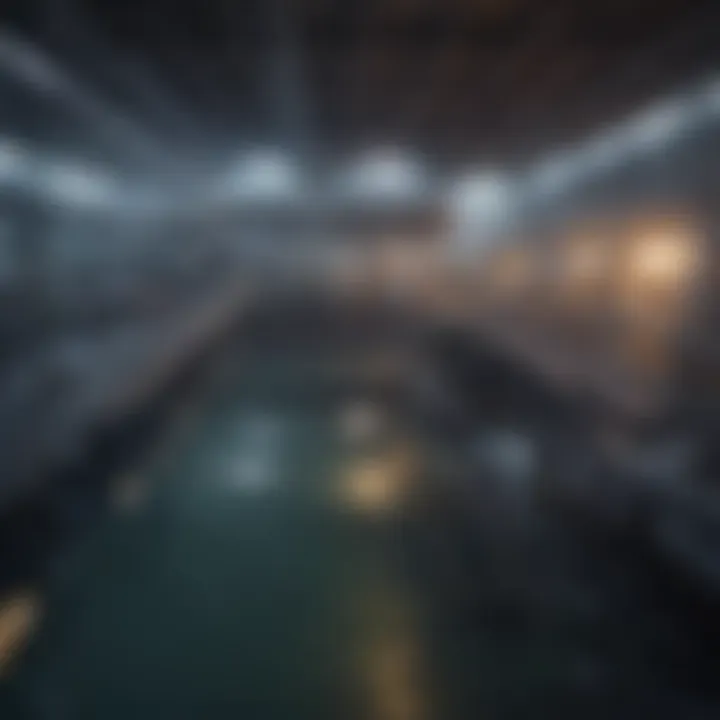
Aeration Tanks
Aeration tanks come into play as a significant component in secondary treatment, allowing the growth of microorganisms, which is essential for breaking down organic pollutants. This process hinges on efficiently aerating the wastewater to foster a thriving biological community.
Types of Aeration Systems
When looking at types of aeration systems, two main categories dominate: diffused aeration and mechanical aeration.
Diffused aeration introduces air into the wastewater through a network of pipes and fine bubbles, creating an effective oxygen transfer mechanism. This method tends to favor biological activity, promoting ideal conditions for microorganisms. On the other hand, mechanical aeration uses surface mixers or turbines to agitate the water physically and promote contact between wastewater and air.
Each type comes with its set of advantagesādiffused aeration is generally quieter and more energy-efficient. Mechanical systems, however, do require more maintenance due to moving parts.
Biological Treatment Process
The biological treatment process within aeration tanks leans heavily on the use of microorganisms to decompose organic matter. This is where the term "activated sludge" often pops up. It refers to the mixed liquor in aeration tanksāthe suspension of microorganisms working diligently to eat away at contaminants. The standout characteristic of this method is its efficiency in converting organic material into microbial biomass and by-products, which can be further treated or used. Nonetheless, a downside is that the process requires careful monitoring and control of conditions like temperature and pH to maintain optimal microbial activity.
Factors Affecting Aeration Efficiency
Several factors can heavily influence aeration efficiency. Among them, the dissolved oxygen (DO) concentration is paramount. Higher DO levels generally enhance the activity rates of microorganisms, furthering degradation of waste. Additionally, temperature and mixing intensities come into play. Careful balance is required; too much aeration can lead to excessive costs and system wear, while too little can stifle biological processes. This tightrope act impacts operational costs and treatment effectiveness, making it vital to find the sweet spot for each facility.
Secondary Clarifiers
Following aeration, secondary clarifiers serve as a pivotal component, allowing for the separation of solids and treated water. This step is essential in ensuring that the effluent meets quality standards before it moves on to tertiary treatment.
Function and Efficiency
The function of secondary clarifiers is straightforward yet vital: they facilitate the settling of floc, which contains microorganisms and other particulates that have been blended with the wastewater. The efficiency of these clarifiers, often influenced by design and operation, directly affects the amount of solids returned to the aeration system for further processing. A well-functioning clarifier leads to reduced suspended solids in the effluent, promoting overall treatment effectiveness.
Aspect of Flocculation
Flocculation refers to the process of forming clumps, or flocs, of suspended solids during clarification. The process relies heavily on gentle mixing, allowing tiny particles to collide and bind together, leading to more efficient settling. The key point here is the significant enhancement in solid removal compared to simple sedimentation. This means a greater degree of purity in the effluent, significantly benefiting subsequent treatment stages.
Impact on Overall Treatment Quality
The impact of secondary clarification on overall treatment quality cannot be overstated. This stage is designed to ensure that the effluent not only meets regulatory standards but is also safe for environmental discharge. The unique feature of secondary clarifiers is their ability to dramatically lower the concentration of suspended solids and biological material, shaping the quality of treated water. Consequently, optimally functioning secondary clarifiers lead to reduced pollution load reaching receiving waters, hence playing a vital role in protecting ecosystems.
Effective secondary treatment processes are key to ensuring that treated water can responsibly re-enter the environment without causing ecological harm.
Tertiary Treatment Mechanisms
Tertiary treatment serves as the final step in the wastewater treatment process. This phase plays a vital role in ensuring the effluent meets the required standards before it is released back into the environment or repurposed for various uses. Essentially, tertiary treatment enhances the quality of treated wastewater to a level suitable for specific applications, such as irrigation or industrial use.
Filtration Techniques
Types of Filters Used
Tertiary treatment often employs a range of filtration techniques to effectively remove remaining impurities from treated water. The main types of filters include sand filters, membrane filters, and cartridge filters. Sand filters are particularly popular due to their ability to trap fine particles through layers of sand, making them efficient for large volumes of water. Membrane filters, on the other hand, utilize semi-permeable membranes that can separate particles and bacteria with high precision. The unique feature of membrane filters is their ability to achieve very high levels of effluent quality, minus most pathogens. However, they can be expensive to operate and maintain compared to sand filters.
Performance Metrics
Evaluating the effectiveness of filtration techniques hinges on various performance metrics such as removal efficiency, flow rate, and operational costs. A key characteristic here is the percentage of total suspended solids eliminated by the filtration system. High removal rates indicate effective filtration, which is why many plants emphasize optimizing these metrics. Unique features include monitoring parameters like turbidity and bacteria counts, making it easier to assess filtration performance. However, constant monitoring and potential downtime can be challenges faced in achieving consistent performance.
Role in Water Reuse
The role of filtration in water reuse cannot be overstated. Properly filtered water is not just safe for returning to natural bodies; it can also be repurposed for irrigation, industrial applications, or even drinking water in advanced treatment scenarios. A significant characteristic is its contribution to sustainable water management practices by minimizing the demand for fresh water sources. The unique aspect is the adaptability of filtration technologies to meet varying reuse requirements. On the downside, the initial infrastructure can be costly, and public acceptance can sometimes pose a challenge due to the perception of using treated wastewater.
Disinfection Processes
Common Disinfection Methods
Disinfection serves as a crucial component in tertiary treatment to ensure that pathogens are eliminated from wastewater. Common methods include chlorine disinfection, ultraviolet (UV) radiation, and ozone disinfection. Chlorination involves adding chlorine compounds to kill bacteria and other harmful microorganisms; its affordability makes it a popular choice in many installations. However, the byproducts of chlorination can be of concern, as they may form potentially harmful compounds. UV disinfection involves exposing water to UV light, destroying bacteria without the use of chemicals. Its unique benefit lies in the absence of chemical residual, making it an environmentally friendly choice. However, it requires proper maintenance to ensure effectiveness.
Assessment of Effectiveness
Assessing the effectiveness of disinfection methods is crucial for ensuring water safety. Metrics typically reviewed include total coliform counts, E. coli levels, and pathogenic microorganism reduction. A key characteristic here is the ability to meet stringent health regulations. Many facilities conduct routine testing to ensure compliance with local and federal standards, turning assessments into a key operational priority. This careful monitoring can sometimes lead to increased costs if a method proves ineffective, calling for mitigation changes or additional treatment stages.
Regulatory Standards
Regulatory standards frame the expectations for how treated water should perform, particularly in relation to public health. A crucial characteristic is that these standards often vary widely by region, which means facilities must stay informed about local guidelines. Specific requirements may dictate the extent of pathogen reduction required for effluents intended for various uses. Often, regulatory bodies perform regular evaluations to ensure compliance, and failing to meet standards can result in significant penalties. The transparency of these regulations is usually beneficial, as it broadens public trust in water reuse initiatives; however, they require constant adaptation and vigilance from treatment plant operators.
Sludge Management Systems
In the realm of wastewater treatment, sludge management systems stand tall as an essential component, holding a significant place in ensuring environmental sustainability. Sludge, which is a byproduct of the treatment processes, contains a mixture of organic and inorganic matter, making its management crucial. Proper handling not only mitigates environmental impact but also boosts overall efficiency in wastewater plants. More than just a necessary evil, the practice of managing sludge has become integral in transforming waste into resources.
Sludge Thickening
Methods for Thickening
Thickening sludge is the first step in its management, and several methods exist to achieve this. The most common techniques include gravity thickening, mechanical thickening, and dissolved air flotation. Gravity thickening relies on the natural process where solids settle at the bottom of a tank, increasing their concentration. This method is straightforward and often favored for its simplicity and low operational costs. In contrast, mechanical thickening uses equipment, such as centrifuges, which speed up the thickening process but can be more complex and costly to maintain. Regardless of the method, the goal remains the same: concentrate waste solids to reduce the volume sent for further treatment or disposal, ultimately facilitating more efficient processing.
One unique feature of mechanical thickening is its ability to recover more water from sludge, enhancing the overall dryness of the end product, although it tends to require more energy.
Benefits to Treatment Efficiency
The main benefit of effective sludge thickening is its contribution to treatment efficiency. By reducing the volume of sludge, it allows for a more manageable operation in subsequent treatment stages. Higher concentration translates to fewer resources needed for further handling and processing, thus lowering operational costs over time. Additionally, thickened sludge generates less residual waste, which is beneficial for land application and other reuse strategies.
Moreover, concentrated sludge can lead to enhanced biogas production if proceeded to anaerobic digestion. This makes treatment not just an environmental necessity, but also a financial opportunity for the plants.
Challenges in Implementation
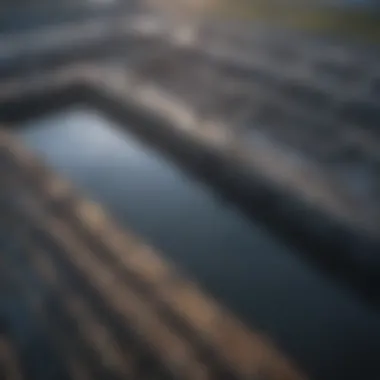
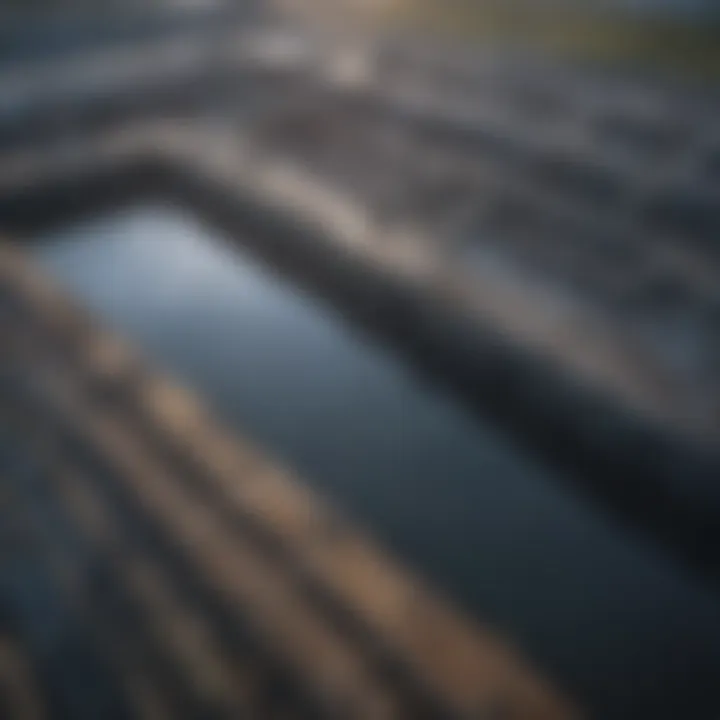
Despite the clear advantages, implementing sludge thickening presents challenges. One of the primary hurdles is the requirement for precise control of operational parameters. Too much or too little thickening can hinder treatment processes or lead to excessive solids accumulation. Moreover, older plants may lack the necessary equipment to implement modern thickening techniques, thus requiring significant investments for upgrades.
In addition, maintaining optimal conditions for thickening in the presence of varying wastewater compositions can complicate operations, posing risks to overall treatment quality. Addressing these challenges requires continuous monitoring and sometimes a rethink of the existing sludge management protocols.
Digestion and Stabilization
Anaerobic vs. Aerobic Digestion
Another cornerstone in sludge management is the digestion process, which can be categorized into anaerobic and aerobic methods. Anaerobic digestion occurs without oxygen and is well-known for its ability to produce biogas, a renewable energy source. It's appealing due to its relatively low energy requirements and high efficiency in breaking down organic matter. However, it necessitates careful management of temperature and retention time to maintain optimal microbial activity.
On the other hand, aerobic digestion uses oxygen to decompose sludge, often yielding a more stable final product. It tends to work faster compared to its anaerobic counterpart but demands additional energy for aeration. Each method presents unique advantages and disadvantages, and the choice depends on the specific goals of the wastewater treatment facility.
Process Operations
Process operations in digestion are crucial as they dictate how effectively the systems work. Key considerations involve monitoring parameters like temperature, pH, and retention time. Efficient operation minimizes odors and maximizes biogas yield, while also reducing the volume of solids. Automation and real-time monitoring can significantly improve the reliability of these processes, allowing for rapid adjustments if needed.
Emphasizing automation and effective control strategies ensures that process operations remain efficient, aligning with modern sustainability goals, but introduces potential costs which some smaller facilities may find burdensome.
End Products and Their Uses
The end products of digestion, particularly digestate, serve several uses. This nutrient-rich material can be repurposed as fertilizer in agricultural practices, benefiting the soil and contributing to a circular economy. Additionally, biogas generated can provide energy for facility operations or be further refined into usable fuel.
In terms of marketability, the versatility of these end products provides an avenue for wastewater treatment plants to generate additional revenue. Nevertheless, regulatory challenges and public perception regarding the use of treated biosolids can hinder product acceptance, requiring ongoing education and transparency with communities.
In summary, the effective management of sludge through thickening and digestion processes plays a vital role in enhancing the overall efficacy of wastewater treatment. Balancing operational challenges with the benefits that arise from resource recovery remains a critical consideration for environmental sustainability.
Final Disposal and Reuse
Final disposal and reuse are crucial stages in the wastewater treatment process. They represent the culmination of the extensive treatment efforts, ensuring that the effluent and residuals are managed sustainably and any potential environmental impact is minimized. Properly executed, these processes not only aid in returning necessary nutrients and resources back into the ecosystem but also present significant opportunities for resource recovery, thereby supporting overall sustainability goals.
Land Application of Treated Sludge
Land application of treated sludge is an effective means of recycling nutrients back into the soil. It involves using stabilized biosolids as a soil amendment. This practice holds various benefits but comes with its own set of risks that need careful management.
Benefits and Risks
The primary benefit of land application lies in its recycling capability. Instead of ending in a landfill, the treated sludge replenishes the soil organic matter, promoting healthier agricultural productivity. However, there is a risk of heavy metal accumulation and pathogens potentially leaching into groundwater. This necessitates comprehensive sludge treatment processes to mitigate such risks.
Even with its advantages, public perception often sways the viability of this practice, especially when it comes to crops meant for human consumption. Thus, balancing benefits against the risks is essential in this context to ensure public trust and safety.
Regulatory Guidelines
Regulatory guidelines dictate how and when and where land application can occur. These guidelines typically aim to maintain transparency and ensure environmental safety. For example, jurisdictions often limit the amount of heavy metals in treated sludge. They might also stipulate buffer zones around water sources to prevent contamination.
Following these regulations not only promotes ecosystem health but also upholds public confidence in the use of biosolids. Non-compliance can lead to significant legal repercussions, and more importantly, can compromise water quality significantly.
Best Practices for Application
To maximize the effectiveness of land application, several best practices should be followed. First, it is important to conduct soil tests to establish nutrient requirements and ensure the right application rates. Moreover, timing of the application plays a vital role; for instance, applying treated sludge during off-seasons can minimize crop contamination and promote soil absorption.
Maintaining detailed application records is another essential practice, ensuring accountability and traceability of usage. While the benefits are numerous, identifying the right methods and procedures can definitely enhance both public perception and agricultural yield.
Reclamation of Treated Water
Reclaiming treated water for various uses is proving to be an increasingly wise choice in many regions. It not only conserves freshwater resources but also showcases innovative wastewater management practices.
Technologies Used for Reclamation
Various technologies are employed in water reclamation, such as membrane filtration and reverse osmosis. These methods allow for highly treated effluent that is suitable for non-potable uses, including irrigation and even industrial applications. The sophistication of these technologies keeps increasing, leading to better quality effluents that meet strict regulations.
However, these innovations may come with higher operational costs and energy demands, challenging their scalability in every context. Adopting these technologies should consider both economic viability and environmental impact.
Applications of Reclaimed Water
Reclaimed water opens doors to a host of applications. From irrigation in agriculture to landscape maintenance, thereās no denying its usefulness. Industrial sectors, like cooling for power plants, can also benefit significantly from using reclaimed water instead of relying on scarce freshwater supplies.
Highlighting such applications reflects the ecological footprint reduction that can be achieved through responsible management, making it a beneficial practice overall.
Challenges in Public Acceptance
Despite the benefits, challenges remain, especially regarding public acceptance of reclaimed water. Health concerns and distrust often play significant roles in how communities view reclaimed water initiatives. Public awareness campaigns and transparency about processes can help alleviate fears.
One of the notable aspects is that addressing these challenges effectively requires community engagement and education. When people understand how the treatment works, they are more likely to embrace reclaimed water use, recognizing its contribution to water conservation.
It is essential to highlight that, while risks exist, so do the benefits if managed and communicated properly.
"The best way to predict the future is to create it." - Peter F. Drucker
Through a strategic approach to final disposal and reuse, communities can pave the way for sustainable water resource management.
Culmination
Summary of Key Components
Through the examination of each layer, from preliminary treatment to final disposal, we gather a sense of how these systems intertwine:
- Preliminary Treatment: Here lies the first line of defense against large debris and grit that can clog systems or harm equipment. Screening and grit removal are foundational tasks that set the stage for efficient further treatment.
- Primary Treatment: Sedimentation tanks and primary clarifiers act as the initial digesting phases, allowing heavier solids to settle out and lighter fractions to pass through for secondary treatment.
- Secondary Treatment: This phase encompasses aeration tanks and secondary clarifiers that utilize biological processes to break down organic matter. It's where much of the transformation occurs, turning polluted wastewater into less harmful effluents.
- Tertiary Treatment: Through filtration and disinfection, final purification prepares the water for potential reuse or safe discharge into the environment. Effective practices in this stage are essential for communities reliant on reclaimed water.
- Sludge Management: Overseeing the byproducts of treatment through thickening and digestion ensures that the processes are sustainable and contribute to recycling efforts.
- Final Disposal and Reuse: The land application of treated sludge and water reclamation not only mitigates waste but also fosters agricultural and ecological benefits.
Future Directions in Wastewater Treatment
Advancements in technology and environmental awareness are driving the evolution of wastewater treatment. Key areas for development include:
- Innovative Treatment Technologies: Emerging techniques such as membrane bioreactors and advanced oxidation processes offer higher efficiency and reduced footprints for treatment plants.
- Automation and Smart Systems: As more facilities adopt AI and IoT technologies, monitoring and managing wastewater processes can transform, making them more responsive and efficient.
- Sustainability Practices: The move towards a circular economy emphasizes the role of wastewater treatment in resource recovery, focusing on extracting energy, nutrients, and clean water from waste streams.
- Public Engagement Strategies: Building trust and understanding with communities regarding treated water use is vital. Efforts to educate and involve the public can lead to greater acceptance and participation.