Hydrogen Storage Techniques for Fuel Cells Explained
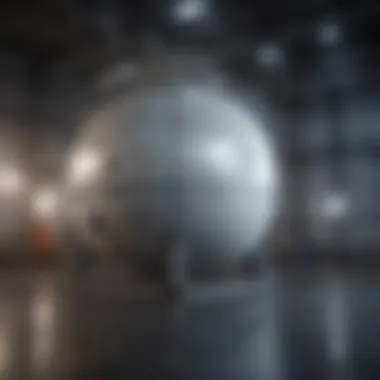
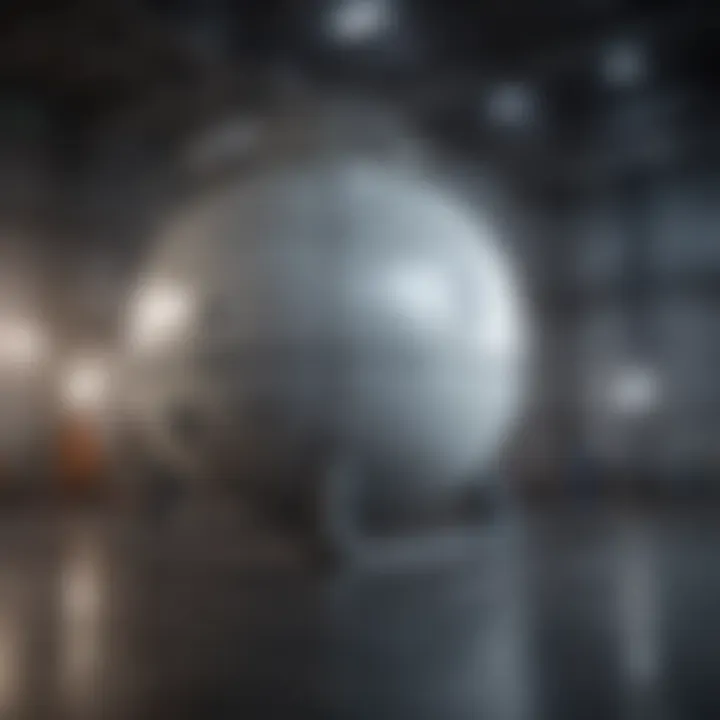
Intro
As the world steers towards sustainable energy, hydrogen fuel cells emerge as a pivotal technology. Storing hydrogen effectively is critical to unlocking the full potential of fuel cells. However, the journey of hydrogen storage is no walk in the park.
Hydrogen, the most abundant element in the universe, holds promise as a clean fuel source. Yet, its energy density and physical properties present distinctive challenges that researchers, engineers, and policymakers must navigate. From high-pressure tanks to chemical storage solutions, each method comes with its own set of hurdles and opportunities. Thus, understanding these methods forms the backbone of any comprehensive discussion on hydrogen storage for fuel cells.
In the following sections, we will unravel the key findings that underpin the methodologies employed in hydrogen storage, giving a clear picture of not just where we are, but where we might go next.
Prolusion to Hydrogen as an Energy Source
Hydrogen has long been touted as a promising candidate in the quest for clean and sustainable energy solutions. Its versatility in various applications, particularly in fuel cells, makes it a vital player in the shift from fossil fuels to more environmentally-friendly alternatives. Understanding hydrogen as an energy source requires a closer examination of its properties, benefits, and the challenges it faces.
One of the standout features of hydrogen is its abundance. It is the most common element in the universe, typically found bonded with other elements, such as in water (HâO). When harnessed for energy, hydrogen produces only water vapor as a byproduct, making it a leader in reducing greenhouse gas emissions. This characteristic positions hydrogen as not just another energy source, but a potential lifeline in the global fight against climate change.
The Role of Hydrogen in the Energy Transition
In the current landscape of energy production, hydrogen is gaining traction, transforming how we think about energy as a foundational element of the grid. By storing energy often generated from renewable sources, like solar and wind, hydrogen can act as a form of energy storage that compensates for their intermittency.
- Flexibility: Hydrogen systems can be integrated into various sectors, ranging from transportation to industrial processes.
- Energy Carrier: It serves as a carrier of energy, enabling the long-distance transportation of power, something electricity cannot achieve as effectively.
- Decarbonization: Industries that currently rely on carbon-intensive processes can adopt hydrogen as a tool for reducing their carbon footprint.
With potential applications in powering vehicles, heating homes, and even generating electricity, hydrogen is set to play a pivotal role in the energy mix of the future.
Overview of Fuel Cells
Fuel cells represent a remarkable technology that converts chemical energy directly into electrical energy through electrochemical reactions. They typically employ hydrogen as fuel, which reacts with oxygen from the air to generate electricity, heat, and water. This efficient process offers several advantages that might pique the interest of energy advocates.
The main benefits include:
- High Efficiency: Fuel cells can achieve efficiencies greater than traditional combustion-based systems.
- Scalability: They are suitable for a wide array of applications, from small portable devices to large-scale power plants.
- Quiet Operations: Unlike many generators, fuel cells operate quietly, reducing noise pollution significantly.
However, some challenges remain in deployment, including system costs and the need for infrastructure. As technologies advance and scales of production increase, obstacles can be mitigated, paving the way for hydrogen fuel cells to become a staple in our future energy ecosystem.
Understanding Hydrogen Storage
The conversation around hydrogen storage is more than just a technical matter; it holds the key to harnessing hydrogen's potential as a clean energy source. As we delve into this intricate subject, we must recognize the pivotal role storage plays in the broader context of fuel cell technology. Effective hydrogen storage not only determines the efficiency and safety of fuel cells but also influences how widely this technology can be deployed in various applications, from transportation to stationary power generation.
Why Hydrogen Storage Matters
Hydrogen does wonders for being a clean energy carrier, but its virtues are tarnished without proper storage solutions. The fact is, hydrogen is the lightest element, making it challenging to contain. Uncontrolled release can be downright dangerous. Thus, understanding how to store hydrogen safely and efficiently is of paramount importance.
Emphasizing the need for robust storage solutions, we find that:
- Safety first: Storing hydrogen comes with a full load of risksâexplosive reactions and leaks can happen if the material and methods used are subpar. High-quality storage methods help mitigate these dangers, making fuel cells a more appealing option.
- Enhanced efficiency: Efficient storage solutions allow for higher energy densities. The more hydrogen you can store in a given volume, the further fuel cells can take you, making them suitable for everyday vehicles and even large industrial applications.
- Scalability: Hydrogen storage determines how quickly and effectively the technology can be expanded to meet demands. Sustainable solutions enable industries to ramp up usage without significant barriers.
In short, storage technologies pave the way for hydrogen's successful integration into the energy landscape. Better storage means better energy management.
Key Characteristics of Hydrogen
When discussing hydrogen storage, it's essential to consider the characteristics of hydrogen itself. These properties inform how we can best store the gas:
- Diffusivity: Hydrogen molecules are exceptionally small and can diffuse through many materials. This property necessitates robust containment methods to prevent leaks.
- Energy content: While hydrogen has high energy density by weight, itâs low by volume. This means we need to be mindful of how we compress or liquefy it for storage, making efficiency a top priority.
- Reactivity: Hydrogen is highly reactive under certain conditions, especially in the presence of oxygen. This factor can complicate storage methods, especially in environments where heat and light fluctuate.
Given these traits, the choice and design of storage systems need to be tailored specifically to the nature of hydrogen, ensuring both effectiveness and safety. Understanding these fundamental characteristics lays the groundwork for exploring the various storage methods.
"Hydrogen can be a game-changer in our transition to cleaner energy, but effective storage is the linchpin of this transformation."
Recognizing how these factors shape our approach to hydrogen storage is vital, as they define the landscape weâre navigatingâone that blends opportunity with challenges and potential with risk.
Methods of Hydrogen Storage
Hydrogenâs potential as a clean energy source hinges significantly upon effective storage solutions. Storing hydrogen efficiently not only enhances the practicality of fuel cells but also enables the broader adoption of hydrogen as an energy carrier. Having a deeper understanding of methods for hydrogen storage is vital because each method comes with its own advantages and drawbacks. By exploring traditional and emerging technologies, stakeholders can better decide the suitable approach for various applications. The efficacy with which hydrogen is stored directly correlates to reductions in cost and improvements in the overall safety of hydrogen utilization, thus driving the shift towards sustainable energy solutions.
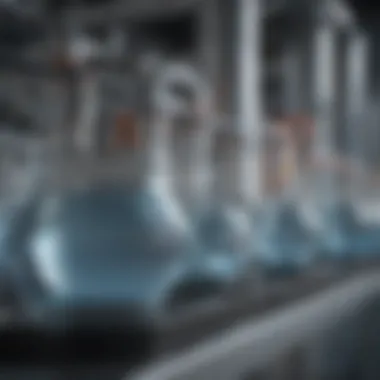
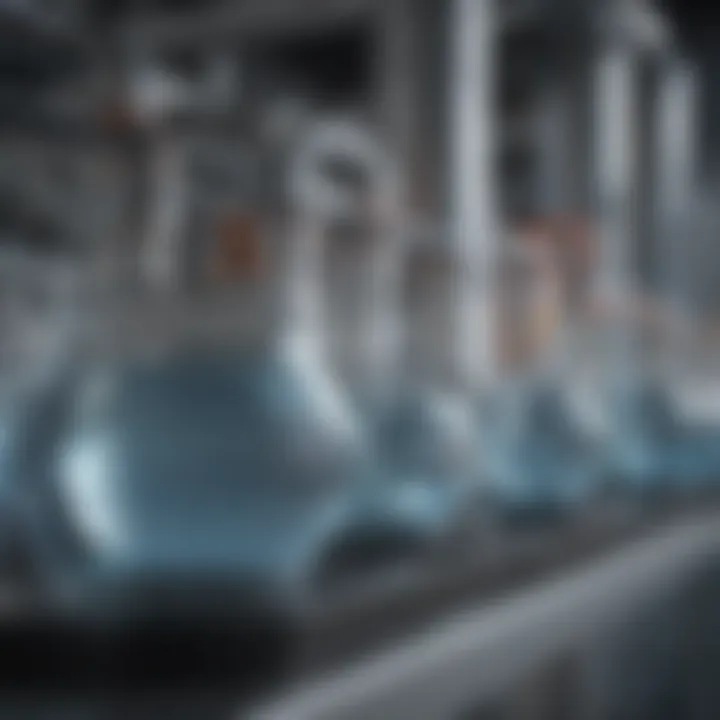
Compressed Hydrogen Storage
Compressed hydrogen storage has established itself as a common method for transporting and storing hydrogen due to its relative simplicity and effectiveness.
Advantages of Compressed Hydrogen
One of the evident advantages of compressed hydrogen is its ease of transportation. When hydrogen is stored under pressure, it occupies a smaller volume, making it more feasible to move large quantities of fuel to different locations. For instance, hydrogen gas can be compressed to pressures up to 700 bar, which makes it suitable for refueling stations or storage facilities.
Moreover, the infrastructure for compressed hydrogen is continually developing, leading to economies of scale. Investing in this method often leads to lower operating costs over time. Furthermore, the mature technology associated with compressed gas storage facilities means that they come with established safety protocols, minimizing risk during operations. The unique feature of compressed hydrogen storage lies in its ability to integrate with existing natural gas frameworks, thus streamlining implementation and supporting the transition towards clean fuels.
Disadvantages and Safety Concerns
Despite the advantages, there are notable disadvantages linked to compressed hydrogen storage. High-pressure systems can entail significant safety risks, such as the potential for explosive decompression if integrity is compromised. This makes regular inspections and maintenance paramount to ensure the safe operation of such systems.
Another consideration is the energy required to compress hydrogen itselfâthis can lead to efficiency losses. The high-energy costs can outweigh the benefits, particularly in applications where energy efficiency is critical. Additionally, hydrogen's low molecular weight means it can permeate some materials more readily, leading to potential leaks and safety hazards that must be effectively managed. Addressing these concerns is critical for advancing the acceptance and viability of compressed hydrogen storage in the broader energy landscape.
Liquid Hydrogen Storage
Liquid hydrogen is another method of storage that is often utilized, particularly in applications that require very large quantities of hydrogen or where weight is a significant factor, such as in aerospace.
Thermodynamics of Cryogenic Storage
The thermodynamics of cryogenic storage involves cooling hydrogen gas to extremely low temperatures (below -253°C) to convert it into a liquid form. This process significantly increases the energy density of hydrogen, allowing for more hydrogen to be stored in the same volume compared to gaseous form. Consequently, liquid hydrogen storage is advantageous for specific applications where space is at a premium or weight limitations exist.
A unique feature of liquid hydrogen is its efficiency in long-distance transportation, particularly over maritime routes. While the costs of liquefaction can occasionally be high, advancements in cryogenic technology continue to emerge, promising reductions in energy expenditure and improvements in logistics efficiency.
Challenges and Efficiency Issues
However, there are notable challenges accompanying liquid hydrogen storage. One of the pressing issues is the energy-intensive nature of the liquefaction process. It requires more energy to chill hydrogen than what could possibly be gained from the hydrogen itself post-storage, raising questions about net efficiency.
Additionally, evaporative losses, known as 'boil-off' hydrogen, can happen if the storage system is not properly insulated. This results in hydrogen loss and contributes to the overall cost. Thus, while liquid hydrogen storage can present a viable option under the right circumstances, stakeholders must carefully evaluate its feasibility based on specific operational needs and their associated energy impacts.
Metal Hydrides
Metal hydrides present an alternative storage solution, wherein hydrogen is absorbed into a host metal, forming a solid-state compound.
Mechanisms of Metal Hydride Formation
The mechanics of metal hydride formation can be quite fascinating. Hydrogen atoms react with certain metals to create metal hydrides, which possess a high volumetric storage density, significantly greater than that of compressed or liquid hydrogen. This unconventional approach showcases the ability of metals such as magnesium and lanthanum to absorb hydrogen in large quantities, making them ideal candidates for hydrogen storage systems.
This method's unique feature is its enhanced safety, as solid-state storage decreases risks associated with high-pressure gases. The stability of metal hydrides tends to exceed traditional storage methods, allowing for safe storage at lower pressures. Yet, the trade-off lies in the fact that generally, metal hydrides have slower kinetics for hydrogen release, which can sometimes pose challenges in applications needing rapid deployment of hydrogen.
Material Selection and Limitations
Nevertheless, selecting appropriate materials for metal hydride storage is complex. The hydrogen absorption and desorption rates are heavily influenced by the specific type of metal used. Limitations may arise from factors such as cost, availability, and potential toxicity of materials, which can dictate overall feasibility for certain projects. Moreover, as nickel and rare-earth metals can be prohibitively expensive, alternative materials are being actively researched, albeit with varying success. Thus, while metal hydrides offer a promising route to safer hydrogen storage options, comprehensive materials research must continue to propel this technology into practical applications.
Chemical Hydrogen Storage
Chemical hydrogen storage encompasses methods that involve the chemical bonding of hydrogen with various compounds, which can then be released when needed.
Types of Chemical Storage Solutions
This approach can take various forms, such as ammonia or liquid organic hydrogen carriers (LOHC). Chemical storage allows for higher energy density compared to other methods, allowing significant amounts of hydrogen to be stored in a more compact form. For instance, ammonia contains a high percentage of hydrogen by weight, making it an attractive option for global shipping and transport.
One important characteristic of chemical storage solutions is the potential for reversibility, meaning hydrogen can be released without extensive processing. This feature can facilitate easy integration with existing chemical industries, promoting synergies across sectors and enhancing overall uptake of hydrogen in the energy mix.
Energy Density Considerations
Energy density is a major consideration when evaluating chemical hydrogen storage methods. While the volumetric efficiency can be impressive, the processes needed to extract hydrogen can often be energy-intensive themselves. This must be scrutinized when these solutions are considered; balance is key. To that end, enhancing reaction kinetics and thermodynamics is a crucial area of research.
Moreover, the chemical stability of the storage compounds themselves is integral in discerning the practical applications of various systems. Some compounds may lead to challenging by-products or environmental concerns that must be addressed to ensure sustainable practices.
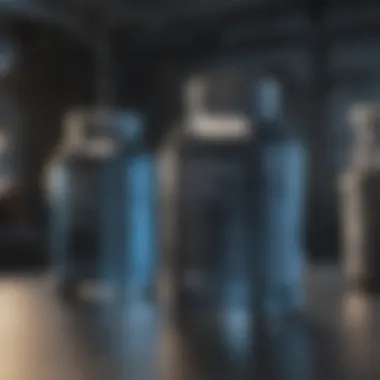
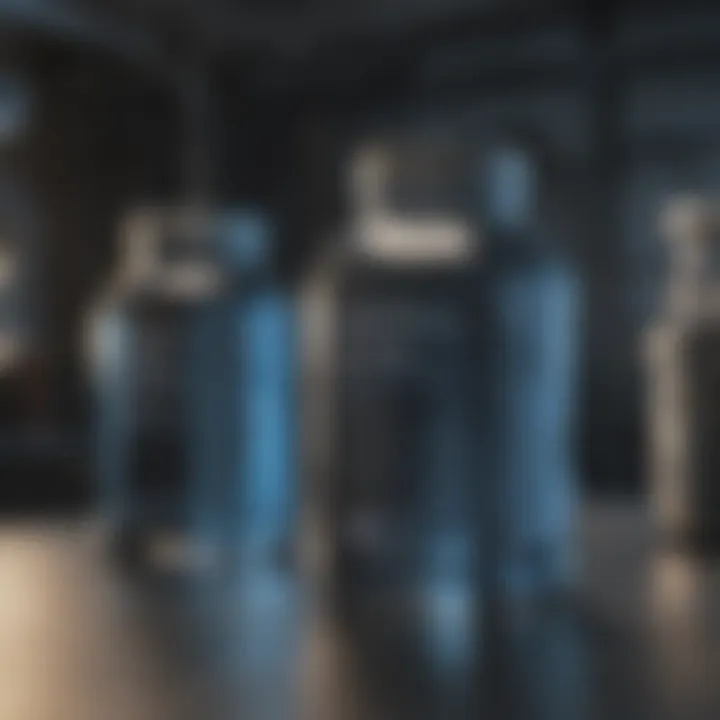
With the ongoing advances in the field, chemical hydrogen storage promises to unlock broader possibilities for hydrogen as an energy source, offering new pathways for cleaner energy innovations.
Evaluating Storage Technologies
As the realm of hydrogen energy development progresses, the evaluation of storage technologies becomes a cornerstone in our approach to fuel cells. At the heart of this topic lies the necessity to assess various methods based on performance, efficiency, and safety. By honing in on these factors, we can make informed decisions about which technology to implement, especially in a world where sustainability is paramount. An effective evaluation can not only enhance the viability of hydrogen as a mainstream fuel source but also align with broader energy goals by providing reliable and safe storage solutions.
Performance Metrics
Energy Density
Energy density is a critical metric when evaluating fuel storage methods. In this context, it's the amount of energy stored per unit volume or mass. The higher the energy density, the more efficient the storage system becomes, as it can hold more energy in a smaller space. This property is especially relevant for mobile applications, like vehicles and portable energy devices, where space is at a premium.
One key characteristic of energy density is its variation between different storage technologies. For instance, compressed hydrogen has a lower energy density compared to liquid hydrogen. However, liquid hydrogen presents challenges like the need for cryogenic temperatures, which complicates logistics. As a result, the unique feature of energy density influences the choice of storage system based on efficiency versus practicality.
Advantages of a high energy density solution include:
- Greater range for vehicles or equipment
- Reduced weight for transport applications
- Improved overall system performance
Yet, disadvantages can also manifest, such as cost implications, operational safety challenges, or infrastructural demands that come with maintaining high-pressure or cryogenic systems.
Cost Efficiency
Cost efficiency directly ties into the feasibility of any hydrogen storage system. It's essentially about getting the most bang for your buck â balancing performance against expenses. A storage technology that allows for high energy output with minimal initial investment and operational costs is often seen as a golden ticket for businesses and researchers alike.
Cost efficiency can mean different things depending on the context. For some, it might refer to the immediate price of materials and setup, while for others, it encompasses long-term savings, including maintenance, safety, and energy losses during storage. One unique feature of cost efficiency is the scale of production; larger facilities often realize reduced costs per unit through economies of scale.
Benefits of focusing on cost efficiency include:
- Longer-term viability of hydrogen as an energy source
- Attraction of investments in hydrogen technology
- Improved competitiveness against alternative energy sources
However, sometimes cost efficiency can stifle innovation. If researchers or companies merely cut corners to save money, they may overlook advances that enhance safety or efficiency.
Safety and Regulation
Given hydrogenâs flammability, safety and regulation are paramount when storing it. Industry standards and risk management protocols are the framework that aims to minimize hazards associated with hydrogen storage systems.
Industry Standards and Guidelines
Industry standards and guidelines play a crucial role in ensuring that hydrogen storage solutions are safe and effective. These are typically set by regulatory bodies, which offer frameworks for how to manage different storage systems. Adhering to them is not just about compliance but involves fostering trust among users and investors.
A notable characteristic is how these standards evolve; they must adapt to ongoing technological advancements and learnings from past incidents. This evolution can be seen in the increasing integration of safety features into design protocols.
The advantage of having solid industry standards is that it promotes uniform practices, enhancing safety across the board. However, strict adherence can sometimes lead to slowed implementation in the field as developers navigate regulatory hoops, often requiring significant paperwork and testing before approval.
Risk Management Protocols
Risk management protocols are essential in governing how risks associated with hydrogen storage are addressed. They encompass everything from assessing risks during the design phase to planning emergency responses in the event of a storage failure. Well-drafted protocols can significantly minimize potential hazards while ensuring that operators are prepared for unforeseen circumstances.
A significant aspect of risk management is its emphasis on continuous improvement. Protocols are regularly assessed and refined, which allows the industry to learn from near misses or accidents. They also involve collaboration with various stakeholders to chart out practical, comprehensive strategies that incorporate best practices.
Benefits of robust risk management include:
- Enhanced safety in hydrogen solutions
- Increased public and regulatory confidence
- Long-term sustainability of hydrogen technologies
Despite these positives, implementing risk management protocols can be burdensome, particularly for smaller organizations that may lack the resources to fully comply or adapt to comprehensive frameworks.
Understanding and implementing effective evaluation of storage technologies is not just a matter of choice; it's about laying the groundwork for a sustainable energy future.
Emerging Technologies in Hydrogen Storage
The pursuit of effective hydrogen storage solutions rests heavily on emerging technologies, which are crucial for advancing the use of fuel cells in various sectors. In a world clamoring for cleaner energy sources, the importance of how we store hydrogen cannot be overstated. Fuel cell technology, while promising, hinges on the ability to safely and efficiently store hydrogen. Without addressing the limitations of current storage methods, the potential for hydrogen to play a significant role in our energy landscape remains stymied. Emerging technologies not only promise efficiency but also sustainability, aligning well with contemporary environmental mandates.
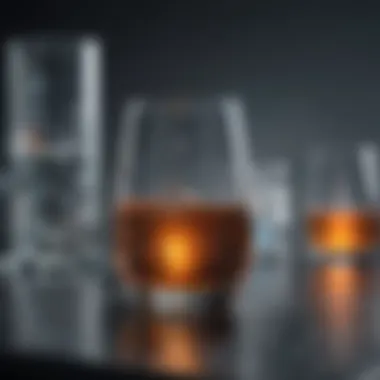
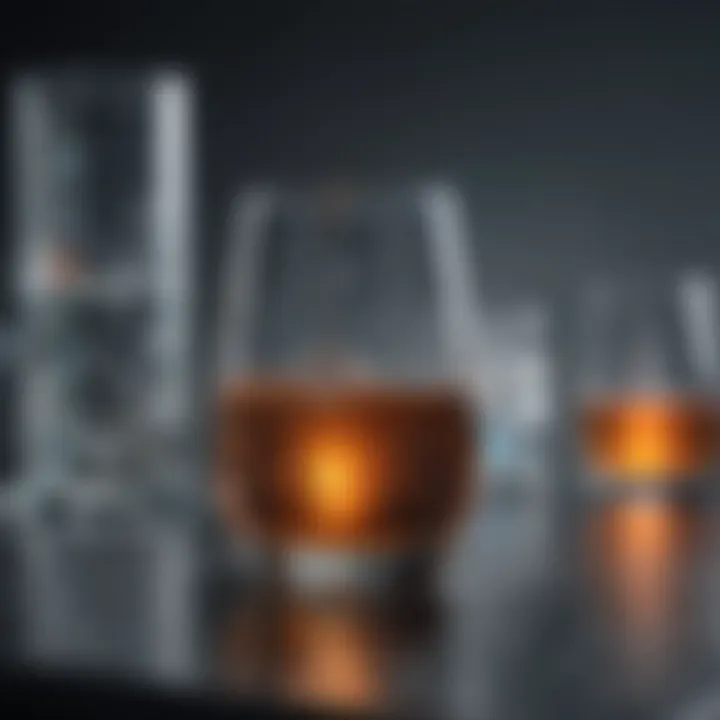
Advancements in Solid-State Hydrogen Storage
Recent strides in solid-state hydrogen storage present a transformative avenue worth exploring. Unlike traditional gas or liquid hydrogen storage methods, solid-state technologies utilize absorptive materials that can absorb and release hydrogen gas at different phases.
This approach inherently enhances safety since the solid materials present less risk of leaks compared to gaseous forms. Some of the key technologies showing promise in solid-state storage include:
- Metal Hydrides: These materials can absorb hydrogen atoms and release them when needed, making them suitable for applications in fuel cells.
- Complex Hydrides: They provide higher energy densities but often face challenges in terms of thermal stability and reaction kinetics.
- Frameworks and Composites: Novel structures like zeolites and MOFs (Metal-Organic Frameworks) are being engineered to hold hydrogen at ambient conditions, which can simplify storage and transport.
The prospect of solid-state storage is not just theoretical; prototype systems are being tested, laying a foundation for practical, commercial applications.
Nanomaterials and Their Potential
Approaching hydrogen storage through nanomaterials introduces innovative possibilities that challenge traditional methods. Nanotechnology can improve the efficiency and effectiveness of hydrogen storage systems significantly. Due to their high surface area and unique properties, nanomaterials can facilitate more effective absorption and release of hydrogen.
Key elements driving interest in nanomaterials for hydrogen storage include:
- Enhanced Adsorption Capacities: Nanomaterials can enhance the surface interactions for hydrogen storage, thereby increasing energy density.
- Customization and Functionalization: The ability to tailor nanomaterials for specific storage qualities adds versatility to hydrogen storage solutions.
- Environmental Impact: Nanomaterials often allow for lower thresholds of energy consumption during hydrogen absorption, contributing to a more sustainable approach.
"Nanomaterials in hydrogen storage not only evolve the performance metrics but also enhance the safety aspects traditionally associated with hydrogen."
As various industries align their strategies towards embracing hydrogen as a clean energy alternative, the development and optimization of emerging technologies in hydrogen storage is a fundamental component that will determine the viability of hydrogen fuel cells in the future. The ongoing research and experimentation with solid-state and nanomaterials showcase a pathway toward more reliable, cost-effective, and efficient hydrogen storage solutions, essential for achieving broader adoption.
Challenge of Scalability
When delving into hydrogen storage for fuel cells, the challenge of scalability emerges as a crucial focal point. Scaling up hydrogen storage technologies is not simply a matter of increasing production; it involves a complex interplay of several factors, including technology readiness, cost-effectiveness, and infrastructure development. Without addressing these critical elements, the broader adoption of hydrogen as an energy source could face considerable obstacles.
Scaling up means that systems which work on a small scale must also function efficiently and safely on a larger scale. Here, several considerations come into play:
- Cost Implications: As production scales, the cost of hydrogen storage solutions must not only remain competitive but also ideally decrease. This can be influenced by economies of scale, where larger production leads to lower costs per unit. However, initial investments in new technologies or facilities can be steep.
- Technological Limitations: What works well in a laboratory or pilot-scale environment may encounter unforeseen hurdles in a commercial setting. The transition from prototype to mass production often reveals nuances in performance that need to be adequately addressed.
- Infrastructure Requirement: A scalable solution requires proper infrastructureâstorage facilities, transportation systems, and distribution networks. Developing such infrastructure is a challenge in itself, often needing regulatory approvals and considerable investment.
Scalability is not just a technical hurdle; it also encompasses market dynamics. The demand for hydrogen necessitates a fluid transport system and effective market integration. It's essential to understand that these challenges are intertwined with societal acceptance of hydrogen technologies and their perceived viability as sustainable alternatives.
"The scalability of hydrogen storage technologies will define their role in the energy landscape of the future."
Current Market Landscape
Looking at the current market landscape, hydrogen storage technologies are at a pivotal stage. There is a noticeable increase in interest from both the public and private sectors, spurred on by a growing recognition of hydrogenâs potential as a clean energy carrier. Existing market players are exploring various pathways to enhance scalability, including public-private partnerships and grants that focus on innovative storage solutions.
Several key trends can be observed:
- Investment Boom: Venture capital is flowing into hydrogen storage technologies. This is more than a passing trend; major companies are seeing hydrogen as part of their future, which subsequently encourages startup ecosystems focusing on innovative storage solutions.
- Collaborative Efforts: Various sectors are collaborating to develop standardized solutions that can expedite scalability. For example, automakers and energy companies are working together to create infrastructures that make hydrogen readily accessible.
- Policy Support: Governments are providing incentives for research and development in hydrogen storage technologies. The EU, for instance, has laid out comprehensive roadmaps to reach hydrogen economy targets, fostering a conducive environment for market expansion.
In essence, the present market for hydrogen storage is a blend of optimism mingled with pragmatism. While we see promising advances, there remains a diffusion of readiness across different technologies and infrastructures waiting to be addressed.
Future Outlook for Hydrogen Storage Solutions
The future of hydrogen storage solutions holds tremendous promise and, at the same time, presents specific challenges that need to be navigated strategically. As technology evolves, there are several trajectories that research and development could take:
- Innovative Technologies: Emerging technologies such as advanced solid-state storage and innovative chemical compounds are expected to gain traction. These methods could potentially offer increased storage density and decreased safety concerns compared to traditional methods.
- Sustainable Practices: As sustainability becomes more pressing, hydrogen produced using renewable energy will likely gain importance. This could significantly affect storage techniques as they need to adapt to the dynamics of renewable energy generation, which is often intermittent.
- Standardization and Regulation: We anticipate enhanced regulation and standardization within the industry, which can significantly ease the process of scaling. This will be crucial in ensuring that safety and reliability are maintained as production volumes increase.
The overall outlook is optimistic, provided that all stakeholdersâresearchers, policymakers, and industry leadersâcollaborate efficiently. The long-term success of hydrogen storage will hinge on the ability to map out clear pathways toward commercialization while fostering an innovative spirit to tackle the existing challenges.
Epilogue and Implications for Future Research
The realm of hydrogen storage presents intriguing possibilities that could reshape the landscape of energy sustainability. As the push for cleaner energy alternatives continues to gain momentum, understanding how to effectively and safely store hydrogen becomes paramount. The journey through hydrogen storage technologies reveals a landscape often fraught with challenges but equally rich in potential breakthroughs.
Examining the methods discussed throughout this article, from compressed hydrogen and liquid states to metal hydrides and chemical storage solutions, highlights the versatility and challenges associated with hydrogen as an energy carrier. Each storage method carries unique advantages and disadvantages, not just in efficiency and cost but also in terms of safety and logistics. These factors must be meticulously weighed as research progresses.
Future research is poised to explore several critical avenues:
- Improvement of Current Technologies: Enhancing existing storage methods, such as improving the density and efficiency of metal hydrides or developing better safety protocols for liquid hydrogen, can lead to significant advancements in the field.
- Innovation in New Materials: The potential that nanomaterials hold is enormous. They may yield lighter, more efficient storage methods that could revolutionize how we approach hydrogen storage.
- Scalability of Solutions: It remains essential to focus on scalable technologies that can transition from laboratory experiments to commercial reality. Overcoming limitations in scalability can determine the success of hydrogen as a mainstream energy solution.
Moreover, the interplay of regulatory frameworks with technological advancements cannot be overstated. Aligning safety standards with innovative practical applications will ensure that hydrogen as a fuel can flourish without unnecessary inhibition.
"The efficiency with which we can store hydrogen today may dictate the energy conversations of tomorrow."
Moreover, as we navigate these avenues, collaboration across various sectorsâacademic, industrial, and governmentalâwill be crucial. The creation of partnerships can foster innovations that previously seemed unattainable. Furthermore, ongoing education and outreach about hydrogen technologies can aid in reducing public concerns around safety.
In summary, the implications for future research on hydrogen storage are profound. By continuing to innovate and explore this domain, we inch closer to a more sustainable energy future. The benefits are not merely theoretical; they can yield tangible returns in terms of energy availability, environmental sustainability, and ultimately, economic growth. Engaging in this pursuit equips us with the tools needed to harness hydrogen's full potential, ensuring its place in the mosaic of clean energy alternatives.