Freezing PBMCs: Methodologies and Challenges
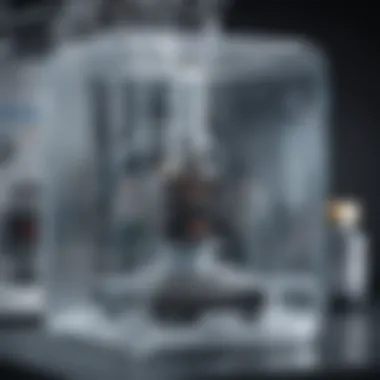
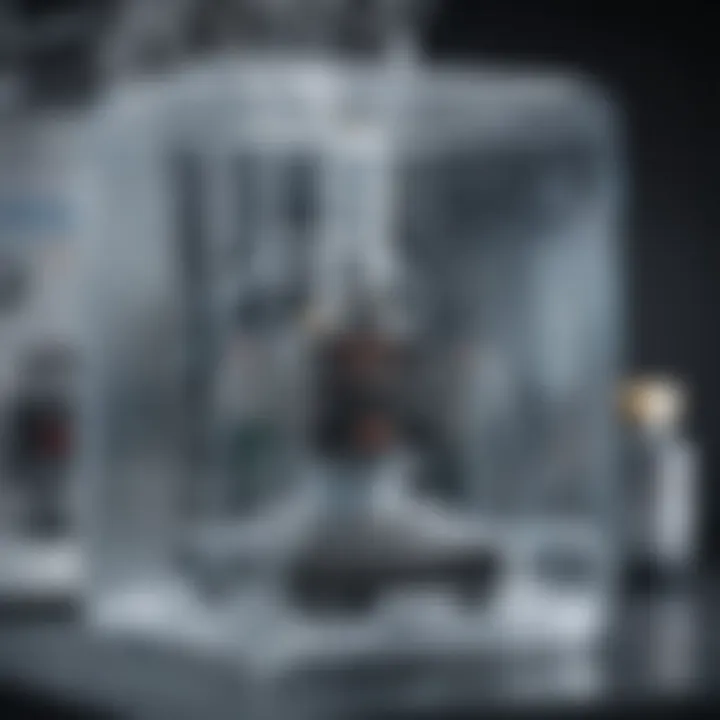
Intro
The cryopreservation of peripheral blood mononuclear cells (PBMCs) provides a critical method for cellular preservation. In biomedicine, PBMCs are invaluable. They are often used in immunological studies, vaccine development, and cancer research. Understanding how to effectively freeze these cells is fundamental for ensuring their viability and functionality in later applications.
Successful freezing of PBMCs involves considering various factors, such as freezing rates, cryoprotectants, and storage temperatures. This overview strives to guide readers through the intricacies of this process while addressing the significant impacts of freezing on cell quality and future research utilities.
Key Findings
Major Results
Freezing PBMCs affects cell viability and function. It can lead to significant loss during thawing unless proper protocols are followed. Key findings indicate:
- Optimal Freezing Rates: Properly controlled rates can reduce ice crystal formation, promoting cell integrity.
- Cryoprotectants: Dimethyl sulfoxide (DMSO) is widely used, but it requires careful handling due to its cytotoxicity.
- Thawing Protocol: Properly thawing cells is just as critical as the freezing process. Rapid thawing methods show better outcomes.
Discussion of Findings
The literature suggests the above are not just best practices; they are fundamentals for successful PBMC preservation. A study showed that with adequate cryoprotectant dosage and optimized freezing rates, PBMCs maintain over 70% viability post-thawing. However, variability exists. Different cell lines may demonstrate unique responses to the same protocols, thus necessitating tailored approaches in a laboratory setting.
Methodology
Research Design
An essential aspect of this overview is the inclusion of robust methodologies to study PBMC freezing. The research necessitates a combination of laboratory experiments, field studies, and data analysis. A blend of qualitative and quantitative methods provides a comprehensive understanding.
Data Collection Methods
Key data will be collected from:
- Laboratory Performances: Measuring cell viability and functionality post-thaw.
- Comparative Studies: Analyzing different freezing techniques across various PBMC types.
- Long-term Observations: Evaluating cell behavior over extended periods post-thaw.
"Optimizing the cryopreservation process enhances the overall quality of PBMCs, which is essential in research and therapeutic practices."
Prelude to PBMCs
Peripheral blood mononuclear cells (PBMCs) hold significant value in both research and clinical applications. Understanding PBMCs is crucial because they serve as a primary tool for immunological studies and various therapeutic interventions. In this section, we will explore the definition, composition, and the vital role PBMCs play in the world of immunology.
Definition and Composition
Peripheral blood mononuclear cells are a diverse group of cells found in the bloodstream, primarily consisting of lymphocytes and monocytes. Lymphocytes include T cells, B cells, and natural killer (NK) cells, while monocytes can differentiate into macrophages and dendritic cells. This composition is essential for understanding immune responses and disease mechanisms.
PBMCs are isolated from whole blood by the use of density gradient centrifugation. This method ensures that other cell types, such as erythrocytes and granulocytes, are separated from the PBMC layer. The purity and yield of PBMCs can vary depending on the method used for isolation. High-quality PBMCs are critical for reliable experimental outcomes in research settings.
Role in Immunology and Research
PBMCs are central components in the study of immune responses. They play an important role in recognizing and responding to pathogens, making them essential for vaccine development and infectious disease research. Moreover, PBMCs are often used in immunotherapy, which aims to harness the body’s immune system to fight diseases such as cancer.
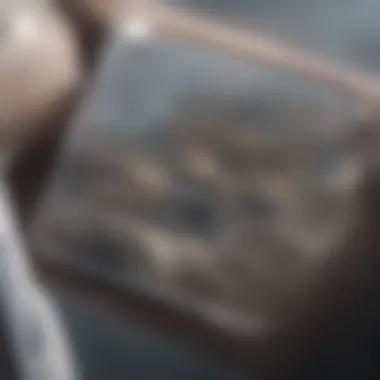
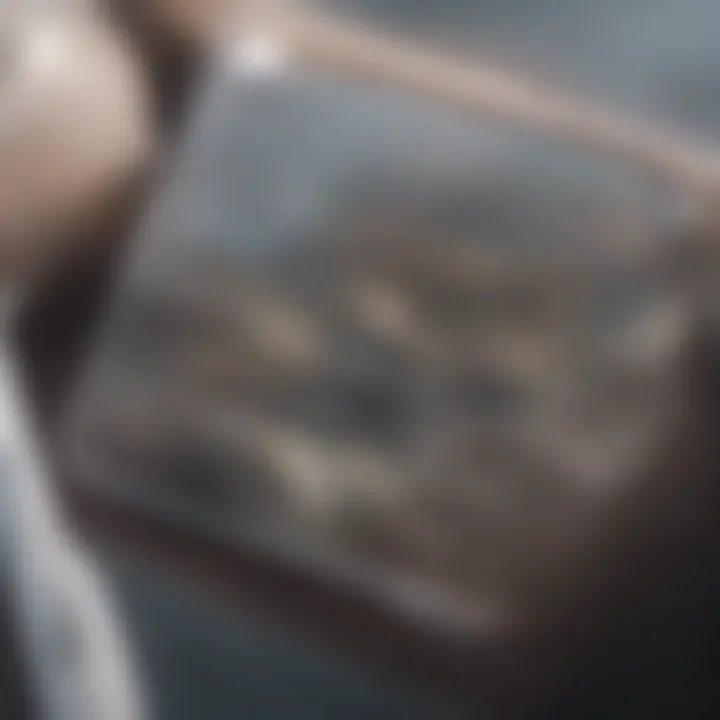
Furthermore, PBMCs serve as a model for understanding autoimmune diseases, where the immune system erroneously targets the body’s own tissues. By studying the behavior and function of PBMCs, researchers can gather insights into disease mechanisms and potential therapeutic targets.
"PBMCs are invaluable in advancing our understanding of the immune system, providing critical insights into both health and disease."
In summary, the importance of PBMCs cannot be overstated. Their unique composition and role in immune function make them indispensable for research in various fields related to health and disease. Understanding these cells is foundational for developing new treatments and enhancing existing therapies.
The Process of Freezing PBMCs
The freezing of peripheral blood mononuclear cells (PBMCs) is a critical process that ensures the viability and functionality of these cells for future use. PBMCs play a vital role in many immunological studies as they represent a rich source of lymphocytes and monocytes from the blood. The importance of this freezing process cannot be understated, as it directly impacts the outcomes of various experiments and clinical applications. Inadequate freezing techniques may lead to cell death or dysfunction, which can compromise research or treatment objectives. Thus, understanding the methodologies involved in freezing PBMCs is essential.
Isolation of PBMCs
Isolation of PBMCs is the first step in the freezing process. This procedure typically involves collecting whole blood, which can be done through venipuncture. The blood is then subjected to density gradient centrifugation, usually with a medium such as Ficoll-Paque. This method separates the PBMCs from other blood components like red blood cells and plasma.
During this process, the blood is layered on top of the gradient medium and centrifuged. Once centrifugation is complete, PBMCs will form a distinct layer at the interface between the plasma and the Ficoll. Careful collection of this layer is critical, as contamination with other cell types can affect viability and experimental results. After isolation, PBMCs are typically washed with a buffer solution to remove excess plasma and cryoprotectants.
Cryoprotectants: Importance and Selection
Cryoprotectants play a crucial role in the freezing of PBMCs. They help minimize ice crystal formation within cells, which can cause cryoinjury. The most commonly used cryoprotectant is dimethyl sulfoxide (DMSO). It effectively penetrates cells and prevents ice formation during the freezing process. However, DMSO's cytotoxicity is a significant consideration, warranting careful optimization of its concentration.
Another viable option is glycerol, which is less toxic than DMSO. Still, it may not provide the same level of cryoprotection. The choice of cryoprotectant may depend on the specific requirements of the downstream applications. It is essential to balance the desired cell viability and functionality against the potential toxicity of the cryoprotectants used.
Freezing Protocols: Step-by-Step Analysis
Implementing a standard freezing protocol is crucial for the successful cryopreservation of PBMCs. Here’s a simplified step-by-step analysis of an effective freezing protocol:
- Prepare the Cell Suspension: After isolating PBMCs, count the cells using a hemocytometer and dilute to the desired concentration, typically 1-2 million cells per milliliter.
- Add Cryoprotectants: Gradually add the selected cryoprotectant to the cell suspension. This is often done slowly to minimize osmotic shock.
- Aliquot Cells: Dispense the cell mixture into cryovials, ensuring uniform distribution across vials.
- Slow Freezing: Place cryovials in a controlled-rate freezer or a cryopreservation container with isopropanol and store at -80°C. Using a controlled freezing rate to go down to -1°C per minute is desirable. Alternatively, placing vials in a styrofoam box to gradually cool in a regular freezer can work if a controlled-rate freezer is unavailable.
- Storage in Liquid Nitrogen: Finally, if a long-term storage solution is required, transfer the vials to liquid nitrogen storage.
"Proper freezing protocols are essential for ensuring cell viability and functionality during subsequent analyses and applications."
Achieving optimal freezing conditions for PBMCs is a careful balancing act that can significantly influence the outcomes of subsequent experiments. By adhering to established protocols and considering the factors outlined during freezing, researchers can enhance their chances of preserving PBMCs in optimal condition for future use.
Factors Affecting Freezing Outcomes
Understanding the factors that impact the freezing outcomes of PBMCs is essential for optimizing cryopreservation protocols. These elements can significantly influence cell viability and functionality after thawing, which are critical for subsequent applications in research and therapy. This section discusses three main factors: freezing rate, cell type variability, and storage conditions and duration. Each of these factors plays a distinct role in achieving successful cryopreservation of PBMCs.
Impact of Freezing Rate
The rate at which PBMCs are frozen is one of the most crucial factors affecting the outcome of cryopreservation. A faster freezing rate is generally associated with the formation of smaller ice crystals, which cause less damage to cellular structures. In contrast, slow freezing tends to create larger ice crystals that can puncture cell membranes and lead to cellular death.
Optimizing the freezing rate involves balancing the temperature transition to ensure optimal cell morphology and functionality. For instance, freezing PBMCs at a rate of approximately 1°C per minute until reaching -80°C is commonly recommended. This gradual process minimizes the risk of cryoinjury. Researchers must also consider specific chilling protocols that can vary depending on the type of PBMC and the freezing container used.
Cell Type Variability
The variability among different cell types is another significant factor impacting freezing outcomes. PBMCs consist of several subtypes, including lymphocytes, monocytes, and dendritic cells. Each subtype exhibits unique sensitivities to the freezing process. For instance, lymphocytes may respond more favorably to cryopreservation compared to monocytes, which are more susceptible to damage during the freezing and thawing processes.
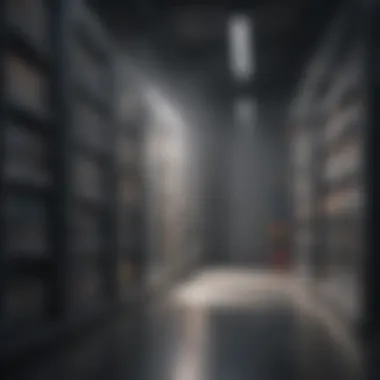
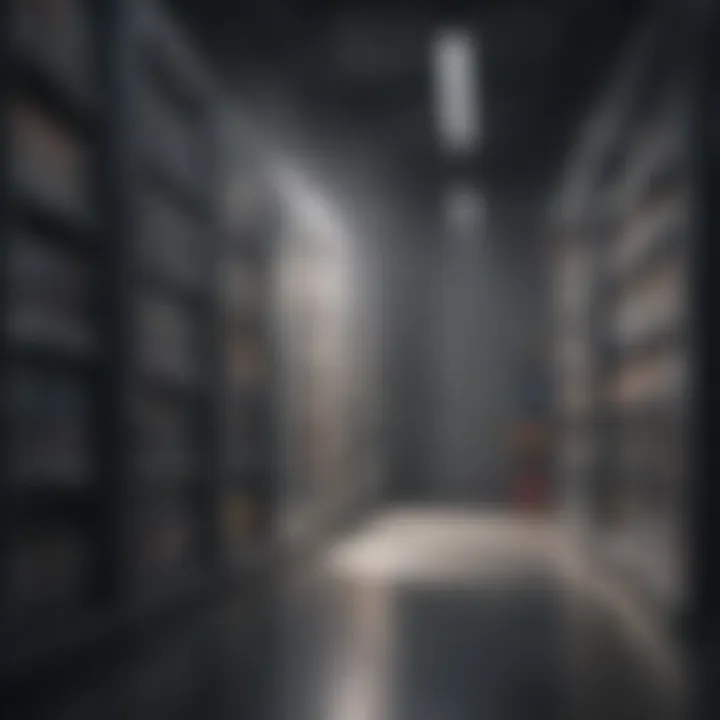
It is vital to consider not just the overall cellular composition but also the biological characteristics of the particular PBMC subtype being frozen. Understanding these differences can guide researchers in selecting appropriate cryoprotectants and freezing protocols to enhance viability and functionality post-thaw.
Storage Conditions and Duration
The conditions under which PBMCs are stored after freezing play a vital role in determining their viability over time. After the initial freezing, cells must be stored at low temperatures, typically at -196°C in liquid nitrogen. This ultra-low temperature drastically reduces metabolic activity and protects cells from degradation.
However, duration also comes into play. Although PBMCs can remain viable for extended periods when stored correctly, factors such as repeated freeze-thaw cycles can negatively impact cell health. Therefore, maintaining a controlled storage environment free from fluctuations is crucial. The following points outline best practices in this regard:
- Regular monitoring of storage temperatures
- Using appropriate storage containers that minimize risk of temperature fluctuations
- Limiting the number of freeze-thaw cycles to preserve cell integrity
Effective cryopreservation protocols are essential in ensuring the quality of PBMCs for downstream applications.
Assessment of Cell Viability Post-Freezing
Evaluating the viability of peripheral blood mononuclear cells (PBMCs) after freezing is critical. This assessment directly impacts the usefulness of PBMCs in various applications, such as immunological studies and therapeutic developments. Understanding how well the cells survive the freezing process can determine the outcome of subsequent experiments and their reliability. Furthermore, it provides insights into the effectiveness of different cryopreservation protocols.
It is not only the cell survival rate that matters, but also their functionality. Viable cells should exhibit proper physiological characteristics. Checking viability ensures that researchers work with a sample that can manifest the expected immunological responses. This assessment can reveal how freezing conditions affect immune cell behavior, which is vital for expounding the mechanisms underlying immune responses.
In summary, assessing cell viability after freezing informs researchers about the success of their cryopreservation methods. High viability rates correlate with reproducible outcomes in experiments. This section will discuss the various methods of viability testing and the functional assays used to analyze the performance of PBMCs once thawed.
Viability Testing Methods
There are several methods available for assessing the viability of PBMCs post-freezing. These include:
- Trypan Blue Exclusion Test: This simple dye exclusion test stains non-viable cells blue. Viable cells remain unstained, allowing for easy counting under a microscope.
- Annexin V/PI Staining: This technique utilizes annexin V to detect early apoptotic cells and propidium iodide (PI) for late apoptotic or necrotic cells. This dual-staining method provides a more detailed insight into cell health.
- Flow Cytometry: Utilizing fluorescent markers, flow cytometry enables precise identification of viable, apoptotic, and necrotic cells. It offers rapid results and high accuracy.
- Metabolic Assays: Tests such as the MTT assay measure cell metabolism by assessing the ability of viable cells to convert tetrazolium salts into formazan crystals, which can be quantified spectrophotometrically.
Each of these methods has its advantages and drawbacks, and the choice often depends on the required sensitivity and specificities for particular research contexts.
Functional Assays for PBMCs
Functional assays evaluate how viable PBMCs respond to stimuli post-thawing. The goal is to ascertain that not only are the cells alive but also functional. Some common types include:
- Cytotoxicity Assays: These assays measure a PBMC’s ability to kill target cells, indicative of their cytotoxic function. For example, the chromium release assay assesses the killing potential of cytotoxic T lymphocytes.
- Proliferation Assays: These tests, like the CFSE dilution assay, measure the ability of PBMCs to proliferate in response to specific antigens. This is crucial in understanding immune responses.
- Cytokine Production Assays: Analyzing the secretion of cytokines after stimulation helps assess the functional capability of PBMCs. Methods such as ELISA can quantify specific cytokines released during immune activation.
- Mixed Lymphocyte Reaction (MLR): This assay evaluates the reactivity of PBMCs against foreign cells, a measure of their role in adaptive immunity.
In summary, functional assays complement viability testing, providing a comprehensive view of how PBMCs respond after being frozen. Incorporating both viability testing and functional assessments ensures robust conclusions about PBMC health and readiness for research applications.
"Accurate assessment of PBMC viability and functionality can significantly influence the success of research outcomes and therapeutic applications."
Challenges in Freezing PBMCs
Freezing peripheral blood mononuclear cells (PBMCs) presents several challenges that researchers must navigate to ensure the effectiveness of cryopreservation. Understanding these challenges is essential because they directly affect cell viability, functionality, and the subsequent applications these cells can be used for in both research and clinical settings. Addressing these obstacles can lead to enhanced outcomes in cryopreservation techniques and, consequently, better data and therapeutic approaches.
Cryoinjury Mechanisms
Cryoinjury refers to damage that occurs to cells during the freezing process. This damage can impede the integrity and functionality of PBMCs when they are thawed for use. Several mechanisms contribute to cryoinjury:
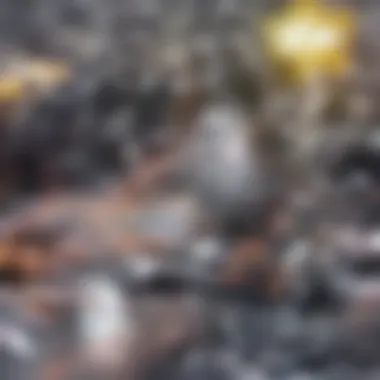
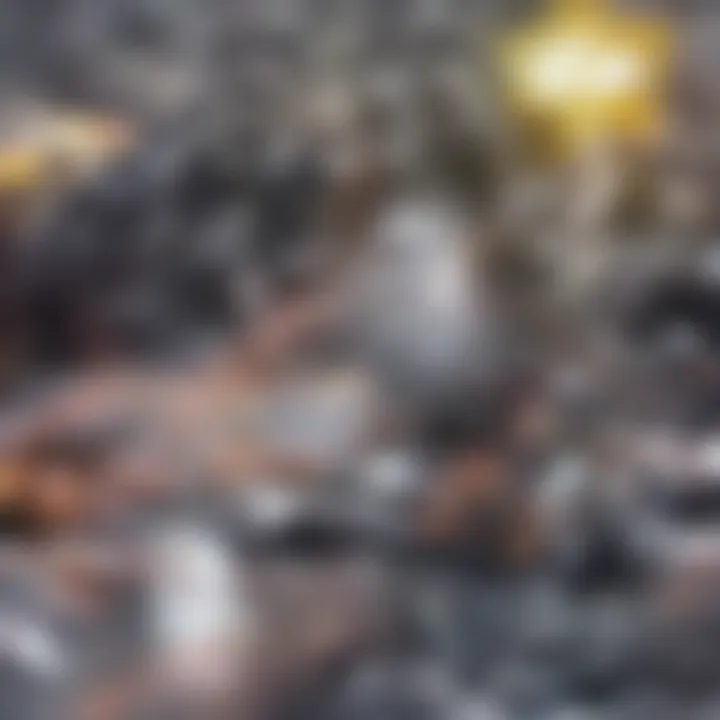
- Ice Crystal Formation: As temperatures drop, ice crystals can form inside and outside the cells. Intracellular ice formation can be particularly detrimental as it disrupts cellular structures, leading to lysis and cell death.
- Osmotic Shock: The movement of water in and out of cells during freezing and thawing causes osmotic changes. Rapid freezing may trap water inside cells, increasing the likelihood of damage when ice expands.
- Chemical Changes: Freezing can induce changes to cellular membranes, and the composition of cryoprotectants may also lead to toxicity. For example, dimethyl sulfoxide (DMSO) is commonly used but can have negative effects if not properly managed.
The interplay of these factors highlights the complexity of freezing PBMCs. Researchers must implement careful protocols to mitigate cryoinjury and maximize cell recovery rates.
Variability in Cryopreservation Techniques
Different cryopreservation techniques can yield varying results. This variability can stem from several factors:
- Temperature Control: The rate of cooling is critical. Slow cooling can lead to more significant cryoinjury, while faster cooling may lead to better outcomes but also increases the risk of intracellular damage. Precise temperature management is vital.
- Cryoprotectants: As previously mentioned, the type and concentration of cryoprotectants play a crucial role. Not all cryoprotectants behave the same way, and their efficacy can differ based on the specific type of PBMCs being frozen.
- Cell Density: The concentration of PBMCs during the freezing process can also affect outcomes. High cell densities may cause cell-cell interactions that can complicate the freezing and thawing processes.
- Handling Techniques: The proficiency of personnel performing the cryopreservation can influence the quality of the outcomes. Variations in handling techniques can unintentionally introduce inconsistencies.
"Understanding the intricacies of cryoinjury and variability in preservation techniques is crucial in optimizing PBMC storage protocols."
Innovations in PBMC Cryopreservation
The topic of innovations in PBMC cryopreservation holds critical importance in enhancing the viability and functionality of preserved cells. As the field of biomedical research expands, the need for more efficient and effective cryopreservation techniques is evident. New methods can lead to better survival rates of PBMCs post-thawing, which is vital for various downstream applications such as immunological studies, vaccine development, and cellular therapies. Innovative strategies address common challenges in cryopreservation, such as cryoinjury and variability in outcomes.
Emerging Technologies and Methods
Recent advances in technology and methodology are revolutionizing how PBMCs are cryopreserved. One significant improvement has been the refinement of cryoprotectant agents. Novel formulations are being developed that may reduce toxicity while enhancing cellular stability during the freezing process. For instance, the use of hydroxyethyl starch (HES) in combination with dimethyl sulfoxide (DMSO) is garnering attention for its potential to confer better protection to cells.
Another area of innovation involves controlled-rate freezers and other automated devices which enhance the freezing rate of cells. By carefully regulating temperature declines, these technologies minimize the formation of ice crystals within cells, thereby reducing the risk of damage. In addition, non-thermal methods, such as vitrification, are also under investigation. Vitrification involves transforming the cell solution into a glass-like state and has demonstrated promise in achieving higher post-thaw viability.
Potential Applications of Improved Techniques
The advancements in PBMC cryopreservation technology are expected to yield numerous applications. One key area is personalized medicine. With better-preserved PBMCs, clinicians could obtain more accurate information from immune profiling tests, leading to tailored therapeutic interventions.
Moreover, these improved techniques facilitate large-scale biobanking efforts. As researchers increasingly rely on archived PBMCs for longitudinal studies, ensuring high-quality samples is essential. Additionally, the implications extend to vaccine research and development. Higher viability of preserved PBMCs allows for more robust assessments of immune responses to vaccines.
In summary, the ongoing innovations in PBMC cryopreservation not only address existing challenges but also open new avenues in clinical and research settings. By optimizing the cryopreservation process, researchers can significantly enhance the utility of PBMCs and improve outcomes in various applications, thus ultimately furthering progress in the biomedical field.
"In the realm of biomedical research, the viability of preserved PBMCs can directly impact the outcomes of crucial studies. Therefore, advancements in cryopreservation techniques are invaluable."
For more detailed information on related topics, you may visit Wikipedia and Britannica.
Finale and Future Perspectives
The freezing of PBMCs represents not only a pivotal technical aspect in cell preservation but also plays a crucial role in various biomedical applications. Successful cryopreservation ensures that PBMCs retain their integrity and functionality for future research and clinical uses. This section will recap the importance of this subject and look ahead to potential advancements that can enhance PBMC preservation techniques.
Recap of Importance
In summary, freezing PBMCs is essential due to its wide-ranging implications in immunology and therapeutic research. Proper freezing protocols can lead to high cell viability and functionality, making these cells valuable for diagnostic and therapeutic manipulations. It is also critical for maintaining the quality of biological samples collected for long-term storage. Any damage caused during the freezing process can hinder research outcomes and clinical applications, reinforcing the need for stringent adherence to optimal cryopreservation techniques. Thus, understanding the methods and factors associated with freezing PBMCs is not merely an academic need but a practical requirement for researchers in the field.
Future Directions in Research
Looking ahead, research on PBMC freezing methodologies is expected to further evolve. Some key areas likely to receive attention include:
- Improvement of Cryoprotectants: New formulations may enhance cell survival post-thaw by minimizing crystalline ice formation. Innovative cryoprotectants might also reduce cellular damage associated with traditional methods.
- Methodological Refinements: Enhanced freezing protocols that incorporate controlled-rate freezers or automated systems could lead to more uniform freezing outcomes.
- Understanding Mechanisms of Cryoinjury: More research may be needed to fully understand how freezing affects PBMCs at the molecular level. This understanding can drive innovations in preservation techniques.
- Field Applications: Application of improved techniques in real-world settings, including personalized medicine, should be pursued to validate these innovations.
Emerging technologies, such as biobanking and improved storage solutions, may further augment the utility of PBMCs. The push for precision medicine will require scientists to constantly evaluate and update PBMC preservation techniques.
The importance of maintaining cell viability through effective freezing cannot be understated, as it influences not only individual research but also collective advancements in therapeutic approaches.
Overall, the future of PBMC cryopreservation holds promise for innovations that will significantly enhance research and therapeutic endeavors. The insights gained from understanding freezing techniques may eventually lead to more effective applications in clinical settings, ultimately benefiting patient outcomes.