Exploring Ultra Wide Band Gap Semiconductors
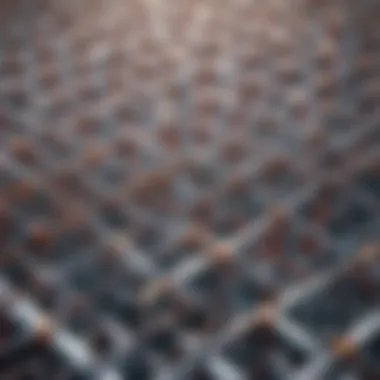
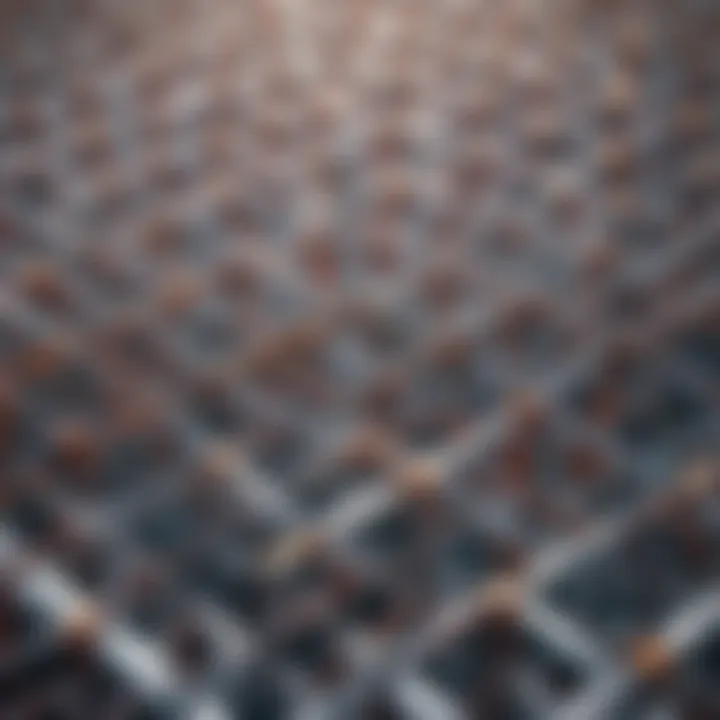
Intro
The exploration into ultra wide band gap semiconductors reveals a captivating interplay of physics and engineering that has taken the scientific community by storm. These semiconductors, defined by their extraordinary band gap properties, present opportunities that extend far beyond traditional materials like silicon. The potential applicability spans across diverse sectors including electronics, renewable energy, and communication technologies. As we peel back the layers of this topic, it becomes evident that we are at the cusp of a new era within semiconductor technology, one that promises enhanced performance and efficiency.
This journey leads us to understand not just the characteristics of ultra wide band gap semiconductors, but also the underlying principles that govern their behavior. The unique properties of materials like gallium nitride (GaN) and silicon carbide (SiC) illustrate just how significant these materials can be when harnessed effectively. But as intriguing as their potential is, it's important to address the trials and tribulations that researchers face in this domain.
As we traverse through the subsequent sections, we will take a closer look at their properties, the methodologies involved in their fabrication, and the various applications that could shape future innovations. We will further analyze the challenges currently plaguing research in this field and the ongoing efforts aimed specifically at overcoming these hurdles. With a blend of technical detail and broader implications, this article aims to provide a thoughtful discourse for educators, students, and professionals alike.
Prelude
Ultra wide band gap semiconductors have carved out their own niche in the ever-evolving landscape of materials science and technology. As we kick things off with this exploration, one must understand that these semiconductors are not merely a footnote in the larger saga of electronic materials; they are pivotal in addressing some of the most pressing challenges of our time, particularly in the realm of energy efficiency and high-performance electronics.
Definition of Ultra Wide Band Gap Semiconductors
Ultra wide band gap semiconductors refer to materials that exhibit a band gap greater than 3 eV, positioning them significantly apart from conventional semiconductors like silicon, which typically fall within the 1 to 2 eV range. To put it simply, the larger band gap allows these materials to withstand higher voltages and temperatures without breaking a sweat. This characteristic is especially crucial when considering applications that demand robust performance under severe conditions, such as power electronics and high-frequency devices.
In essence, the term refers to the energy required to excite an electron from the valence band to the conduction band, providing a gauge of how effective the material is in conducting electricity. This feature is directly related to the ability to operate in extreme environments without degradation.
Importance in Modern Technology
The relevance of ultra wide band gap semiconductors in today's technological landscape cannot be understated. For instance, in power electronics, they offer the promise of significantly enhanced efficiency with minimal energy loss, a consideration that is increasingly vital as industries push toward greener practices. Imagine a world where electric vehicles can charge faster and last longer; these materials are key to making that vision a reality.
Moreover, the capacity to operate at higher temperatures provides a gateway to new applications in aerospace and defense sectors where electronic devices endure extreme environments. The potential to enhance the reliability of power systems means fewer failures and reduced maintenance costs.
Several points can illustrate their significance further:
- High Efficiency: They provide advantages in energy conversion, which aligns with global energy goals.
- Robust Performance: Their durability translates to stability in conditions that would typically compromise other materials.
- Emerging Applications: Research continues to unfold novel uses, expanding their role in fields like optoelectronics and renewable energy.
"The advancements in ultra wide band gap semiconductors represent a cornerstone for future energy systems, marrying the need for efficiency with the capabilities of modern technology."
Wielding such potential, these semiconductors are not just a theoretical concept or an academic focus—they are emerging as tangible solutions to enhance and innovate across various industries.
Fundamental Properties
Understanding the fundamental properties of ultra wide band gap semiconductors is essential for comprehending why these materials hold such potential in modern technology. Their unique characteristics set them apart from traditional semiconductors, making them particularly beneficial for certain applications. In this section, I'll explore some critical elements: energy band structure, electrical conductivity, and thermal properties. Each of these aspects plays a pivotal role in how ultra wide band gap semiconductors can be applied effectively across various industries.
Energy Band Structure
The energy band structure of a semiconductor describes the range of energy levels that electrons may occupy. Ultra wide band gap semiconductors have a significantly larger band gap compared to conventional semiconductors like silicon. Typically, band gaps greater than 3 eV are observed in materials like gallium nitride and silicon carbide.
A larger band gap enhances the ability to operate under high temperatures and high voltages while minimizing leakage current. This feature is crucial for applications such as power electronics where high efficiency is prioritized. The following points highlight the implications of this unique band structure:
- Improved efficiency: Less energy lost as heat.
- Enhanced performance: Can function in more extreme conditions without failure.
- Diverse applications: Usable in high-power and high-frequency devices, making them versatile across multiple sectors.
"The broader the band gap, the greater the potential for disruptive technologies."
Electrical Conductivity
Electrical conductivity in ultra wide band gap semiconductors might seem counterintuitive at first. Although their wider band gap means they generally act as insulators at room temperature, they become conducting in higher temperatures or when doped correctly. This ability to toggle between conducting and insulating states lends itself to a variety of applications.
Characteristics to consider include:
- Doping: Adding impurities can enhance conductivity, enabling controlled electronic properties.
- Temperature Effects: As temperature increases, electron mobility may improve, allowing for conduction despite the large band gap.
- Reduced power losses: Lower intrinsic carrier concentration leads to efficient devices that can save energy in applications.
Thermal Properties
Thermal properties are equally important in defining the capabilities of ultra wide band gap semiconductors. Because they can operate at high temperatures, they find their niche in high-performance applications. This can be attributed to several factors:
- High thermal conductivity: Materials like silicon carbide can dissipate heat effectively, preventing device overheating.
- Thermal stability: Their robust nature means they remain stable and efficient under increased operational temperatures, unlike traditional semiconductors that may degrade.
- Potential for miniaturization: Excellent thermal properties allow engineers to design smaller, more compact electronics without desirable performance losses.
With all these properties combined, ultra wide band gap semiconductors stand at the forefront of a technological revolution. By understanding these fundamental properties, stakeholders in various fields can effectively leverage their advantages for innovations in semiconductor technology.
Comparison with Traditional Semiconductors
When discussing semiconductors, contrasting ultra wide band gap (UWBG) semiconductors with their traditional counterparts is crucial. This comparison highlights not just the advancements brought forth by UWBG materials, but also the areas where they excel beyond more familiar semiconductors like silicon and gallium arsenide. The insights derived from this examination can clarify why further investment in UWBG technology is both necessary and beneficial.
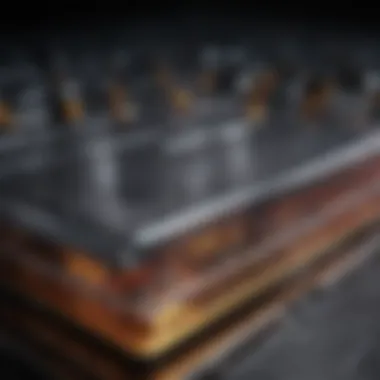
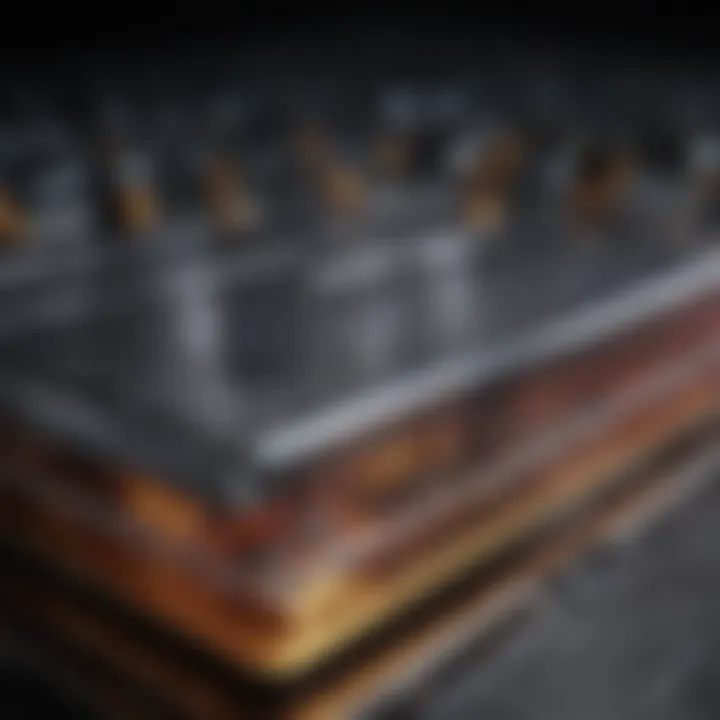
Band Gap Differences
One of the most notable distinctions between UWBG semiconductors and traditional semiconductors is found in their band gap widths. Traditional semiconductors typically feature band gaps that hover around one to two electronvolts. For instance, silicon has a band gap of about 1.12 eV while gallium arsenide has a band gap of 1.42 eV.
In contrast, UWBG semiconductors boast band gaps exceeding three electronvolts. Gallium nitride, for instance, has a band gap around 3.4 eV. This significant difference offers a slew of advantages:
- Higher Breakdown Voltage: This allows for devices to operate at higher voltages, minimizing the risk of failure.
- Reduced Leakage Current: With a wider band gap, these semiconductors can perform more efficiently, particularly in high-temperature environments.
Such properties lend themselves well to advanced power electronics and high-frequency applications, which remain challenging for traditional semiconductors.
Material Durability and Stability
When it comes to material durability and stability, UWBG semiconductors clearly have the upper hand. One core factor to consider is their chemical and thermal resilience. For example, silicon carbide can withstand more punishing environments compared to silicon. This characteristic leads to increased reliability in applications involving power supplies and automotive systems.
- Corrosion Resistance: Unlike traditional materials, UWBG semiconductors are less susceptible to corrosion which usually arises from environmental factors.
- Thermal Conductivity: Higher thermal conductivity permits better heat dissipation, so devices made from these materials tend to operate cooler and maintain performance integrity.
Conclusively, durability translates to longer service life, which is a critical element for sectors such as manufacturing and aerospace.
Temperature Tolerance
Temperature tolerance is another area where UWBG semiconductors shine brightly against traditional silicon. Standard semiconductors have a more limited thermal operating range, which can lead to issues like thermal runaway in extreme conditions. In contrast, UWBG materials, such as silicon carbide and gallium nitride, can function effectively in high-temperature environments, sometimes reaching above 200 °C.
Here are some noteworthy points:
- Wide Operational Range: This allows UWBG devices to be employed in a variety of applications, from automotive electronics to high-performance computing.
- Improved Safety: Devices that operate at elevated temperatures reduce the chances of component failure, leading to safer systems overall.
- Energy Efficiency: Running at higher temperatures can potentially improve overall efficiency, a key consideration as industries push toward greener technologies.
The ability of ultra wide band gap semiconductors to outperform traditional materials in band gap width, durability, and temperature tolerance positions them as the logical choice for next-generation technological solutions.
Fabrication Techniques
The fabrication techniques used for ultra wide band gap semiconductors are crucial to their performance and applicability. These methods not only determine the quality and characteristics of the semiconductor materials but also influence their cost-effectiveness and scalability for commercial use. As industries seek efficient and robust components, understanding these techniques is paramount. The choice of fabrication method can have profound implications on material properties such as crystallinity, defect density, and overall electrical performance, thus affecting their success in real-world applications.
Molecular Beam Epitaxy
Molecular Beam Epitaxy (MBE) is a precise fabrication method that enables the growth of semiconductor layers one atomic layer at a time. This technique involves the deposition of elemental and compound atomic beams onto a substrate in a high vacuum environment. MBE allows for exceptional control over thickness and composition, which results in high-quality films. This precision is particularly beneficial for constructing complex multilayer structures or quantum dot systems, often used in optoelectronic devices.
One could think of MBE like baking a cake. If you layer your ingredients correctly, you get a beautiful result. However, if the layers are uneven or poorly mixed, the outcome can be less than desirable. Similarly, using MBE correctly leads to semiconductors that exhibit outstanding electronic properties.
"The epitaxy process can be as delicate as fine art, where every brush stroke matters in achieving the perfect finish."
Hydride Vapor Phase Epitaxy
Hydride Vapor Phase Epitaxy (HVPE) is another technique gaining traction in the fabrication of ultra wide band gap semiconductors. In HVPE, materials are deposited from a vapor phase source, often involving hydride gases, to produce high-purity layers on a substrate. This method is notable for its ability to produce large area substrates quickly and is particularly advantageous for materials such as Gallium Nitride.
A relative strength of HVPE lies in its scalability and potential for production automation. As demand grows, being able to efficiently produce high-quality materials is essential. Think of this method like a factory assembly line. It’s designed to crank out quantities without sacrificing quality.
Chemical Vapor Deposition
Chemical Vapor Deposition (CVD) is among the most widely adopted methods for the fabrication of ultra wide band gap semiconductors. CVD involves chemical reactions that occur in a vapor state, resulting in the deposition of a solid material onto a substrate. This technique can be adjusted to manipulate various parameters, including pressure, temperature, and gas composition, allowing researchers and engineers to tailor the semiconductor properties.
Using CVD is like gardening; the conditions you set up will determine how well your plants (semiconductors) grow. If the environmental factors aren’t right, the yields can be poor. Therefore, maintaining optimal conditions during the deposition process is vital for achieving desired material characteristics such as uniformity and crystal structure.
In summary, each fabrication technique holds specific benefits and challenges. As researchers continue to refine these processes, the door opens wider for the integration of ultra wide band gap semiconductors across various innovative applications.
Key Materials in Ultra Wide Band Gap Semiconductors
The realm of ultrawide band gap semiconductors is fascinating mainly due to the unique materials that comprise it. These materials are not just random choices; they play pivotal roles in defining the performance and functionality of devices. Each of these materials brings a set of specific advantages, making them suitable for particular applications. Understanding the properties and behaviors of these key materials—Gallium Nitride, Silicon Carbide, and Zinc Oxide—is essential for any researcher or professional in the field. Their significance lies in the ability to push the envelope in electronic, optoelectronic, and energy solutions.
Gallium Nitride
Gallium Nitride (GaN) has emerged as a powerhouse in the semiconductor world, primarily due to its high thermal conductivity and ability to operate at elevated temperatures. The notable feature of GaN is its wide band gap of about 3.4 eV, which facilitates high-frequency devices. This property has made it a favored choice in power electronics and RF applications. The increasing use of GaN for power transistors and diodes reflects its capacity for efficiency and compact design—two characteristics that are vital in modern electronic systems.
Moreover, GaN enables devices to switch faster, which is critical for minimizing energy losses. As the demand for energy-efficient devices continues to rise, GaN solidifies its position as a cornerstone material in the future of electronics. Additionally, its potential for integration with silicon substrates will further broaden its application range, making it a topic of intense research and development.
Silicon Carbide
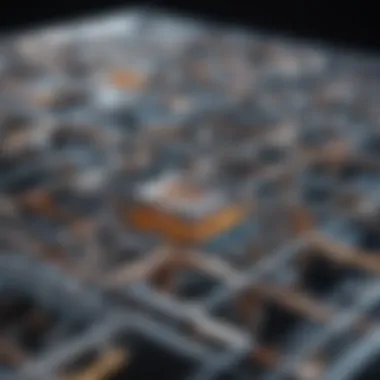
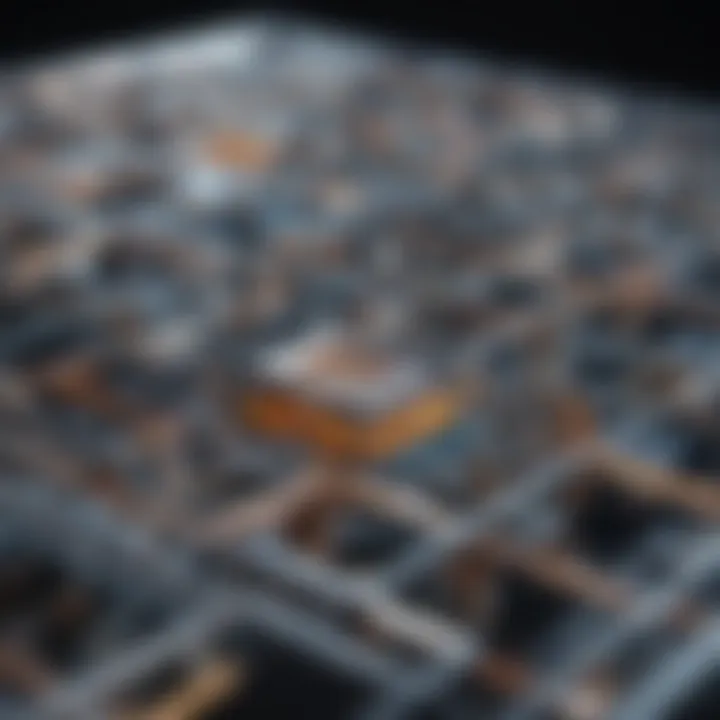
Then there’s Silicon Carbide (SiC), which is like the Swiss Army knife of semiconductors. SiC has a band gap of around 3.26 eV, granting it substantial advantages over traditional silicon. It’s particularly well-regarded for its robust thermal stability and chemical resistance—traits that are invaluable in harsh environments. In sectors like automotive and aerospace, where reliability is key, SiC shines bright.
Its performance in high-power and high-temperature applications is hard to overlook. For instance, in electric vehicles, SiC is used in the inverters that convert DC to AC, which boosts overall efficiency and minimizes losses. This performance has already established its reputation on the industrial scale, hinting that this material is not just a temporary trend, but a necessary evolution in semiconductor technology.
Zinc Oxide
Last but not least, we have Zinc Oxide (ZnO). Known for its direct band gap of about 3.3 eV, ZnO is often celebrated for its versatility. This material is gaining traction particularly in optoelectronics due to its capability to emit light efficiently. ZnO is utilized in light-emitting diodes and laser diodes. However, its flexibility doesn’t stop there; it has potential applications in solar cells and transparent conductive coatings, making it quite valuable in renewable energy technologies.
Another attractive aspect is its eco-friendliness compared to other semiconductor materials. With the increasing focus on sustainability, ZnO’s merits make it worth serious consideration for future innovations. As research continues, the material’s full capabilities are slowly coming to light, highlighting the exciting possibilities it may unleash in various industries.
In summary, the key materials in ultrawide band gap semiconductors—Gallium Nitride, Silicon Carbide, and Zinc Oxide—are crucial for advancing technology across multiple domains, reflecting their individual strengths and diverse applications.
By honing in on these materials, researchers and industry professionals can unlock the potential for smarter, more efficient electronic and energy systems.
Applications in Various Industries
The vast potentials of ultra wide band gap semiconductors (UWBG) hold significant relevance across multiple industrial sectors. The unique properties of these materials, such as high thermal conductivity, large breakdown field, and excellent radiation hardness, make them ideal candidates for various applications. This section explores how UWBG semiconductors are transforming power electronics, optoelectronics, and the renewable energy sectors, each of which contributes substantively to technological advancement and sustainability.
Power Electronics
When it comes to power electronics, the transition from conventional silicon-based devices to UWBG semiconductors has been a game-changer. Silicon carbide (SiC) and gallium nitride (GaN) are two prominent materials in this field, enabling devices that can operate at higher voltages, temperatures, and frequencies. This not only leads to increased efficiency but also allows for smaller, lightweight designs in power supplies and converters.
- Key benefits of UWBG in power electronics include:
- Higher efficiency in power conversion, reducing energy losses considerably.
- Enhanced thermal performance, allowing devices to operate at elevated temperatures without degradation.
- Smaller form factors, which is crucial for applications ranging from electric vehicles to renewable energy systems.
Furthermore, as demands for electric vehicles (EVs) and renewable energy systems continue to soar, the need for robust and efficient power management solutions becomes paramount. UWBG semiconductors play a vital role in enabling faster charging and better performance of these systems.
"The shift to UWBG materials is paving the way for a new era of power efficiency and miniaturization that can impact everything from smartphones to electric utilities."
Optoelectronics
In the realm of optoelectronics, including light-emitting diodes (LEDs) and laser diodes, UWBG semiconductors are carving out a significant niche. Gallium nitride, in particular, has transformed the landscape of blue and ultraviolet light sources, which are essential in numerous applications, from consumer electronics to advanced medical devices.
The importance of UWBG in optoelectronics can be summarized as follows:
- Improved efficiency and brightness in LEDs, reducing power consumption and enhancing durability—leading to longer lifespans for lighting applications.
- Development of cutting-edge laser diodes, capable of emitting coherent light across short wavelengths, important for applications in spectroscopy and communications.
- Contribution to telecommunications technology, especially in fiber optics, where UWBG materials are used to create high-frequency components that boost data transmission rates.
With advancements in optoelectronic devices using UWBG materials, there is a marked increase in the quality of display technologies and lighting solutions, driving further innovations.
Photovoltaics and Renewable Energy
The integration of UWBG semiconductors in photovoltaics represents a progressive leap in solar technology. By utilizing materials like perovskites, researchers are working to develop solar cells that not only harness greater amounts of solar energy but also operate efficiently under varying environmental conditions.
Specifically, UWBG materials offer:
- Higher energy conversion efficiencies, theoretically surpassing the limits of conventional silicon-based solar cells.
- Enhanced thermal stability, which reduces efficiency losses in high-temperature environments, making solar power feasible even in harsher climates.
- The potential for flexible solar panels, which could revolutionize how and where solar energy can be harvested.
In sum, ultra wide band gap semiconductors are vital to the advancement of renewable energy technologies. Their contributions to more efficient and durable solar solutions are helping to reduce carbon footprints and promote sustainability.
As industries continue to evolve, UWBG semiconductors will increasingly become foundational to various new technologies, paving the way toward a greener and technologically advanced future.
Current Challenges in Development and Implementation
The journey towards establishing ultra-wide band gap semiconductors as a mainstream technology is not without hurdles. It involves a delicate balance of performance, cost, and integration capabilities. This section dives into the pressing challenges that need to be addressed to harness the full potential of these materials in diverse applications.
Manufacturing Costs
One primary obstacle plaguing the advancement of ultra-wide band gap semiconductors is the high manufacturing costs. Producing materials like Gallium Nitride and Silicon Carbide often involves expensive raw materials and complex fabrication processes. The higher the band gap, the more stringent the production requirements become. For instance, while traditional semiconductors like silicon can be produced at a relatively low cost, the same cannot be said for ultra-wide band gap alternatives. This discrepancy poses a significant barrier, especially for industries that are cost-sensitive.
- Material and Equipment Costs: The equipment used in processes such as Molecular Beam Epitaxy and Chemical Vapor Deposition itself can be prohibitively priced, adding to the final product's cost.
- Yield Rates: The production yield of high-quality wafers is often low, which can inflate the costs further. If you end up with many non-viable chips from a batch, it can severely impact profitability.
As a result, there is an urgent need for research in reducing these manufacturing costs. Finding more efficient techniques or alternatives could help make these semiconductors more accessible for widespread use.
Material Quality Control
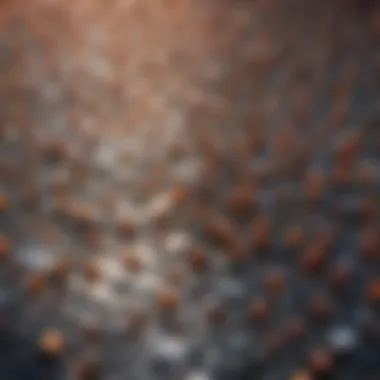
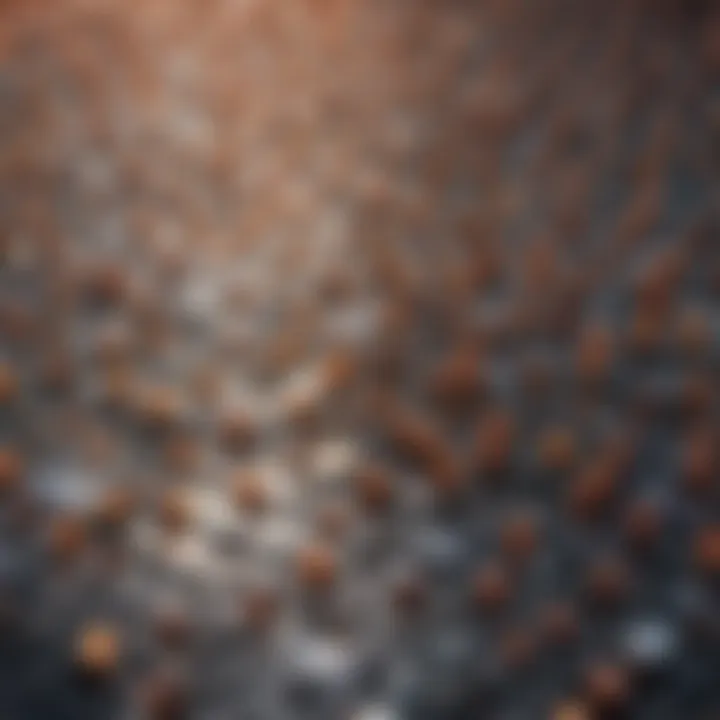
Quality control is another critical factor in the development and implementation of ultra-wide band gap semiconductors. The properties of these materials can vary significantly with minute changes in the fabrication process. Any defects or inconsistencies in the material can lead to subpar electrical performance or thermal stability issues.
For example, even slight variations in impurity levels during the growth phase can affect the electron mobility, thus hindering the semiconductor's efficiency for applications. Key considerations include:
- Defect Density: Ensuring that defect levels are minimized is essential for reliable performance. High defect densities can lead to high leakage currents, impacting the overall functionality of devices.
- Homogeneity: Achieving uniform material properties across the wafer is vital. Non-uniformities may cause variability in device performance, making them less reliable in critical applications.
The importance of rigorous quality control cannot be overstated. Continuous advancements in analytical techniques and better monitoring systems could go a long way toward ensuring that produced materials meet the required specifications.
Integration with Existing Technologies
Successfully integrating ultra-wide band gap semiconductors with existing technologies is another considerable challenge. Many industries rely on established semiconductor technologies, primarily silicon-based devices. Moving to new materials requires careful planning, as these new semiconductors often have different properties that necessitate changes in device architecture and design methodologies.
- Compatibility: Ensuring that new semiconductors work seamlessly with existing systems is fundamental. Compatibility with established manufacturing processes and equipment can save time and costs.
- Standardization: Creating industry standards for new technology can help in faster adoption. Industries will be more willing to switch if they know what to expect regarding performance and reliability.
All these elements point to a pressing need for collaboration between researchers, manufacturers, and industries to devise strategies that facilitate smooth integration of ultra-wide band gap semiconductors in the current technological landscape.
The journey is filled with challenges but overcoming them is key to unlocking the full potential of ultra-wide band gap semiconductors in the modern world.
Future Directions in Research
As the field of ultra wide band gap semiconductors continues to evolve, pinpointing future directions in research becomes paramount. This section sheds light on the most promising avenues poised to redefine our understanding and application of these materials. From discovering new materials to enhancing fabrication methods, these initiatives aim to tackle existing challenges and leverage the unique advantages of ultra wide band gap semiconductors.
Innovative Material Discoveries
When it comes to ultra wide band gap semiconductors, innovation in material exploration is crucial. Researchers are investigating various compounds beyond the commonly known gallium nitride and silicon carbide. For example, materials like aluminum gallium nitride are generating buzz due to their exceptional properties, including high thermal conductivity and resistance to radiation.
Key benefits of innovative material discoveries include:
- Enhanced Performance: New semiconductor materials can potentially offer greater performance efficiencies at high temperatures.
- Increased Versatility: Discovering novel compounds may lead to the development of devices that function effectively across a wider range of applications.
- Cost Reduction: As new materials emerge, they may use cheaper production processes or abundant raw materials, reducing overall costs.
In summary, the ongoing quest for innovative materials promises to broaden the scope and applications of ultra wide band gap semiconductors, thereby attracting significant interest and investment from industry and academia alike.
Enhancements in Fabrication Methods
The way these materials are fabricated strongly influences their performance and reliability. As research pretains to fabricatioin techniques, improving existing methods or developing new ones has become a focus area. Techniques such as hydride vapor phase epitaxy and molecular beam epitaxy are under scrutiny for optimizing growth rates and enhancing crystal quality.
Considerations for enhancements in fabrication methods include:
- Scalability: It is vital that these methods can be scaled up without compromising quality, making it easier to meet industrial demand.
- Precision: Innovations must ensure precise control over doping and defects within the semiconductors, which can significantly impact their functionality.
- Sustainability: Finding ways to fabricate these materials that minimize waste and energy consumption is becoming increasingly important in today’s eco-conscious environment.
Through ongoing developments in fabrication techniques, the goal is to create ultra wide band gap semiconductors that not only perform better but are also environmentally friendly.
Applications in Emerging Technologies
With advancing research in ultra wide band gap semiconductors, a variety of applications in emerging technologies are becoming more viable. These materials are gradually shaping industries such as quantum computing, electric vehicles, and advanced telecommunications.
Some noteworthy applications include:
- Quantum Computing: The properties of ultra wide band gap semiconductors could enable faster and more efficient quantum bits, leading the way for breakthroughs in computing power.
- Electric Vehicles: The demand for high-efficiency power electronics in electric vehicles is soaring. Ultra wide band gap semiconductors are key to developing more efficient charging and driving systems.
- Telecommunications: The push for faster data transmission is requiring innovation in semiconductor technologies. Ultra wide band gap materials could enable new, high-frequency applications, improving wireless communication capabilities.
"Research into ultra wide band gap semiconductors isn’t just about performance; it’s about paving the way for revolutionary technologies that could shape our world for decades to come."
Culmination
The conclusion of this article encapsulates the essence of ultra wide band gap semiconductors, spotlighting their transformative potential in our technologically driven world. In discussing these materials, we underscore the significant breakthroughs they offer compared to traditional semiconductors.
Summary of Key Insights
Throughout the article, several pivotal insights have emerged regarding ultra wide band gap semiconductors, including the following:
- Enhanced Performance: These semiconductors demonstrate superior electrical and thermal properties, making them prime candidates for high-power applications.
- Wide Range of Applications: From power electronics to optoelectronics, their versatility is notable. Their role in solar cells is particularly impressive, where efficiency improvements can lead to substantial energy savings.
- Current Challenges: While the advantages are clear, challenges such as manufacturing costs and material quality control remain pressing. These issues can hinder widespread adoption and must be addressed.
- Future Directions: Continued research is vital to overcome present obstacles and unlock full potential. Innovative materials and improved fabrication techniques are on the horizon, promising an exciting future.
Final Thoughts on Future Impact
As industries pivot towards greener practices, these semiconductors will play a crucial role. With increased focus on renewable energy and efficient power conversion technologies, the demand for materials that can operate under extreme conditions will only surge.
In summarizing the discussions within this article, it’s clear that ultra wide band gap semiconductors are not just a niche market; they represent the cutting edge of modern technology. Their ability to bridge the gap between current needs and future demands will keep researchers engaged and industries evolving.
"In the world of semiconductors, innovation is the lifeblood, and ultra wide band gap materials are paving the way for a groundbreaking future."
As we look ahead, the horizon is bright, with tremendous possibilities on the, the application spectrum may extend beyond what we can currently envision. The further exploration of these materials will undoubtedly yield fascinating outcomes, aligning with both technological advancements and sustainability goals.