Exploring Type III Restriction Enzymes in Detail
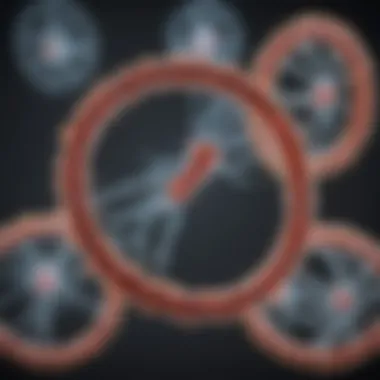
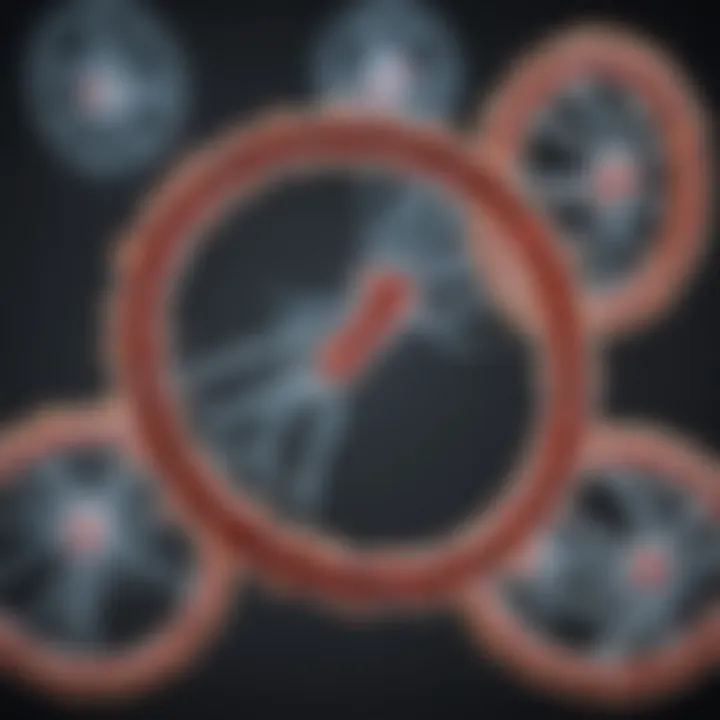
Intro
In the expansive realm of molecular biology, restriction enzymes have emerged as indispensable tools, and among them, Type III restriction enzymes deserve a closer look. These intriguing proteins offer a captivating insight into the intricate balance between biology and biotechnology. Their unique methods of recognizing and cleaving DNA set Type III enzymes apart from their counterparts, revealing layers of complexity that merit exploration.
The significance of Type III restriction enzymes extends beyond mere genetic manipulation. They play vital roles in natural cellular defense mechanisms, providing a novel vantage point from which researchers can investigate DNA interactions. As we delve into this article, we aim to dissect their biological importance, structural composition, and the functions that make them pivotal in scientific advancements.
Understanding these enzymes not only enriches our appreciation of molecular interactions but also enhances our ability to harness their power for genetic engineering applications. This article will serve as a thorough guide through the multifaceted landscape of Type III restriction enzymes, presenting essential knowledge for students, researchers, educators, and professionals alike.
Prelude to Restriction Enzymes
Restriction enzymes, also known as restriction endonucleases, are vital tools in molecular biology, and understanding them is crucial for anyone involved in genetic research or biotechnological applications. These enzymes have unique properties that allow them to recognize specific sequences of DNA and cleave them at defined points. This ability to cut DNA at precise locations makes them indispensable in the cloning and manipulation of genetic material.
In this section, we will shed light on the overall framework of restriction enzymes, emphasizing their contribution to genomic studies, the evolution of their roles in biotechnology, and the intricacies involved in their classification.
Overview of Restriction Enzymes
Restriction enzymes serve as the biological scissors that enable scientists to slice, rearrange, or even replicate DNA sequences. Each enzyme is attuned to fiercely guard the genetic information of an organism. That's right; they are nature's own defense against foreign DNA! With over 4,000 known types characterized across different species, these enzymes can be broadly categorized based on their structure, the nature of their cleavage, and their co-factors.
Some notable examples include:
- Type I Enzymes: These enzymes cut DNA at random distances from their recognition sites. They are complex and require ATP to function.
- Type II Enzymes: Perhaps the most commonly used in the lab, these enzymes cut at specific sites within or near their recognition sequences, making them highly predictable.
- Type III Enzymes: These target specific sites similar to Type II but require ATP and are less widely utilized, primarily due to the complex nature of their functionality.
With their precise cutting abilities, restriction enzymes greatly facilitate genetic engineering tasks, allowing for manipulation at the molecular level. Their utility spans a range of applications from gene cloning to the development of recombinant DNA technology.
Significance in Molecular Biology
In the landscape of molecular biology, restriction enzymes are not just convenient tools; they are transformative agents for scientific discovery. The ability to dissect DNA at will has led to numerous breakthroughs, the consequences of which resonate across the fields of genetics, virology, and biotechnology.
One key benefit these enzymes offer is in the area of gene cloning. Imagine you want to study a specific gene—restriction enzymes can help in isolating that gene, enabling thorough analysis of its function, expression patterns, or regulatory sequences. This process culminates in the creation of plasmids or vectors which can then be introduced into host cells for further study.
Moreover, these enzymes have a role in therapeutic applications. They pave the way for gene therapy by allowing for targeted insertion or modification of genes that may be responsible for diseases.
In summary, restriction enzymes are a cornerstone of molecular biology. Their precise methodologies for DNA manipulation echo through every nook and cranny of genetic research and biotechnological development, shaping the future of bioengineering and personalized medicine.
"Without the advent of restriction enzymes, our understanding of genetics would be a flicker amid the darkness of the unknown."
As we move forward through this exploration, grasping the details of type-specific restriction enzymes becomes paramount, particularly as we hone in on the Type III enzymes that possess distinct characteristics that differentiate them from their counterparts.
Understanding Type Restriction Enzymes
The realm of molecular biology is filled with exquisite mechanisms that govern the intricate dance of DNA manipulation. Among these, Type III restriction enzymes stand out due to their unique hallmark characteristics and roles in bacterial life. It’s crucial to grasp the essence of these enzymes to unlock their potential applications, especially in synthetic biology and genetic engineering.
Type III restriction enzymes play a pivotal role in the defense mechanisms of bacteria. They function not only as molecular scissors, slicing foreign DNA, but also as guardians that contribute to bacterial immunity. Understanding these enzymes empowers researchers to exploit their functions, thus advancing studies in gene editing and biotechnology.
Classification of Restriction Enzymes
To appreciate the distinct place of Type III enzymes in the grand scheme of restriction enzymes, one must first understand their classification. Restriction enzymes fall into three primary categories, each with unique properties and mechanisms:
- Type I: These are multifaceted enzymes that cut DNA far from their recognition sites. They function more like molecular machines, often requiring ATP to perform their tasks.
- Type II: The most commonly used in laboratories, Type II enzymes cut DNA at specific sequences, allowing for precise alterations. Their straightforward action has made them favorite tools in genetic engineering.
- Type III: Our focus here, Type III enzymes, recognize specific DNA sequences and cleave the DNA a short distance away from their recognition site. They are somewhat of a middle ground, operating in pairs and often relying on ATP but cutting a significant distance from the precise site.
Understanding these classifications lays the groundwork for delving into Type III restriction enzymes’ unique characteristics and signaling their importance in molecular biology.
Characteristics of Type Enzymes
The defining traits of Type III restriction enzymes set them apart. Here are some crucial characteristics that distinguish them:
- Recognition Mechanism: Unlike Type I or Type II enzymes, Type III enzymes recognize specific palindromic sequences. This specificity means they can target precise sites within a longer strand of DNA, which is advantageous in genetic manipulation.
- Cleavage Distance: Type III restriction enzymes cleave DNA at defined distances away from their recognition sites (often around 25-30 base pairs away), resulting in blunt ends rather than sticky ends, which can influence subsequent cloning strategies.
- Cooperativity: These enzymes usually function as complexes that require the presence of two or more identical units. This cooperativity mechanism means that, essentially, they work better together than alone, enabling a higher level of regulation and control in DNA manipulation.
"The unique properties of Type III restriction enzymes make them valuable tools in the understanding and manipulation of complex biological systems."
In essence, to grasp the significance of Type III restriction enzymes, one must appreciate their classification and inherent characteristics. Their multifaceted nature and cooperative mechanisms enable nuanced applications in biotechnology and molecular biology, proving that these enzymes are more than just tools; they are key players in the intricate web of life.
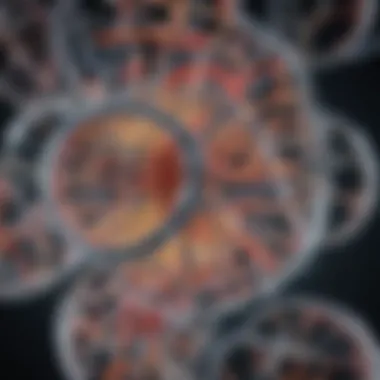
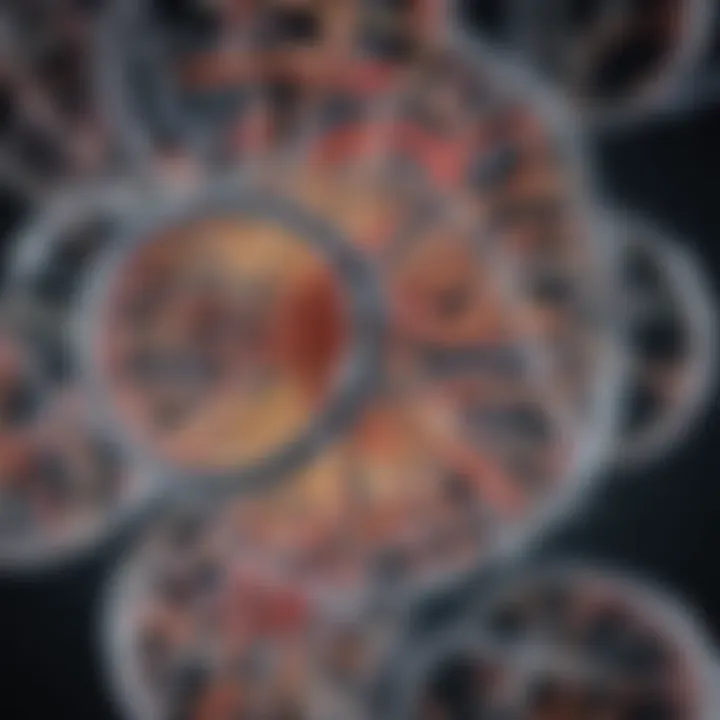
Mechanism of Action
Understanding the mechanism of action in Type III restriction enzymes is fundamental for comprehending how these enzymes function within molecular biology. Their unique mechanics determine how they recognize and cleave DNA, playing a vital role in gene regulation and editing. This section breaks down the various elements associated with this process, demonstrating its significance and implications.
DNA Recognition Process
The DNA recognition process is where the magic begins. Type III restriction enzymes are known to have a distinctive mode of DNA recognition, different from their counterparts like Type I and Type II enzymes. Rather than recognizing specific palindromic sequences like Type II enzymes, Type III restriction enzymes look for extended motifs that are not necessarily repeated. This broader range of recognition allows them to function effectively in diverse genetic environments, offering flexibility in their applications.
In specific, Type III enzymes typically bind to their target DNA sequences with their two-component systems, which involve a restriction subunit and a modification subunit. The restriction subunit is responsible for DNA cleavage, while the modification subunit ensures that the host DNA remains protected from cleavage. This dual-action approach is akin to a double-edged sword; it protects the organism's own DNA while being ready to attack foreign sequences.
"The choice of recognition strategy gives Type III enzymes a unique edge in the vast world of genetic material, adapting to various environments and challenges."
Cleavage Mechanism
Once the DNA is recognized, the next step is the cleavage mechanism. Here, Type III restriction enzymes employ a complex series of biochemical reactions to cut the DNA strands. Unlike Type II enzymes that make clean cuts, Type III restriction enzymes typically create staggered cuts. This means they cut one strand before the other, leaving behind sticky ends that can easily bond with other DNA molecules. This property is particularly advantageous in recombinant DNA technology, where such sticky ends can facilitate the insertion of foreign DNA sequences into plasmids or other vectors.
The cleavage does not happen at the site of recognition directly. Instead, Type III enzymes tend to require ATP hydrolysis as part of the cleavage mechanism. This dependency on ATP reserves underscores the energy-intensive process that defines their action, making them particularly responsive to the availability of cellular energy stores.
Comparison with Other Enzymes
When comparing Type III restriction enzymes to other enzyme types, several key distinctions emerge.
- Mechanism of Cleavage: As previously noted, Type III enzymes employ staggered cuts, contrasting sharply with Type II, which makes precise cuts at specific sites.
- Recognition Sequences: Type II restriction enzymes are known for their high specificity, while Type III enzymes maintain flexibility, interacting with longer motifs.
- Dependency on ATP: Notably, enzymes in Type III require energy in the form of ATP for processing, unlike Type II enzymes that do not.
This comparative analysis highlights why Type III enzymes might be considered more adaptable, though perhaps less predictable in experimental settings. Understanding these differences not only clarifies their individual roles but also emphasizes the importance of selecting the appropriate enzyme based on experimental requirements.
In summary, the mechanism of action for Type III restriction enzymes combines a sophisticated recognition system with a unique cleavage mechanism that sets them apart. By exploring these features, we gain a deeper appreciation of their place in biotechnology, molecular cloning, and genetic engineering.
Biological Roles
The biological roles of Type III restriction enzymes are multifaceted and crucial to understanding their significance in microbial ecology and biotechnology. Unlike their type I and II counterparts, Type III enzymes occupy a unique position within the restriction-modification systems that protect bacteria from foreign DNA. Probing into their functions reveals not just their participation in bacterial defense, but also hints at their broader implications in genetic diversity and gene transfer.
Role in Bacterial Defense
Type III restriction enzymes are akin to a knight in shining armor for bacteria. These enzymes function primarily as a defense mechanism against invasive genetic elements such as phages and plasmids. When foreign DNA enters the bacterial cell, it can wreak havoc, potentially leading to cell death or dysfunction. Type III enzymes counter this threat through a fascinating mechanism of action.
Upon recognizing the foreign DNA, Type III enzymes undergo a process that initiates the cleavage of this unwanted genetic material. They do this in a sequence-dependent manner, which is particularly interesting; they demand a specific sequence in the DNA to bind effectively. The ability to selectively target certain sequences means that they have a refined "sixth sense" to identify self versus non-self DNA. This selective pressure helps bacteria maintain their genetic integrity and contributes to their survival.
Moreover, their action doesn’t solely involve digesting foreign DNA; it acts as a warning signal. During the confrontation, they can also regulate other cellular pathways, alerting the bacterium to potential threats. This interconnectedness enhances our understanding of evolutionary advantages associated with having such enzymes.
Implications for Horizontal Gene Transfer
Horizontal gene transfer (HGT) describes the transfer of genetic material between organisms in a manner other than traditional inheritance. This mechanism plays a pivotal role in microbial evolution, and Type III restriction enzymes are intricately tied to this process.
On one hand, these enzymes can limit HGT by targeting incoming plasmids or phage DNA that may carry genes harmful or unnecessary to the host organism. By doing so, they protect against the potential spread of unwanted genetic traits, like antibiotic resistance, which can destabilize ecosystems and pose a significant public health risk.
On the flip side, the landscape becomes more complex when considering that bacterial species often share genes across various populations. Type III enzymes, while protective, may inadvertently facilitate gene sharing by creating a selective environment in which only certain DNA can persist.
The dance between defensiveness and adaptability illustrates the dual role these enzymes play in shaping bacterial populations. They serve as gatekeepers, monitoring what enters and leaves a cell, thus playing a pivotal role in the evolutionary battleground of genetic exchange.
"The biological significance of Type III restriction enzymes extends beyond simple defense; they are essential for maintaining the delicate balance of genetics within and across microbial communities."
In summary, the biological roles of Type III restriction enzymes illuminate their importance as a defense mechanism against foreign DNA while also showcasing their influence on horizontal gene transfer. This duality not only provides an evolutionary edge to bacteria but also raises pressing questions about the implications for gene transfer in a world faced with increasing antibiotic resistance and genetic engineering challenges. Understanding these roles is crucial for students, researchers, and professionals who wish to harness the potential of these enzymes in biotechnological applications.
Structural Properties
Understanding the structural properties of Type III restriction enzymes is crucial to grasping how these proteins operate at a molecular level. By delving into their unique configurations and compositions, we obtain insights not only into their specific functions but also into their broader implications in molecular biology and biotechnology. This exploration includes considerations of protein folding, complex formation, and the dynamics of enzyme-substrate interactions, which are all fundamental to the effective application of these enzymes in various research contexts.
Protein Structure and Composition
The protein structure of Type III restriction enzymes is characterized by multiple domains that play specific roles in functionality. These enzymes often exhibit a multidomain architecture, integrating elements that allow them to recognize target DNA sequences and facilitate subsequent enzymatic reactions. Essentially, the structure is formed in such a way that it can recognize a specific DNA sequence and then engage in cleavage, all of which hinges on the precise arrangement of amino acids within the polypeptide chain.
Key aspects of their composition include:
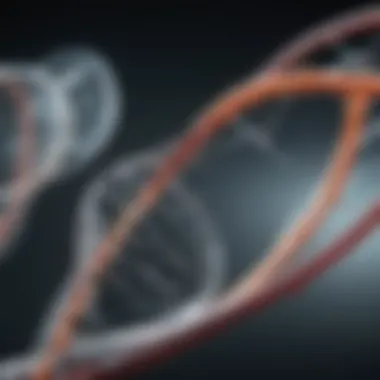
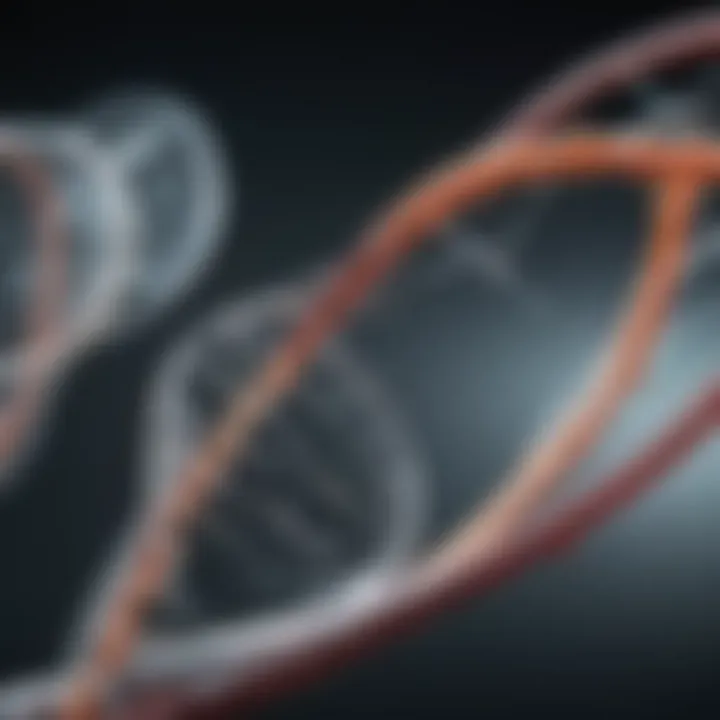
- Active Site Configuration: The arrangement of residues within the active site is critical. This site is tailored for recognizing the specific DNA sequence and catalyzing the cutting action
- Regulatory Domains: These areas integrated into the structure influence enzyme activity and are essential when responding to cellular signals.
- Oligomerization: Many Type III enzymes function as multimers, meaning they form complexes made up of several subunits. This allows for intricate regulation and enhancement of specificity in their actions.
The precise structure can significantly influence the enzyme's effectiveness in various applications such as genetic engineering and synthetic biology. The three-dimensional configuration must be understood to manipulate these enzymes effectively, whether for gene editing, cloning, or therapeutic purposes.
Enzyme Stability and Functionality
Stability is a pivotal factor that impacts the functionality of Type III restriction enzymes. Factors such as temperature, pH, and ionic strength can dramatically affect how these enzymes operate. Their stability directly correlates with their ability to maintain structure in even challenging conditions, which is vital for laboratory applications. If an enzyme becomes unstable, its capacity to cut DNA at intended sites diminishes, leading to undesired consequences in research or therapeutic outcomes.
Several factors contributing to enzyme stability include:
- Protein Folding: Proper folding is critical for creating a functional active site.
- Post-Translational Modifications: These can either stabilize the enzyme or modulate its activity.
- Environmental Conditions: Changes in temperature or pH can lead to denaturation or activation of different forms of the enzyme.
A robust understanding of these elements of structure and stability can inform researchers in optimizing experimental conditions to ensure maximum efficiency and effectiveness of Type III enzymes in various applications.
"The functionality of enzymes is not just about their active sites; it is about the relationship between structure and stability that determines their usability in a lab setting."
Ultimately, the intersection of structural properties with enzyme stability underscores the need for ongoing research in understanding Type III restriction enzymes. Their intricate designs are as awe-inspiring as they are essential for advancements in biotechnology.
Experimental Approaches
Understanding Type III restriction enzymes necessitates a look into the experimental approaches used to study these intricate proteins. Whether for academic inquiry or practical applications, knowing how to accurately isolate and assess the activity of these enzymes is essential. This section provides an overview of the methods researchers employ to unlock the secrets of Type III restriction enzymes and the significance of these methods in research and biotechnology.
Methods for Enzyme Isolation
The isolation of Type III restriction enzymes involves several strategic techniques aimed at purifying the enzyme from the microbial sources where it resides. Approaches often include:
- Cell Lysis: The first hurdle in isolation is breaking open the cells that secrete the enzyme. Various lysis methods, such as sonication or enzymatic lysis, can be employed depending on the target organism's cell wall composition.
- Precipitation: Following lysis, researchers utilize selective precipitation methods, like ammonium sulfate precipitation, to enrich the enzyme from the cell lysate. This step often relies on the enzyme’s solubility characteristics, allowing it to separate from other proteins.
- Chromatography: A staple in protein purification, chromatography methods—such as ion exchange, size exclusion, or affinity chromatography—help achieve a higher degree of purity. For example, using a column with specific binders can target the enzyme while leaving other impurities behind.
These methods, while essential, come with their own sets of challenges. Factors such as the enzyme’s stability, molecular size, and endogenous inhibitors can hinder isolation efforts. Hence, optimizing conditions during each step is critical.
Techniques for Activity Assessment
Once isolated, the next step is to assess the activity of Type III restriction enzymes. Various techniques can be employed, which helps researchers confirm functionality and understand the enzyme's dynamics. Here are some common methods used:
- Agarose Gel Electrophoresis: This classic method allows for visualization of DNA cleavage. By running a sample of DNA on a gel before and after incubation with the enzyme, researchers can compare the results. An increase in band intensity or a change in band pattern provides a clear indication of enzyme activity.
- Fluorescent Assays: Emerging technologies include using fluorescent tags on DNA substrates. The release of fluorescence upon cleavage can be quantified, providing a sensitive measurement of activity over time.
- Kinetic Studies: Researchers may also engage in kinetic assays, calculating parameters such as Km and Vmax to evaluate the efficiency and affinity of the enzyme for its DNA substrates. This data helps illuminate the enzyme's characteristics under various substrate concentrations.
"Accurate assessment of enzyme activity is just as crucial as isolation; it provides the insight needed to exploit the enzyme in biotechnological applications."
In summary, the experimental approaches for isolating and assessing the activity of Type III restriction enzymes are foundational to understanding their role in molecular biology. These methods not only shed light on the enzymes themselves but also influence their applications in gene cloning and editing, showcasing their significance in modern genetic research.
Applications in Biotechnology
The realms of biotechnology are wide and varied, but Type III restriction enzymes offer a particularly fascinating suite of tools for genetic manipulation. Their specificities and unique mechanisms for DNA cleavage allow them to play innovative roles across numerous biotechnological applications. Understanding their function is not just of academic interest; it has real-world implications that drive advancements in genetics, medicine, and agriculture.
Gene Cloning Techniques
Gene cloning, the process of isolating and making copies of specific genes, greatly benefits from Type III restriction enzymes. By utilizing these enzymes, researchers can effectively manipulate DNA sequences in a targeted fashion. For instance, the capacity of Type III enzymes to recognize sequences that are only a short distance apart allows for sophisticated cloning strategies. Their activity in cutting DNA at particular sites provides a reliable method for inserting genes into plasmids or other vectors.
One such method employed involves the use of specific Type III restriction sites to ligate genes of interest. This ligation process leads to creation of recombinant DNA, which is crucial in the production of proteins, study of gene functions, and development of genetically modified organisms. Another application of these enzymes is in creating DNA libraries, where diverse genetic material is stored for future analysis or manipulation.
- Benefits of gene cloning with Type III enzymes:
- Precision in targeting specific DNA sequences
- Reduces the risk of unintended cleavages elsewhere in the genome
- Facilitates high-throughput cloning methods
Researchers also observe that these enzymes can be employed in restriction cloning procedures where they can help generate fragments for subcloning, ensuring that the desired sequences are isolated and amplified, paving the way for various downstream applications.
Gene Editing Innovations
With the rise of gene editing technologies like CRISPR, Type III restriction enzymes have gained newfound attention. Their capability to induce double-stranded breaks at specific locations can be harnessed to create precise gene edits. This method allows for modifications that can correct genetic disorders, enhance crop resilience, or even produce animal models for studying human diseases.
Moreover, Type III enzymes can work synergistically with other techniques, such as homologous recombination. When a break is introduced in conjunction with a donor template, precise edits can be incorporated into the genome, thus enabling researchers to tailor genetic sequences to their preferences.
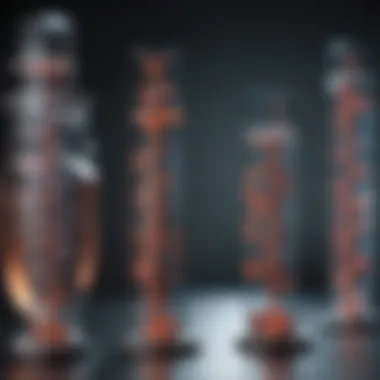
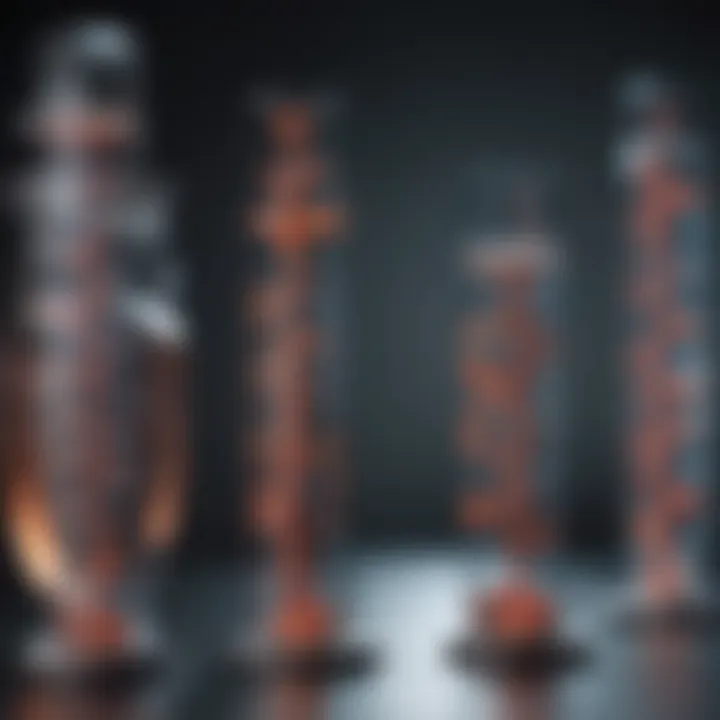
"The potential for Type III restriction enzymes to evolve with gene editing technologies opens new doors in molecular biology research."
Envisioning the future, Type III enzymes could serve as essential components in biosensors that will allow for improved monitoring of gene expression, ultimately improving agricultural outputs or designing smarter therapeutic approaches.
Both the applications in gene cloning and gene editing demonstrate how Type III restriction enzymes are not just a relic of molecular biology, but rather active players in shaping the future landscape of biotechnology.
Challenges and Limitations
Understanding the challenges and limitations surrounding Type III restriction enzymes is crucial for both fundamental and applied research. As we dive into the world of these remarkable enzymes, it becomes evident that while they offer significant benefits in genetic engineering and biotechnology, navigating the potential pitfalls is equally important. Acknowledging these hurdles can lead to improved methodologies and innovative solutions in scientific experiments.
Enzyme Specificity Issues
One of the most pressing challenges is the specificity of Type III restriction enzymes. Unlike their Type II counterparts, which exhibit a more predictable cutting pattern, Type III enzymes can be quite finicky in their target recognition.
- Cutting not always at established sites: These enzymes typically recognize specific sequences but may not cleave DNA solely at those sites. This characteristic can confound researchers aiming to refine precise gene editing techniques.
- Variability among strains: The same enzyme might not behave identically when sourced from different strains of bacteria, leading to inconsistency in experimental results.
This variability fundamentally challenges the reproducibility that is the cornerstone of scientific research. While it is expected that certain conditions such as temperature or pH might influence enzyme activity, the inherent specificity of Type III enzymes often demands meticulous optimization for each desired application. Researchers must spend meaningful time validating their enzyme choices and operational conditions to mitigate these specificity issues.
Potential for Off-Target Effects
Another pressing concern involves off-target effects, where the enzyme inadvertently cleaves unintended sites. This unintended action can have significant ramifications in gene editing applications where precision is paramount.
"The collateral damage from off-target cleavages can lead to undesired genetic modifications that may complicate downstream analyses and applications."
- Consequences of off-target cuts: Off-target effects might not only disrupt existing genes but could also activate or silence other genes unexpectedly. Thus, the original goals of a genetic engineering project could be derailed.
- Development of assays for detection: Researchers increasingly need to rely on advanced sequencing techniques to ascertain the fidelity of their gene-editing efforts. This necessitates additional resources and expertise, thus adding layers of complexity to projects.
Future Directions in Research
The field of restriction enzymes, particularly Type III, stands at an interesting crossroads. The continual evolution of biotechnology underscores the need for innovation and adaptability. This section highlights the importance of future research directions, focusing on the benefits their insights could provide, along with specific considerations needed for deeper exploration.
Innovative Commercial Applications
Type III restriction enzymes boast distinctive characteristics that potentially expand their utility in commercial settings. Unlike their counterparts, these enzymes have unique cleavage patterns and require a specific recognition sequence, offering an edge in precision. Current market trends illustrate how biotechnology is transforming industries, and Type III enzymes can pave the way for novel applications. Some areas of interest include:
- Therapeutic Development: With their ability to edit genes accurately, these enzymes can aid in creating tailored therapies for genetic disorders. This ability to precisely target DNA could revolutionize medicine.
- Agricultural Biotechnology: Agricultural advancements require tools that can modify crops efficiently. Type III enzymes can facilitate the development of pest-resistant varieties, thereby ensuring food security and sustainability.
- Biomanufacturing: Companies are leveraging biotechnological processes to produce novel materials. With the adaptability that Type III restriction enzymes present, future commercial applications may lead to more sustainable practices.
Emerging research that focuses on optimizing enzyme activity and specificity in specific commercial contexts is critical for realizing these potential applications. As these enzymes gain traction, understanding their market dynamics will inform researchers about existing gaps and opportunities.
Emerging Technologies in Gene Regulation
The combination of Type III restriction enzymes with emerging technological advances presents a promising frontier in gene regulation. As the need for more sophisticated tools to understand and manipulate genetic information grows, the utility of these enzymes steps into the spotlight. Here are several noteworthy points regarding their interplay:
- CRISPR-Cas Systems: The recent advancements in CRISPR technology highlight gene editing's potential. Integrating Type III restriction enzymes within CRISPR systems could enhance specificity and reduce off-target effects, making gene modification safer and more reliable.
- Synthetic Biology: The principles of synthetic biology aim to redesign organisms for useful purposes. Implementing Type III enzymes may lead to innovative pathways for biosynthesis and metabolic engineering, allowing for new avenues in creating biofuels or pharmaceuticals.
- High-Throughput Screening: The quest for better methods to study genes can be enhanced by employing Type III enzymes in high-throughput platforms. This could accelerate the process of identifying regulatory elements, which would be invaluable for biological research and therapeutic discovery.
"The confluence of Type III restriction enzymes and emerging technologies heralds a new era in the realms of gene regulation and biotechnology, offering potentially transformative solutions to agricultural and medicinal challenges."
As researchers delve deeper into the nuances of these enzymes, the scientific community will be better equipped to harness their potential in the quest for more effective, cutting-edge solutions to global challenges.
Epilogue
In this final segment, we reflect on the wide-ranging implications and significance of Type III restriction enzymes within the context of molecular biology and biotechnology. These enzymes are not just tools in the lab; they play pivotal roles in understanding genetic mechanisms and manipulating them for various applications. This conclusion captures the essence of the discussions throughout this article.
Summary of Key Insights
To summarize, Type III restriction enzymes exhibit unique characteristics that distinguish them from their counterparts. They act on specific DNA sequences, typically recognizing two non-palindromic sites and requiring ATP for their action. This non-traditional method of DNA cleavage has made them a fascinating subject for researchers.
Key insights include:
- Classification: Type III enzymes are classified based on their recognition and cleavage patterns. This places them in a distinct category vital for various experimental designs.
- Biological Role: Their role in bacterial defense mechanisms underscores their evolutionary importance, shedding light on how bacteria evolve in response to phage threats.
- Applications: The potential applications in biotechnology are vast. From gene editing to cloning, these enzymes stand out as critical resources that improve genetic manipulation techniques.
- Future Research: The challenges posed by issues like enzyme specificity and potential off-target effects are worth noting. Research must continue to refine the application of Type III enzymes for safer and more efficient methods in genetic engineering.
In essence, the understanding of Type III restriction enzymes not only enhances our grasp on molecular genetics but also propels forward innovations in biotechnology.
Implications for Future Study
The ongoing study of Type III restriction enzymes holds significant promise for advancing genetics, molecular biology, and biotechnology. A few implications for future exploration include:
- Innovations in Genetic Engineering: As new technologies and methods emerge, the role of Type III enzymes can evolve. They could be pivotal in establishing more sophisticated tools for gene editing, particularly in areas like CRISPR technology.
- Therapeutic Applications: There's a burgeoning interest in utilizing these enzymes for therapeutic purposes, especially in treating genetic disorders. Investigating how to minimize off-target effects could lead to groundbreaking treatments.
- Expanded Characterization: Understanding the complete protein structures and functions of these enzymes may reveal new insights into their capabilities and limitations, opening doors for novel applications.
The future of Type III restriction enzymes looks promising, and a deeper dive into their mechanisms and interactions can yield exciting discoveries that might change the landscape of molecular biology.