Exploring Scanning Electron Microscopes: Functions and Impact
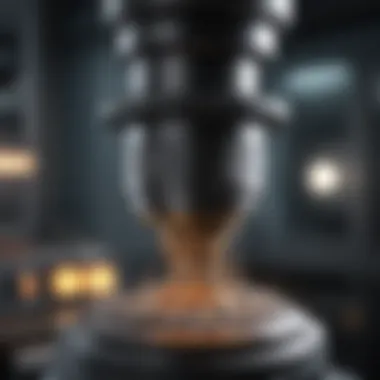
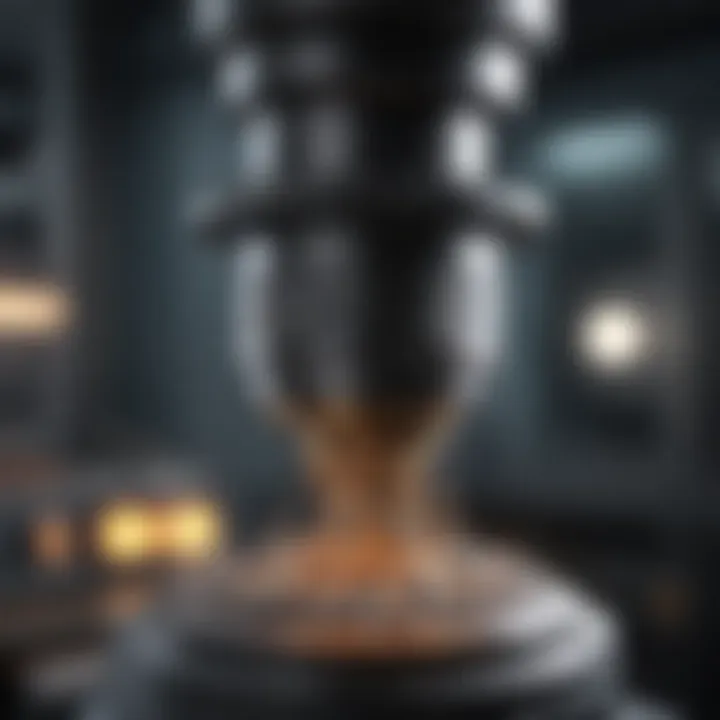
Intro
Scanning electron microscopes (SEMs) are marvels of modern technology that throw open the doors to the microscopic world, allowing us to see materials in extraordinary detail. Unlike traditional optical microscopes that rely on light, SEMs utilize a focused beam of electrons to illuminate specimens. This method provides images that are not only higher in resolution but also reveals surface structures and textures, often down to the nanometer scale.
As we explore the functionalities of these instruments, it becomes clear how integral they have become in various fields. From materials science to biology, their impact is profound, shaping the way we perceive materials and biological structures. Numerous advancements in SEM technology continue to enhance our ability to investigate the micro and nanoscopic realms, pushing boundaries that were previously thought unreachable.
This article will navigate through the operational principles of SEMs, their applications across diverse disciplines, and the significant contributions they make to scientific inquiry and innovation. By shedding light on their technological underpinnings and limitations, we aim to enhance your understanding of SEMs and their role in contemporary scientific exploration.
Foreword to Scanning Electron Microscopes
Scanning Electron Microscopes, or SEMs, have revolutionized the field of microscopy, allowing us to peer into the microscopic world with unprecedented clarity. In today's scientific landscape, their role is not merely ancillary but fundamental. The increasing demand for precision imaging in various sectors, from materials science to biology, underscores the importance of understanding how SEMs function and the impact they have on research and technology.
At the heart of SEM technology lies its ability to produce high-resolution, three-dimensional images that reveal intricate details of samples, making it an invaluable tool for professionals across disciplines. Given the breadth of applications—ranging from examining the surface structures of metals and polymers to investigating biological tissues—this introduction lays the groundwork for a deeper exploration of SEMs in the subsequent sections.
Definition and Overview
A Scanning Electron Microscope is an advanced imaging tool that employs focused beams of electrons to scan the surface of a sample. Contrary to traditional light microscopes that utilize visible light, SEMs offer much greater resolution and depth of field, enabling researchers to visualize structures at the nanometer scale. The images generated by SEMs are not only informative but also the preferred method for characterizing materials due to the vast amount of data they can provide. The electron interaction mechanism with the sample surface leads to various signals that arise, such as secondary electrons, backscattered electrons, and x-rays, which contribute to the formation of detailed images and compositional analysis.
Historical Context
The journey of scanning electron microscopy began in the late 1930s, with the efforts of scientists like Ernst Ruska and Max Knoll, who developed the first prototype of the electron microscope. Initially, the concept was rudimentary, focusing on using electrons to gain higher resolution images than those obtained with light. However, it wasn't until the 1960s that Raymond C. McIntyre and others propelled the technology into practical applications. During this time, the introduction of the first commercially available SEMs made a significant impact, allowing researchers to explore nanoscale surfaces more effectively.
Over the decades, SEM technology has undergone numerous upgrades and enhancements, such as the introduction of scanning modes and advanced detectors, allowing for a broader range of applications and increasing the accessibility of this technology in various fields. This evolution reflects the continuous quest for better imaging techniques and the role of SEMs as a staple in modern scientific inquiry. The context of SEM's development is crucial as it highlights not only the technological advancements but also the increasing recognition of its significance in fields ranging from semiconductor research to materials engineering.
Principles of Operation
Understanding the principles of operation is crucial to appreciate how scanning electron microscopes (SEMs) work. Each element within an SEM plays a significant role in its functionality, from generating the electron beam to how images are formed. Through a detailed exploration of these principles, we can see not only how SEMs capture high-resolution images but also the benefits they offer in diverse scientific fields.
Electron Generation
At the heart of a scanning electron microscope is the electron gun, which performs the vital task of producing electrons. Typically, the gun employs a heated tungsten filament or a field emission source to emit electrons. When the filament heats up, it releases electrons through thermionic emission. In the case of field emission, a strong electric field pulls electrons from a sharp tip.
Once emitted, the electrons are accelerated through a high-voltage electric field to gain significant energy before they travel toward the sample. This process is not just about getting electrons flying; the energy and focus of this beam are essential for analyzing different materials. For instance, higher energy beams can penetrate denser materials, allowing for varied applications like metallurgy or nanotechnology. Understanding this initial step is key to grasping how resolution and depth of field are achieved in the final images.
Interaction with Sample
As the focused electron beam hits the sample surface, a series of interactions takes place that are crucial for image formation. During this stage, electrons collide with the sample's atoms, causing a number of phenomena, namely:
- Secondary Electron Emission: Some of the electrons knock out secondary electrons from the sample. These secondary electrons are what typically create the image viewed on the screen.
- Backscattered Electrons: A portion of the incident electrons bounce back off the sample. Their energies can provide information about the composition and atomic number of the materials being analyzed.
- X-ray Emission: In some instances, interactions might lead to the emission of X-rays. These X-rays can be analyzed further to reveal the elemental composition of the sample.
These interactions highlight the capacity of SEMs to provide detailed insights into a sample’s surface morphology, allowing researchers to examine the physical structure precisely. The essence of this interaction phase underscores how diverse materials can be characterized under different environmental conditions; for example, studying biological samples versus metals can lead to vastly different outcomes.
Image Formation Techniques
The formation of images in SEM is a meticulous process that hinges on the efficient collection of the electrons emitted upon interaction with the sample. Once the secondary and backscattered electrons are generated, they are funneled towards detectors.
There are multiple techniques for reading the signals:
- Everhart-Thornley Detector: This device is often used to capture secondary electrons, helping create high-resolution images of the surface topography of samples.
- Backscattered Electron Detector: This one picks up backscattered electrons, assisting in examining the composition and density variations within a material.
- Energy Dispersive Spectroscopy (EDS): A technique often coupled with SEM to analyze the X-rays and derive elemental information, further enhancing the understanding of the sample composition.
"The various image formation techniques not only display intricate surface details but also reveal compositional info, bridging the gap between qualitative and quantitative analysis."
Together, these methods deliver images that enhance material characterization and ensure rich data collection for diverse disciplines. It’s evident that the principles of operation in scanning electron microscopy serve as a foundational pillar upon which this powerful imaging tool stands, further illustrating its pivotal role in modern scientific research.
Components of a Scanning Electron Microscope
The components of a scanning electron microscope (SEM) are crucial to its overall functionality. Each part works in concert to allow scientists to visualize samples at a microscopic level with impressive detail. It's not just about having the right tools; it's about how they interact to produce stunning results in various fields of study, from materials science to biology. Understanding these components helps appreciate the intricate functionality of the SEM.
Electron Gun
The heart of an SEM is the electron gun. This device generates the electron beam that scans across the sample surface. Typically, the gun uses sources like tungsten filaments or field emission guns, each possessing distinct characteristics. The tungsten filament is more common because of its reliability and cost-effectiveness.
"The electron gun's design and performance directly impact the quality of the images produced by the microscope."
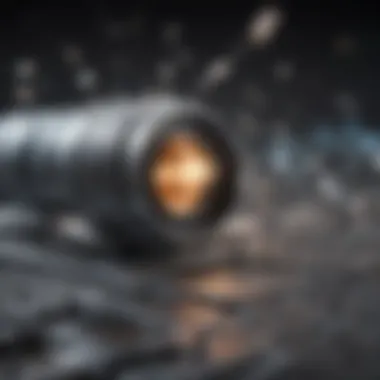
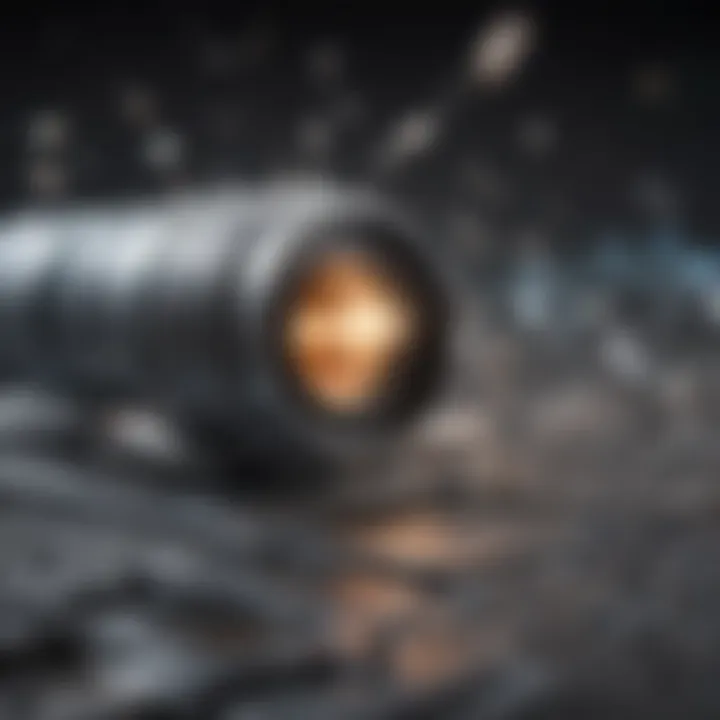
Field emission guns, although pricier, provide a higher brightness and a smaller source size, allowing for better resolution in imaging. The electrons are accelerated and focused into a fine beam, which is vital. This quality beam penetrates the surface of samples, enabling detailed imaging.
Lenses and Scanning Mechanism
After the electron beam is generated, it passes through a series of lenses. These lenses focus the beam onto the sample. Scanning lenses are especially designed to shift the beam across the surface, creating a raster pattern. This meticulous scanning allows for comprehensive coverage of the sample.
Key note: The resolution and depth of focus of the images are heavily influenced by these lenses. A high-quality lens setup makes all the difference in visual outcomes.
Another vital part of the SEM is its scanning mechanism. It moves the electron beam precisely over the sample while synchronizing with data acquisition systems. This coordination ensures that the images captured are both high-resolution and faithful to the actual structure of the sample being examined.
Detectors
Once the electron beam interacts with the sample, various detectors come into play. The main types include secondary electron detectors and backscattered electron detectors, each serving its own purpose in image formation.
- Secondary Electron Detectors (SED): These detect electrons that are ejected from the sample surface, providing detailed topographical images. They are particularly useful for observing surface features at high resolutions.
- Backscattered Electron Detectors (BSED): These capture electrons that bounce back from the sample, giving insights into the composition and atomic number variations across the surface.
The data collected from these detectors is crucial for creating the final images and analyses. A well-designed SEM will have sophisticated detection systems in place that are sensitive enough to capture even subtle variations in electron emissions.
Applications of Scanning Electron Microscopes
Scanning Electron Microscopes (SEMs) play a pivotal role in various scientific disciplines, enabling researchers to gain high-resolution images of sample surfaces. These images not only aid in visual analysis but also provide critical insight into the material composition and structure. Understanding the applications of SEMs not only informs practitioners about the breadth of their usability but also highlights their relevance in propelling innovation and discovery.
Materials Science
In materials science, SEMs serve as indispensable tools in studying the physical and chemical properties of materials. Researchers utilize SEM to observe fracture surfaces of metals, polymers, and ceramics, which sheds light on how these materials fail and how they can be improved. For instance, by examining a broken metal sample under SEM, one can discern the microstructural defects that contributed to its failure.
This application extends to the development of new materials as well, where SEM helps in evaluating the interactions at the atomic level. Through techniques like Energy Dispersive X-ray Spectroscopy (EDS), scientists can analyze elemental composition and distribution which is critical for advancing materials engineering.
Biology and Medicine
In the realms of biology and medicine, SEMs are revolutionizing the way we understand life at a microscopic level. From examining the fine details of cell structures to investigating pathogens, SEMs provide crucial insights into biological processes. For example, with electron microscopy, researchers can delve into the surface topography of cells, leading to a better grasp of cellular mechanisms.
Additionally, SEMs have vital applications in the fields of histopathology. By examining tissue samples, pathologists can identify abnormal cells and diagnose diseases, enhancing the accuracy of medical assessments. This contributes directly to patient care, optimizing treatment strategies and outcomes.
Nanotechnology
Nanotechnology is another arena where SEMs excel. As scientists work with materials at the nanoscale, understanding their properties becomes increasingly intricate. SEMs offer the capability to visualize nanostructures in unprecedented detail, facilitating the exploration of phenomena unique to nanoscale materials.
This imaging enables innovations in drug delivery systems, where nanoparticles can be designed to target specific cells within the body. Similarly, the observation of nano-coatings on surfaces — whether they be anti-corrosive or hydrophobic — can lead to advancements in industrial applications. The real-time imaging abilities of SEM can help in monitoring processes that occur at this tiny scale, providing insights that are invaluable in research and development.
Electronics and Semiconductors
In the fast-evolving world of electronics and semiconductors, SEM plays a critical role in the design and fabrication of devices. The accurate measurement of semiconductor patterns and features is paramount in ensuring the functionality of electronic components like transistors and integrated circuits. SEMs can evaluate the surface defects, layer thicknesses, and other nuances of semiconductor materials.
Moreover, as electronic devices continue to shrink in size, the precision required in manufacturing becomes more stringent. SEM allows engineers to surface inspect and analyze the nanoscale aspects of chip manufacturing, identifying defects that could lead to failures in performance. Thus, it propels the ongoing quest for higher efficiency and newer technologies in electronics.
"The capability of SEM to capture minute details transforms fields such as materials science and biology, allowing researchers to unveil insights that guide the development of next-generation technologies."
In summary, the applications of Scanning Electron Microscopes in materials science, biology, nanotechnology, and electronics underline their significance in diverse research areas. Each application not only enhances our understanding but also drives impactful innovations that resonate across industries.
Advantages of Scanning Electron Microscopes
Scanning Electron Microscopes (SEMs) stand as pillars in modern scientific investigation. Their contributions to various fields are invaluable, primarily due to their distinct advantages that other microscopy techniques may not offer. By comprehensively understanding these advantages, one can better appreciate the vital role SEMs play in advancing research and innovation across disciplines such as materials science, biology, and nanotechnology. The following sections delve into each noteworthy advantage, offering insight into why SEMs have gained significant traction in the scientific community.
High Resolution
One of the most compelling benefits of a Scanning Electron Microscope is its exceptional resolution capabilities. SEMs enable researchers to view samples at a granular level, often achieving resolutions of 1 nanometer or better. This level of detail is crucial when examining the surface morphology and nanostructures of materials. For instance, in semiconductor research, identifying defects at the nanometric scale can be a game changer. The deeper understanding of material configurations assists engineers in refining processes and enhancing product reliability.
With SEMs, scientists can analyze not just the texture but also the composition of a surface, allowing for precise material characterization. A high-resolution image can reveal intricate details that would otherwise go unnoticed. This leads to new discoveries that can influence product design, performance, and even lifespan. The clarity provided by SEMs can be likened to getting a high-definition view of a world that is otherwise shrouded in fog.
Three-Dimensional Imaging
Another remarkable aspect of SEM technology is its ability to create three-dimensional images of samples. While traditional microscopy typically yields flat, two-dimensional representations, SEM has revolutionized this perspective. Utilizing techniques such as stereoscopy and digital image processing, SEM can construct a stereoscopic representation of the sample's surface contours.
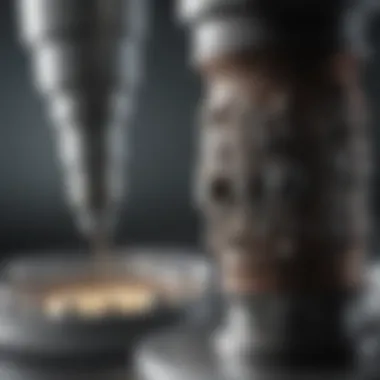
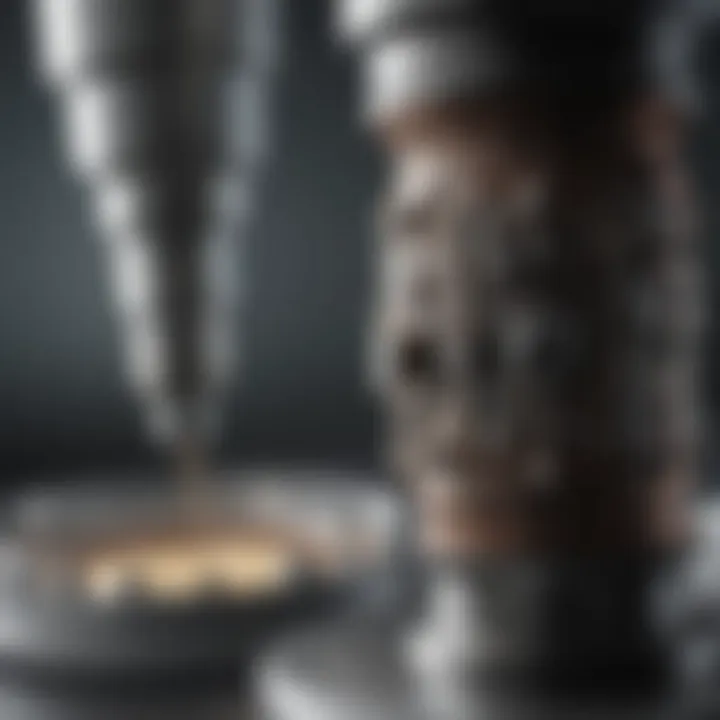
This is particularly important in biological studies, where the spatial arrangement of cells and tissues must be understood for accurate analysis. For example, examining the complex architecture of a cell surface can provide insights into cellular interactions that play crucial roles in health and disease. The 3D interpretation allows for more comprehensive data collection, enabling researchers to visualize not only individual components but also how they integrate within a system.
Moreover, the ability to visualize structures in three dimensions facilitates better communication of findings, making this data more digestible for researchers and stakeholders alike.
Versatility in Material Analysis
SEMs are often heralded for their versatility in analyzing an array of materials, be it metals, polymers, or biological specimens. They are adept at characterizing conductive and non-conductive materials, which is an essential attribute given the diverse nature of samples in scientific inquiry. The technique of coating non-conductive materials with a thin layer of conductive material often enhances SEM imaging without altering the sample's characteristics.
This versatility extends to various scientific fields. For instance, in materials science, SEMs can reveal which particle sizes, shapes, and distributions are most appropriate for specific applications, such as catalysts or structural materials. In environmental studies, SEMs help in understanding the interactions between pollutants and biological systems. Their ability to operate across a wide range of scenarios—whether they are investigating micro-structures in a metal alloy or the intricate textures of biological tissues—makes them indispensable tools in contemporary research settings. Their adaptability is akin to a Swiss Army knife, equipped to handle an array of tasks, each with unique demands.
"SEMs empower researchers with unparalleled capabilities for material analysis, fostering innovations across multiple domains."
In summary, the advantages of Scanning Electron Microscopes are profound and multifaceted. From high-resolution capabilities that unveil the minute details of surfaces to the remarkable three-dimensional imaging that enhances understanding of complex structures, SEMs represent a cornerstone of modern microscopy. Their versatility further cements their position as essential instruments across a multitude of disciplines.
Understanding these advantages not only highlights the importance of SEMs but also underscores their impact on the advancement of countless fields of study.
Limitations of Scanning Electron Microscopes
While scanning electron microscopes (SEMs) have transformed the landscape of microscopic analysis, they come with their own set of limitations that are crucial to understanding. Recognizing these constraints is essential for anyone working in the field, as it allows researchers and practitioners to set realistic expectations and determine the most suitable methods for their specific applications. As formidable as SEMs are in producing high-resolution images, factors like sample preparation and the vacuum environment can significantly impede their function.
Sample Preparation Requirements
Preparing samples for SEM analysis can be a meticulous task. Unlike optical microscopy, where samples may often be examined in their natural state, SEM necessitates a more rigorous process. Samples typically require a conductive coating, especially if they are non-metallic. This involves depositing a thin layer of a conductive material, such as gold or palladium, which can alter the sample's characteristics. This coating process can be a double-edged sword; while it improves image quality, it can also mask certain surface details, particularly if the layer is too thick.
Moreover, the necessity for samples to be sufficiently small and flat poses additional challenges. Larger or irregularly shaped samples may not fit well into the SEM chamber, leading to complications in imaging. This requirement can limit the types of materials that can be analyzed, particularly in fields like biology, where samples may be complex and three-dimensional in nature.
In terms of time and effort, sample preparation often ends up being the most labor-intensive phase of using an SEM. Each sample may take significantly longer to prepare than to scan, leading to bottlenecks in research.
Vacuum Environment Constraints
Another substantial limitation of SEMs is their requirement for a vacuum environment. The electron beam generated in these microscopes needs a vacuum to travel freely without interference from air molecules — which can scatter electrons and degrade image quality. However, this vacuum presents its own hurdles.
For one, the need for a vacuum means that any volatile materials or non-solid samples can be problematic. Liquid samples, for instance, cannot be measured directly in an SEM. Instead, they must be frozen or otherwise stabilized, which further complicates the preparation process and may alter the nature of the sample.
Additionally, maintaining a vacuum requires specialized equipment and can be costly and time-consuming. Any leaks or fluctuations can lead to interruptions in measurement and analysis, affecting the reliability of results. This constraint also makes certain types of in situ experiments impossible, where real-time observation in a non-vacuum state could offer significant insights.
Thus, while scanning electron microscopes have changed the game in scientific imaging, understanding their limitations is as vital as leveraging their strengths, particularly for high-caliber research.
In summary, recognizing the limitations related to sample preparation and vacuum requirements is not just about identifying challenges. It’s about strategizing how best to use SEM technology efficiently while knowing when to consider alternative methods in microscopy.
Recent Advances in Scanning Electron Microscopy
Recent advancements in scanning electron microscopy (SEM) have allowed this technology to remain at the cutting edge of scientific inquiry. The importance of keeping up with these advancements cannot be overstated, particularly for those working in fields that rely on precise imaging and analysis. These innovations not only enhance imaging capabilities but also broaden the operational scope of SEMs. In today’s rapidly evolving technological landscape, the focus is on improving efficiency, accuracy, and overall usability of these instruments. Through this lens, the recent advances in automated imaging and integration with other analytical techniques play a pivotal role.
Automated Imaging Techniques
One significant leap in SEM technology is the development of automated imaging techniques. Traditionally, operating an SEM required a high level of expertise and manual intervention, which could be time-consuming and subject to human error. Automated systems now allow for high-throughput imaging, enabling users to capture images with minimal user input. This significantly streamlines workflows and frees researchers to focus on interpretation rather than just data collection.
Some benefits of automated imaging techniques include:
- Consistent Image Quality: Automation reduces variability in image acquisition, leading to more reliable results.
- Increased Efficiency: Automation can process more samples in less time, which is crucial for large-scale studies.
- User-Friendliness: With advanced software guidelines, even those with limited SEM experience can competently operate the system.
- Advanced Data Analysis: Integration of AI and machine learning with SEMs now allows for sophisticated analysis of captured images, identifying features that might be missed by the human eye.
Moreover, automating focus, stigmation, and alignment processes minimizes the downtime typically encountered in manual operations. This development not only encourages broader access to SEM technology but also propels the research capabilities of institutions that may have previously been constrained by a lack of qualified personnel.
Integration with Other Techniques
Additionally, we are seeing an upsurge in the integration of SEM with other microscopy and analytical techniques. This melding of technologies enhances the potential to glean more comprehensive data from a single experiment. For example, coupling SEM with energy dispersive X-ray spectroscopy (EDX) allows, accurate elemental analysis alongside high-resolution imaging. This kind of synergy enables researchers to tackle complex problems, particularly in materials science and nanotechnology.
Some notable integrations include:
- Scanning Transmission Electron Microscopy (STEM): By merging SEM with transmission techniques, users can attain both surface and internal structural information, enriching their understanding of material properties.
- Focused Ion Beam (FIB): This combination allows for site-specific analysis and sample preparation for nanoscale studies, providing insights into the microstructure of materials that are otherwise inaccessible.
- X-Ray Photoelectron Spectroscopy (XPS): When SEM is integrated with XPS, researchers can obtain chemical state information of elements in addition to visualizing surface morphology.
These integrations not only augment analytical capabilities, but they also propel SEM applications into new fields of study. The expanding horizons of SEM capabilities reflect the ongoing evolution in science and technology, catering to the ever-increasing complexity of materials and biological systems that researchers face today.
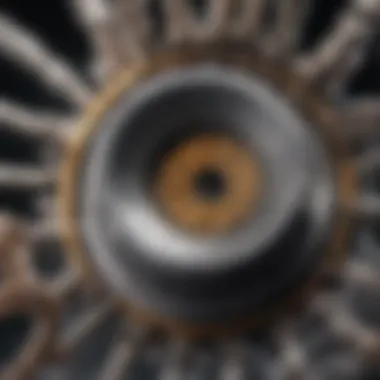
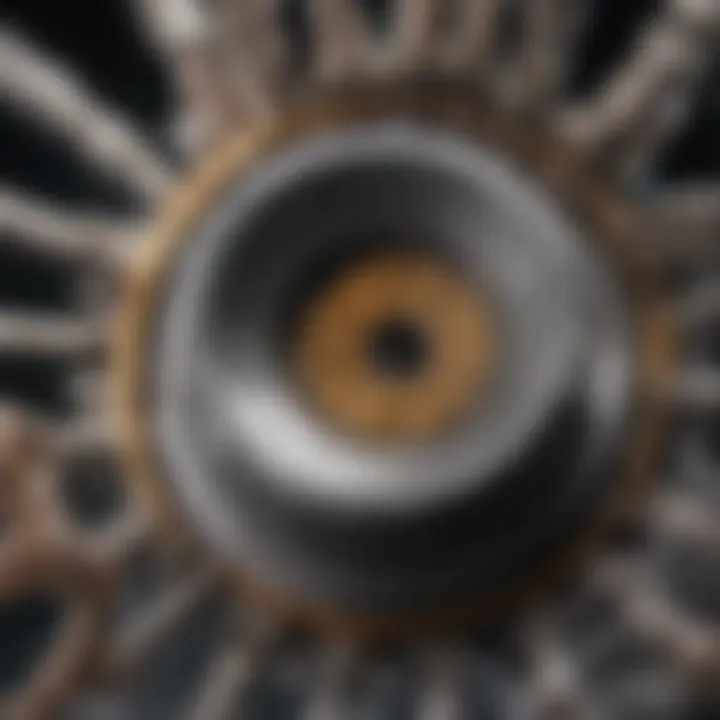
Advances in scanning electron microscopy open new avenues of research and deeper insights into the fundamental properties of materials, ultimately enhancing our understanding of the universe at the micro level.
Comparative Analysis with Other Microscopy Techniques
In the realm of microscopic imaging, scanning electron microscopes (SEMs) hold their ground as powerful tools, but they are not alone on this battlefield. Understanding how SEMs stack up against other microscopy techniques can illuminate both their strengths and their weaknesses. This comparative analysis sheds light on various methods of microscopy, exploring the nuances that make each approach suitable for particular tasks while highlighting how SEMs fit into the grander scheme of scientific observation.
Transmission Electron Microscopy
Transmission Electron Microscopy (TEM) is like the older sibling in the family of electron microscopy. It operates on differing principles compared to SEM, primarily by transmitting electrons through a very thin specimen. This technique results in considerable detail at the atomic level, which can be especially essential for fields such as materials science and biology. While SEM provides surface details, TEM reveals subsurface structures and compositions.
One significant characteristic of TEM is its ability to provide images with extremely high resolution, down to the atomic scale. This is essential when scientists are interested in observing phenomena at such a small scale. Besides resolution, the contrast in TEM is primarily dictated by the electron density of the sample, which can sometimes complicate imaging of low-contrast samples.
However, TEM comes with its challenges. The sample preparation is considerably more rigorous than that required for SEM, often demanding ultra-thin sections which can introduce artifacts. Additionally, the operational complexity and expense of TEM instruments can limit access for some research teams.
Atomic Force Microscopy
On another front, Atomic Force Microscopy (AFM) provides an alternative that is fundamentally different from both SEM and TEM. This technique scans a sample's surface using a fine tip that interacts with surface atoms, producing topographical maps at a resolution close to atomic level. Unlike SEM’s reliance on electron beams, AFM can operate in various environments, including liquids, making it a versatile choice for biological and polymer samples.
AFM excels in providing three-dimensional surface profiles of materials, revealing not just height but also mechanical properties. Some key advantages are the ability to image insulating materials without any special preparation and the potential for functional imaging. This allows researchers to characterize nanostructures with distinct functional properties, which SEM might miss.
Nevertheless, AFM typically has a lower imaging speed and can be more susceptible to noise from vibrations or thermal drift. Furthermore, while it provides exceptional detail of surface morphology, it doesn’t provide the depth information that SEM or TEM might achieve.
The choice of microscopy technique must align with the specific requirements of the research question being investigated. By comparing methods, scientists can select the most appropriate tool tailored for their needs.
The End
The comparative analysis elucidates the unique advantages and limitations of SEM, TEM, and AFM, showcasing why no single technique dominates the field of microscopy. Each method has its particular strength — whether it’s the high surface resolution of SEM, the atomic insights from TEM, or the versatility offered by AFM. Understanding these differences enables researchers to harness the right tools for their inquiries, ensuring that the path of scientific exploration is paved with the most robust data and insights.
Future Directions for Scanning Electron Microscopy
The field of scanning electron microscopy (SEM) is evolving rapidly, and looking ahead, it’s clear that we are on the brink of significant transformations. As researchers and technologists aim to enhance the utility of SEMs, exploring future directions is not just beneficial—it is crucial. This section discusses various emerging technologies that are likely to revolutionize SEM capabilities. Additionally, we will consider potential new applications that could benefit countless disciplines.
Emerging Technologies
The next frontier for SEM technology is heavily influenced by advancements in several key areas. First off, machine learning and artificial intelligence are starting to play an integral role in image processing and analysis. These tools allow for faster classification of materials and more accurate identification of features in the samples. It’s almost as if one is getting an expert’s opinion at lightning speed. Moreover, automated systems for high-throughput imaging are being developed, allowing scientists to process vast amounts of data without being bogged down by tedious manual analyses. This capability will tremendously boost productivity in laboratories.
In addition to software developments, hardware refinements are equally noteworthy. Field Emission Guns (FEG) are becoming more prevalent due to their ability to produce higher resolution images. This means researchers can capture finer details of the sample morphology, which is essential in fields like nanotechnology. Similarly, innovations in electrical and electron optics are paving the way for techniques that enhance the reliability of measurements under a wider range of conditions.
Potential New Applications
As SEM technology advances, so too do the potential applications across various fields. One of the most exciting prospects is the integration of SEM with in situ analysis techniques. This approach enables researchers to observe dynamic processes in real-time, paving the way for discoveries in materials science that were previously unattainable. For instance, monitoring the crystallization of an alloy can lead to insights into its structural integrity and ultimately enhance its performance.
Furthermore, SEM could find its place in environmental sciences. For example, characterizing aerosols and pollutants on a micro-level can provide crucial information for climate research. Understanding the interactions of particles in various environments may help researchers devise strategies to mitigate pollution effectively.
Additionally, with continued advancements, we may see SEM being applied in clinical and diagnostic settings. Examining biological specimens at a microscopic level could lead to breakthroughs in diagnostics, particularly in detecting cellular abnormalities related to diseases.
"The future of scanning electron microscopy is not just about improved images; it’s about transforming how we explore and understand the minute structures of the world around us."
In summary, the future of scanning electron microscopy is poised to be marked by technological innovations that not only enhance functionality but also unlock new avenues of research across multiple disciplines. This evolving landscape promises not only to improve existing practices but also to open doors to groundbreaking applications that can have profound impacts on science and medicine.
End
The conclusion of this article serves to reinforce the significant role of scanning electron microscopes (SEMs) in modern science. As we’ve explored in the earlier sections, SEMs provide invaluable insights into materials across various disciplines, from biology to nanotechnology. Their ability to produce highly detailed images at the microscopic level cannot be understated. This technological marvel has not only enhanced our understanding of material properties but also facilitated innovations in research methodologies.
In summarizing the key points, it’s clear that SEMs excel in high-resolution imaging and three-dimensional analysis. Their versatility makes them a critical tool for researchers striving to push the boundaries of knowledge. However, it’s essential to remember the limitations, such as the stringent sample preparation and vacuum requirements that can hinder real-time observation.
Understanding these elements equips researchers with a clearer perspective on how to leverage the strengths and recognize the constraints of scanning electron microscopy. Moving forward, the continuous evolution of SEM technology promises to open new avenues of exploration, further embedding SEMs into the fabric of scientific discovery.
Summary of Key Points
- High Resolution: SEMs provide detailed images, essential for analyzing surfaces and structures at the nanoscale.
- Three-Dimensional Imaging: The ability to generate 3D representations aids in better data representation and interpretation.
- Applications Across Fields: SEMs are employed in disciplines like materials science, biology, and nanotechnology.
- Limitations: Despite their strengths, SEMs also come with challenges in sample preparation and operational constraints.
"Scanning electron microscopy has reshaped the landscape of material analysis and has become a cornerstone in scientific research."
Importance of SEM in Future Research
The future of scanning electron microscopy looks quite promising as it continues to evolve alongside advancements in technology. As fields such as nanotechnology and materials science push forward, SEMs will undoubtedly play a pivotal role in addressing complex questions and challenges. Here are several considerations about the future implications of SEM:
- Integration with Emerging Technologies: The blending of SEM with techniques like cryo-electron microscopy can lead to groundbreaking discoveries in biomolecular structures, potentially revolutionizing our understanding of life at the molecular level.
- Real-time Imaging Capabilities: Future developments may focus on enhancing the imaging speed of SEM, allowing researchers to capture dynamic processes in samples. This could offer unprecedented insights into reaction mechanisms and material behaviors under different conditions.
- Broader Accessibility: As SEM technology becomes more affordable and user-friendly, a wider range of disciplines may start utilizing these sophisticated tools.
- New Areas of Exploration: SEMs could also venture into fields like archaeology and forensic science, providing detailed analysis of artifacts or evidence with a level of precision that's previously unattainable.