Exploring Quantitative Phase Contrast Microscopy
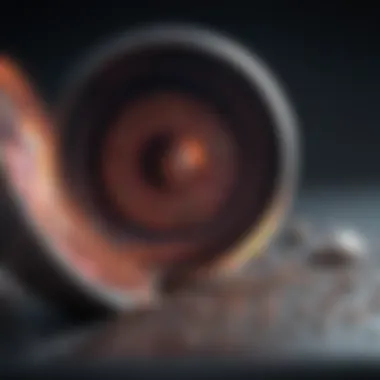
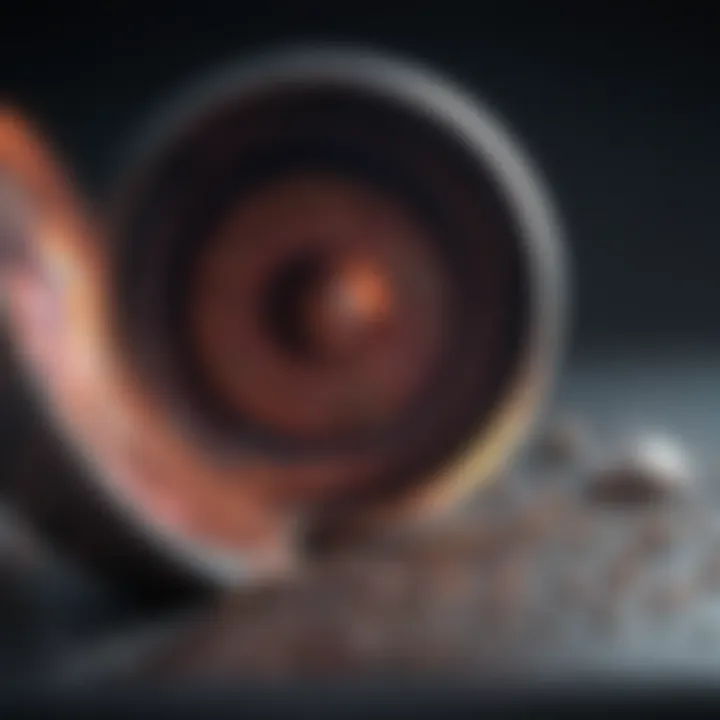
Intro
Quantitative phase contrast microscopy (QPCM) offers a powerful method for studying transparent biological specimens. Unlike traditional microscopy techniques that often rely on staining to enhance visibility, QPCM captures images based on phase shifts in light as it passes through different transparent materials. This allows researchers to visualize live cells and intricate biological processes without altering the specimen's native state. The technique is gaining traction across various fields of biomedicine, cell biology, and material sciences.
Through this exploration, we will investigate several critical aspects of QPCM, including its foundational principles, methodologies, and significant applications. A careful examination of advancements in technology and the challenges faced by practitioners will also give us insight into the future of this imaging technique.
Key Findings
Major Results
QPCM stands out due to its ability to produce quantitative data about the optical thickness of biological samples. This quantitative aspect differentiates it from standard phase contrast microscopy, which primarily offers qualitative insights. Studies have shown that QPCM can effectively monitor cellular behaviors, such as migration and proliferation, under various conditions. This provides a robust platform for real-time observations in living organisms.
Further, enhancements in image processing techniques have led to improved accuracy in measurements derived from QPCM. The integration of machine learning algorithms with QPCM has shown promising results in automating the analysis process, which could greatly enhance productivity in research settings.
Discussion of Findings
The results indicate that QPCM is not merely an imaging method; it is a versatile tool that elevates our understanding of cellular dynamics. Observations from live-cell imaging have revealed critical information about cellular structures and their functions. Researchers have noted that QPCM is particularly effective in visualizing features that are typically difficult to discern, further emphasizing its role in advancing biological research.
Additionally, the applicability of QPCM spans across multiple disciplines—ranging from developmental biology to cancer research. Each study highlights its utility in revealing insights that are not accessible through conventional microscopy approaches.
Methodology
Research Design
The methodology employed in QPCM studies often involves a combination of optical physics and biological techniques. Most studies typically begin with the design of the optical system, ensuring that it is capable of capturing the phase information effectively. The experimental setup usually includes a light source, a phase contrast optical system, and a digital imaging device to gather data.
Data Collection Methods
Data collection in QPCM involves two primary methods: direct imaging of live specimens and subsequent computational analysis. Researchers capture time-lapse images of live cells, allowing for the monitoring of cellular events over time. Using sophisticated software, they process these images to extract quantitative phase data.
The meticulous approach ensures that the phase shift information is accurately translated into meaningful biological interpretations. These interpretations can then be used to inform future experiments or clinical applications.
"QPCM provides an unrivaled opportunity to visualize and understand the complexity of biological systems in real-time, offering insights that traditional microscopy cannot deliver."
As we continue, the exploration will delve deeper into specific applications and real-world implications of QPCM in various fields of research.
Prelude to Quantitative Phase Contrast Microscopy
Quantitative Phase Contrast Microscopy (QPCM) represents a significant leap in imaging transparent biological specimens, allowing researchers to observe cellular structures with great clarity and detail without the need for staining. This enhances our understanding of various biological processes. The importance of this technique lies in its ability to provide quantitative data on cell morphology and dynamics, making it invaluable in biological research and medical diagnostics.
Definition and Concept
Quantitative Phase Contrast Microscopy is a technique that transforms phase shifts in light as it passes through a specimen into quantitative data. Traditional microscopy often relies on staining to enhance the visibility of cellular components. However, QPCM allows for a non-invasive approach, thus preserving the natural state of biological samples. The concept revolves around measuring the optical path length differences induced by the various refractive index of cellular components. As a result, researchers can obtain high-contrast images and accurate quantitative measurements such as cell thickness, volume, and refractive index variation.
Historical Background
The foundation of phase contrast microscopy was laid by Fritz Zernike, who introduced the concept in the 1930s. His invention aimed to visualize transparent objects, which were often difficult to see using traditional optical methods. Over the decades, advancements led to the integration of quantitative methods into this microscopy form, resulting in QPCM. The evolution of digital imaging technology has further propelled the progress of QPCM, leading to enhanced detection sensitivity and imaging speed. Understanding this historical context enriches the appreciation of the method's current capabilities and potential future developments.
Fundamental Principles of QPCM
Understanding the fundamental principles of Quantitative Phase Contrast Microscopy (QPCM) is essential for comprehending how this technique operates and the unique advantages it offers in imaging biological specimens. QPCM operates on the premise of phase shift and light interaction with matter. By mastering these principles, researchers gain insights into the quantitative aspects of imaging, which can reveal critical details about cellular structures without the need for complex staining or sample modifications.
Light Interaction with Matter
In QPCM, light behaves as both a wave and a particle. When light encounters a biological sample, it interacts with various components of the specimen, leading to different effects. The interaction depends on factors like the refractive index, thickness, and optical properties of the material.
These interactions can be categorized as:
- Refraction: Light bends as it passes through materials of differing densities. This bending alters the path of light, which is pivotal in capturing clear images of thin samples.
- Reflection: Some portion of the light is reflected off the surface of the specimen. QPCM utilizes this reflective light to enhance contrast and detail.
- Transmission: Light passing through the sample is key for obtaining images. The extent of transmission influences the results captured in QPCM.
By measuring these interactions, researchers can extract quantitative information about cell morphology and dynamic processes within living cells. Mimicking how our eyes perceive these changes, QPCM translates the alterations in phase into observable images, offering a view of living cells over time without damaging them.
Phase Shifts and Interference Patterns
The interplay of light waves is central to QPCM. When light encounters different regions of a sample, it undergoes phase shifts. These shifts occur due to variations in the optical path length as light moves through materials of differing refractive indices.
When two light waves combine, they create interference patterns, which are crucial for QPCM. The interference can be constructive or destructive:
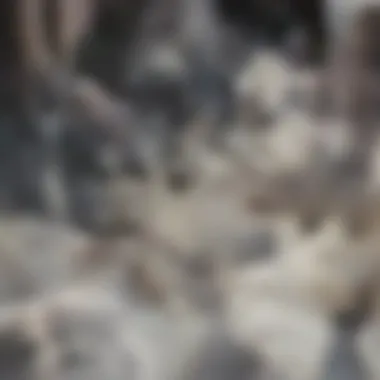
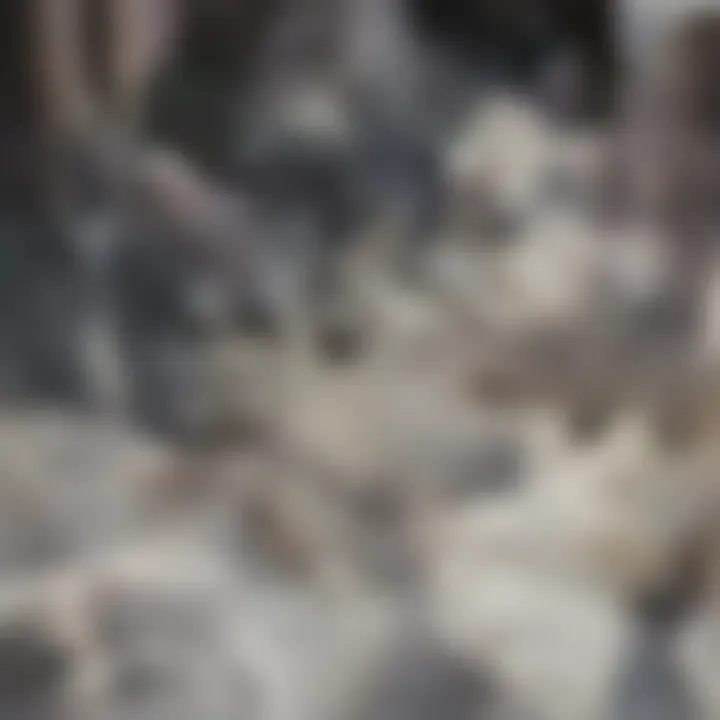
- Constructive interference occurs when the phase difference between the two waves is a multiple of 2π, amplifying the signal and contributing to brighter regions in the image.
- Destructive interference happens when the phase difference is a half multiple of π, reducing the signal strength and leading to darker areas in the rendered image.
By analyzing these interference patterns, researchers can quantify phase shifts, thus obtaining detailed information about the sample. This capability allows for enhanced imaging, making it possible to visualize cellular details that are otherwise invisible using conventional light microscopy.
"Quantitative phase contrast microscopy not only delivers images but also provides information embedded within the samples, allowing for a deeper understanding of cellular dynamics."
In summary, the concepts of light interaction and phase shifts form the backbone of QPCM, enabling researchers to explore biological specimens with unprecedented clarity. By leveraging these principles, QPCM stands as a significant advancement in the realm of imaging technologies.
Key Components of QPCM Systems
Quantitative phase contrast microscopy (QPCM) is a powerful tool in the realm of imaging, particularly for biological studies. Understanding the key components of QPCM systems is essential to appreciate how this technique functions effectively. The success of QPCM relies on several specific elements that collectively contribute to its ability to visualize transparent specimens with high precision. Each component plays a critical role in enhancing the imaging capabilities, providing researchers with greater insights into biological processes.
Optical Setup
The optical setup of a QPCM system is fundamental to its performance. It primarily includes the light source, the objectives, and the phase plates. The light source often uses coherent light, like a laser, to illuminate the specimen uniformly. This coherence is crucial as it enhances the contrast in phase, enabling the detection of subtle changes in the optical path length within the cells.
The use of special objectives can significantly improve the imaging quality. These objectives are designed to focus light tightly onto the sample, allowing more detailed observations of thin specimens. Phase plates, placed in the optical path, manipulate the light to induce phase shifts that are critical for forming the quantitative images. These setups allow researchers to capture images that can render quantifiable data on cell structures and behaviors without staining.
Detector Technology
Detector technology is another critical aspect of QPCM systems. The detectors convert the light signals obtained from the specimen into digital images that can be analyzed. Common detectors used in QPCM include charge-coupled devices (CCDs) and complementary metal-oxide-semiconductor (CMOS) sensors.
CCDs are renowned for their sensitivity and low noise, offering high-quality images even under low illumination conditions. This makes them ideal for observing delicate biological specimens. On the other hand, CMOS sensors, while traditionally less sensitive, have made significant advancements in speed and resolution.
"The choice of detector can markedly affect the quality and specifics of the data gathered in QPCM research."
The integration of these detectors with sophisticated imaging software allows for advanced data processing and analysis. This capability is crucial for extracting meaningful quantitative information from phase images, enabling researchers to analyze physiological properties such as cell health, morphology, and interactions.
In sum, the key components of QPCM systems, which include the optical setup and detector technology, are paramount for achieving high-resolution, quantitative imaging of biological specimens. Understanding these components allows users to optimize their QPCM configurations, ultimately enhancing the research quality and enabling more precise biological insights.
Quantitative Imaging Techniques
Quantitative imaging techniques play a vital role in advancing our understanding of biological processes at the cellular level. These techniques enable researchers to obtain precise measurements of cellular structure and behaviors without the need for invasive methods or staining. This aspect is especially significant in studying living cells, as it preserves their natural condition and cellular dynamics.
The effectiveness of quantitative phase contrast microscopy lies in its ability to convert phase shifts caused by cellular components into measurable intensity variations. This improvement in visualization is not just aesthetic, but it also facilitates the analysis of various parameters such as cell morphology, motility, and even intracellular dynamics. Researchers benefit from accessing quantitative data, providing them with insights that are often unattainable through traditional microscopy methods.
Digital Holographic Microscopy
Digital holographic microscopy represents a highly sophisticated extension of traditional QPCM techniques. This method allows researchers to capture the 3D information of an object by recording the interference pattern of light waves.
One of the prominent benefits of this approach is its ability to reconstruct full-field images. The captured holograms can be processed later to extract quantitative information, such as phase shift data, which can be converted into thickness measurements of the specimen. This provides a non-invasive means of analyzing cellular structures, offering high spatial resolution and the ability to observe live cells over time. Furthermore, the digital storage of holograms allows for replay and analysis, leading to a deeper understanding of dynamic cellular processes.
Some key advantages of digital holographic microscopy include:
- Non-invasive observation: preserving live cell integrity
- High accuracy: delivering precise quantitative measurements
- Capability for time-lapse studies: monitoring dynamic events
Fourier Transform Methods
Fourier transform methods further enhance the capabilities of QPCM by applying mathematical techniques to analyze the data obtained from imaging. These methods transform spatial domain information into the frequency domain, which helps in identifying and quantifying different features of biological specimens.
By utilizing Fourier analysis, researchers can extract valuable information about the contrast and resolution limits of their imaging systems. This method assists in enhancing image quality while managing the noise levels that often accompany optical imaging. It is particularly effective in distinguishing features that may not be apparent in raw phase images.
Key aspects of Fourier transform methods include:
- Improved contrast: enabling better differentiation of similar structures
- Noise reduction: filtering out irrelevant data for clearer images
- Flexibility: applicable to various samples and conditions
"Quantitative imaging techniques, including digital holographic microscopy and Fourier transform methods, are essential for unlocking secret information within biological systems."
Overall, these quantitative imaging techniques significantly elevate our understanding of biological phenomena. They are instrumental in providing comprehensive insights required in both basic research and applied sciences.
Applications of QPCM in Biological Research
Quantitative Phase Contrast Microscopy (QPCM) has emerged as a pivotal tool in biological research, particularly for the study of live cells and tissues. This imaging technique allows scientists to investigate transparent specimens without the necessity of staining, preserving the inherent cellular features and functionality. The importance of QPCM in biological research lies in its ability to provide detailed, quantitative information about cellular structures and dynamics in real-time. This enhances our understanding of various biological processes and creates opportunities for innovations in diagnostics and therapeutics.
Cell Imaging and Tracking
The application of QPCM in cell imaging and tracking is significant for a number of reasons. First, it allows for the observation of live cells in their natural environment. Traditional imaging techniques often require fixing and staining cells, which can alter their normal state and potentially lead to incorrect interpretations. QPCM enables researchers to visualize cellular behavior without interference.

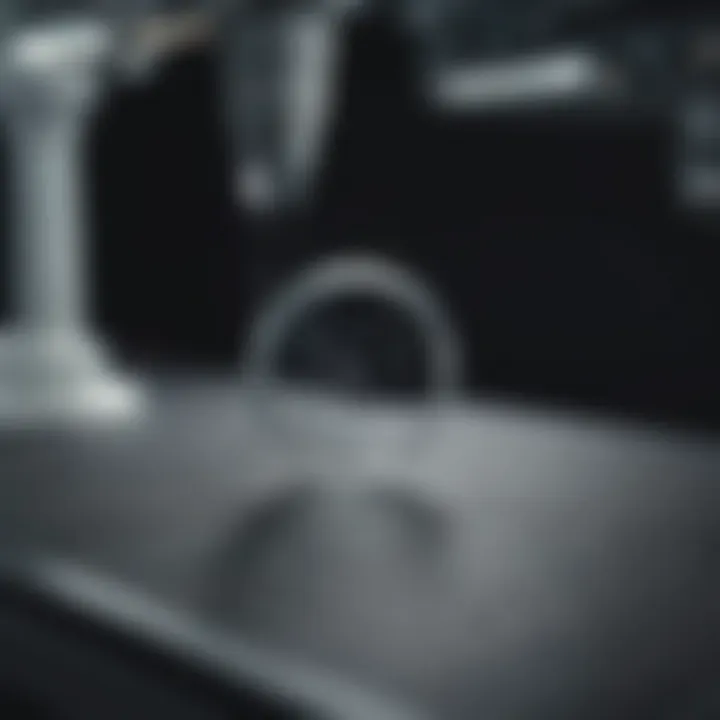
Researchers utilize QPCM for high-resolution imaging, capturing details such as cell morphology and motility. This is particularly useful in studying cancer cells. For instance, tracking their movement can reveal insights about invasion and metastasis. The quantification of phase shifts aids in detecting changes in cellular density and structural variations, which can indicate physiological responses to external stimuli.
Moreover, QPCM contributes to the analysis of cellular processes in a non-invasive manner. With advancements like digital holographic microscopy, researchers can achieve significant improvements in imaging speed and resolution. This technology allows for the monitoring of live cell processes, such as division and differentiation, without losing valuable data about their microenvironment.
Studying Cellular Processes
Studying cellular processes with QPCM provides a powerful means of understanding complex biological systems. The quantitative data obtained through phase contrast can reveal not only static images but also dynamic behaviors of cells in suspension or in culture. For example, researchers can observe and quantify cell metabolism and growth rates effectively.
Additionally, QPCM facilitates the investigation of cellular interactions, such as cell-cell communication and responses to pathogens. The ability to visualize these interactions offers deeper insights into mechanisms underlying immune responses and disease progression.
However, challenges remain in interpreting the quantitative data from QPCM. The data processing complexity can hinder rapid analysis and real-time observation. Researchers must develop robust algorithms and processing techniques to extract meaningful information from the volumetric data collected.
To summarize, the applications of QPCM in biological research significantly enhance our capability to visualize and quantify biological processes in real-time. By employing techniques like cell imaging and studying cellular behavior dynamically, researchers can draw reliable conclusions that advance our understanding of cellular biology. The non-invasive nature of QPCM further provides a distinct advantage in preserving the natural state of specimens.
Advantages of Quantitative Phase Contrast Microscopy
Quantitative phase contrast microscopy (QPCM) offers several distinct advantages that enhance its utility in biological research. By not requiring staining, this technique allows for the observation of living cells in their natural state. The insights gained from QPCM can be crucial for studies involving cellular behavior and dynamics.
Non-Invasive Imaging
One of the most significant benefits of QPCM is its non-invasive nature. Traditional microscopy techniques often rely on dyes that can disrupt cell processes or alter the physiological states of specimens. In contrast, QPCM captures images without any additional preparation, maintaining the biological integrity of samples. This feature is especially relevant in time-lapse studies, where cellular changes over time are to be monitored. Efficacy in observing viable cells allows researchers to conduct experiments without introducing external variables that could skew results.
In addition to preserving cell viability, QPCM minimizes photodamage that can occur with prolonged light exposure. High-intensity light sources used in other techniques may lead to alterations in cellular structures or functions. QPCM, by utilizing a lower intensity light, circumvents such complications, making it suitable for long-term observations. The ability to visualize dynamic processes in situ opens a door for more realistic biological assessments.
Enhanced Contrast and Resolution
Another notable aspect of QPCM is its capacity for enhanced contrast and resolution. Unlike conventional light microscopy, which can struggle to adequately visualize transparent samples, QPCM effectively converts phase shifts into intensity variations in images. This property facilitates the differentiation of organelles or other structures within the cell that would otherwise be indistinct under traditional techniques.
By improving contrast without the need for additional labels or stains, QPCM provides clear images that allow for precise measurements. The enhanced resolution enables researchers to conduct quantitative analysis of cellular features, enabling better understanding of various biological processes. This is particularly relevant in areas such as cell morphology, volume measurements, and morphological variations.
"The strength of QPCM lies in its ability to elucidate cellular features that are often missed in standard imaging techniques."
Simplicity in observing live specimens lends itself to various applications, such as tracking cell migration and analyzing division processes. Moreover, advancements in QPCM technology continually improve its capabilities, promising even better resolution and sensitivity in the future.
In summary, the advantages of quantitative phase contrast microscopy, including non-invasive imaging and enhanced contrast, position it as a vital tool in modern biological research. These strengths render QPCM a preferred methodology for scientists looking to explore the intricacies of cellular life without compromising the integrity of their samples.
Limitations and Challenges
Understanding the limitations and challenges of Quantitative Phase Contrast Microscopy (QPCM) is crucial for its application and development. While QPCM offers significant advantages in imaging biological specimens, it is not without its obstacles. This section examines key limitations regarding sensitivity to environmental fluctuations and the complexity of data processing. Recognizing these challenges is essential for researchers to optimize their methodologies and improve the reliability of results.
Sensitivity to Environmental Factors
One of the primary challenges associated with QPCM is its susceptibility to environmental factors. Fluctuations in temperature, humidity, and vibrations can impact the stability of the optical system, leading to variations in phase measurements. These environmental changes can introduce noise into the imaging process, therefore affecting the clarity and accuracy of the captured images. Maintaining a stable environment for QPCM is important for consistent results.
Some strategies can mitigate these effects:
- Use of vibration isolation tables to minimize external disturbances.
- Temperature control systems to maintain a consistent environment during imaging sessions.
- Regular calibration of the system, ensuring that deviations are accounted for.
Despite these approaches, total elimination of environmental influence is difficult. This inherent sensitivity can pose limitations in field studies or less controlled laboratory settings, where conditions are variable. Promoting better environmental control standards in research facilities may help improve QPCM's performance under challenging conditions.
Data Processing Complexity
Data processing in QPCM is another critical limitation worth discussing. The imaging techniques in QPCM generate large datasets that require sophisticated algorithms for analysis and interpretation. These datasets often contain intricate phase information that needs to be accurately reconstructed to produce meaningful images.
Several key aspects make the data processing complex:
- High computational requirements: Analyzing the volume of data generated by QPCM systems demands significant computational power and memory. This can lead to delays or bottlenecks during image processing.
- Advanced algorithms needed: Conventional imaging software may not suffice for processing QPCM data. Researchers often need to develop custom algorithms or adopt specialized software, increasing the time and expertise required.
- Potential for artifacts: Improper data processing can lead to artifacts, which may mislead interpretations of biological structures or processes.
Researchers must balance the demands of complexity with the need for efficient workflows. Training in advanced data processing techniques or collaborating with computational specialists may enhance the analysis, supporting the overall effectiveness of QPCM in research.
Developing strategies to address the challenges faced by QPCM will not only improve its application but also pave the way for further innovations in microscopy techniques.
Comparative Analysis with Other Microscopy Techniques
Quantitative phase contrast microscopy (QPCM) is often discussed in the context of various microscopy techniques. This comparative analysis serves to clarify the unique advantages and challenges of QPCM when positioned alongside traditional and modern methods. Understanding these distinctions is vital for researchers and educators who need to choose the most effective imaging technique for their specific applications.
Contrast Methods Comparison
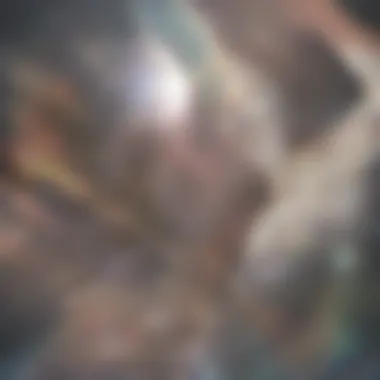
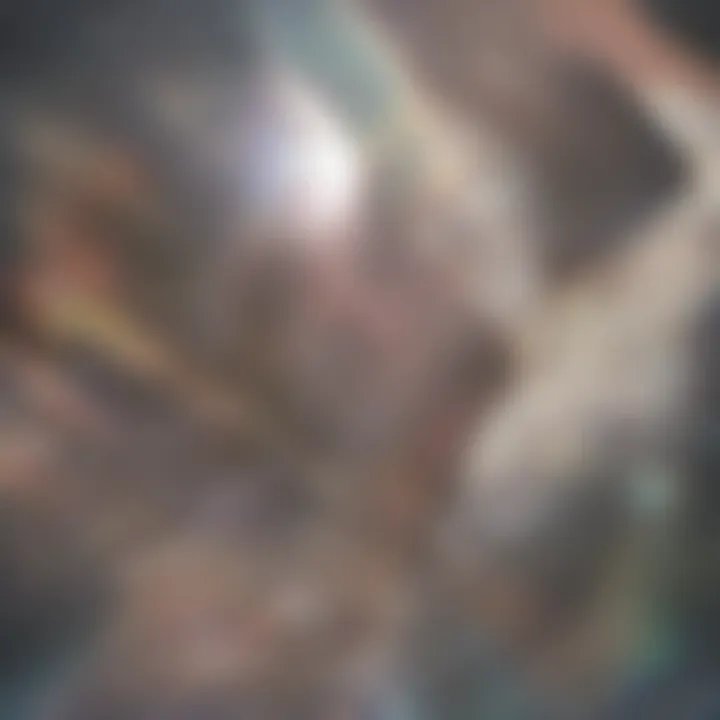
In microscopy, contrast is essential for visualizing specimens. QPCM enhances contrast of transparent biological samples without staining. This non-invasive approach contrasts with techniques like standard phase contrast microscopy or differential interference contrast microscopy, which also aim to boost visibility but may introduce artifacts.
- Phase Contrast Microscopy: This relies on phase shifts from the specimen, producing contrast that can sometimes misrepresent fine structures.
- Differential Interference Contrast: This type uses polarized light and can create false relief of structures, which may not always reflect true morphology.
On the other hand, QPCM quantitatively measures phase differences, leading to greater accuracy in assessing cellular dimensions and refractive indices. This precision is crucial for experiments assessing cell health or functionality over time.
Moreover, because QPCM does not require staining, it reduces the risk of disrupting cellular processes. This characteristic enables long-term live-cell imaging, providing insights into dynamic biological phenomena.
Resolution Capabilities
Resolution is a critical factor in microscopy, determining the clarity and detail of the images produced. QPCM provides high-resolution images comparable to techniques like confocal microscopy and super-resolution microscopy. The unique ability of QPCM to characterize refractive index variations contributes to resolving minute details that other methods might obscure.
Key Points to Consider:
- Spatial Resolution: QPCM maintains high spatial resolution enabling effective imaging of subcellular structures.
- Temporal Resolution: With its fast acquisition times, it allows for capturing rapid cellular events, essential for time-lapse studies.
In summary, while techniques like confocal and electron microscopy provide excellent structural resolution, they often come with significant limitations in specimen preparation. QPCM's combination of non-invasive imaging and strong resolution capabilities makes it a valuable tool in contemporary biological research and medical diagnostics.
Future Directions in QPCM Research
The field of quantitative phase contrast microscopy (QPCM) is continually evolving. As technology advances, several promising pathways for future research emerge. These developments not only improve the technique itself but also expand its applications across various disciplines. Understanding these future directions is vital for researchers aiming to stay ahead. Innovations in technology and expanding applications will drive QPCM's relevance in both scientific research and clinical practice.
Innovations in Technology
Recent advancements in optical components and imaging software play a crucial role in the future of QPCM. One significant area of improvement includes the enhancement of detector technologies. High-sensitivity cameras, such as the CMOS and EMCCD, allow researchers to capture high-resolution images in low-light conditions. This is particularly valuable for studying delicate biological samples that may otherwise be damaged under conventional imaging techniques.
Moreover, algorithms for image reconstruction and analysis are being refined. Machine learning techniques are becoming more prevalent in processing phase contrast images. These methods can provide more accurate phase reconstruction and quantitative analysis, yielding insights that were challenging to achieve before.
Developments in digital holography also show potential. This approach can significantly reduce the optical complexity of QPCM while maintaining image quality. By using computational methods, researchers can capture three-dimensional information about specimens more efficiently. As these technologies continue to mature, they will likely lead to new imaging modalities and capabilities within QPCM.
Expanding Applications
The versatility of QPCM allows it to be applied in various fields beyond traditional biological research. Future directions focus on broadening its potential uses, bringing benefits to new areas of study. For instance, in material science, QPCM can be valuable for examining the phase structures of polymers and composite materials under various environmental conditions. This capability can lead to insights that enhance material design and durability.
In clinical settings, the integration of QPCM with other imaging modalities could improve diagnostic capabilities. For example, combining QPCM with fluorescence microscopy may enable researchers to analyze cellular morphology alongside specific protein expressions, providing a comprehensive view of cellular dynamics.
Furthermore, QPCM can enhance live-cell imaging techniques. This will allow scientists to monitor cellular processes in real time without the need for fluorescent labels or fixation, preserving the natural state of the cells.
Future research in QPCM holds the potential to impact numerous scientific fields, offering novel insights and methodologies that were once out of reach.
In summary, the future of quantitative phase contrast microscopy is bright, with technological innovations and expanding applications paving the way for exciting developments. As this field progresses, it will undoubtely contribute significantly to our understanding of both biological and non-biological systems.
Closure
The conclusion of this article encapsulates key elements discussed throughout the exploration of quantitative phase contrast microscopy (QPCM). Understanding the techniques and applications of QPCM sheds light on its profound implications in the realm of biological and medical research. As a technology that enhances visualization without the need for staining, QPCM stands out for its non-invasive approach, which preserves the natural state of biological specimens. This is particularly significant in fields where detail and accuracy are paramount.
Summary of Insights
Through this article, various aspects of QPCM have been thoroughly examined. The core insights include the fundamental principles of phase contrast, its operational mechanisms, and the critical role of digital processing in enhancing image clarity. Additionally, the comparative analysis revealed QPCM's competitive advantages over conventional microscopy techniques. These advantages lie in its ability to provide high-contrast images while maintaining resolution, leading to a deeper understanding of cellular dynamics. Furthermore, discussions on limitations emphasized the necessity for continued innovation to mitigate challenges such as sensitivity to environmental factors.
Overall, these discussions highlight that QPCM is not merely an imaging tool but a significant advancement that propels researchers towards unexplored territories in cellular analysis.
Implications for Future Research
The implications of QPCM for future research are profound and multifaceted. As technology advances, one can expect enhanced detector technologies and image processing algorithms to emerge. Innovations in artificial intelligence are likely to play a pivotal role in data analysis, making the extraction of quantitative information from phase contrast images more efficient.
Moreover, expanding applications beyond biology into materials science or nanotechnology could pave new pathways for discovery. Researchers might also explore integrating QPCM with other techniques, fostering a more comprehensive understanding of complex biological systems. These developments suggest a future where QPCM continues to evolve, ultimately leading to breakthroughs that could potentially revolutionize how scientists observe and interpret living systems.
Importance of References in Academic Writing
- Credibility: References lend authority to the content presented. Properly credited work indicates that the findings are grounded in previously established knowledge, which can bolster the credibility of the claims made within this article.
- Contextual Understanding: This area of research is nuanced. References can help situate QPCM within broader themes in microscopy and biological imaging. They provide context for advancements and challenges in the field, thus informing the reader about the intricacies involved in quantitative phase contrast applications.
- Resource for Further Study: References offer an invaluable resource for readers keen on diving deeper into specific techniques or applications of QPCM. For students, educators, and professionals, they represent a springboard for future investigation and learning.
Considerations Regarding References
When choosing references, it’s essential to evaluate their relevance, reliability, and recency. In rapidly developing fields such as microscopy technology, older references may no longer represent the state of the art. Thus, prioritizing recent studies and reviews is crucial. Furthermore, references should encompass a mix of both foundational texts and contemporary research findings to provide a comprehensive understanding of the field.
"References not only strengthen the legitimacy of scientific work but also promote an ongoing dialogue in research communities."
Practical Repository of References
Inclusion of well-regarded references enhances the article's overall integrity. Here are some suggestions for key resources that may be beneficial:
- Primary research articles from journals such as Nature Photonics and Journal of Biomedical Optics.
- Reviews and meta-analyses that summarize advancements in QPCM.
- Websites and platforms like Wikipedia and Britannica for general background information on microscopy terms and techniques.
- Social media platforms like Reddit and Facebook can provide discussions and shared research that may not yet be formally published.
In summary, the references section of this article not only supports the scholarly nature of the discourse but also serves as a vital resource for readers interested in further exploration of quantitative phase contrast microscopy.