Insights into Piezoelectric Crystal Ultrasound Technology
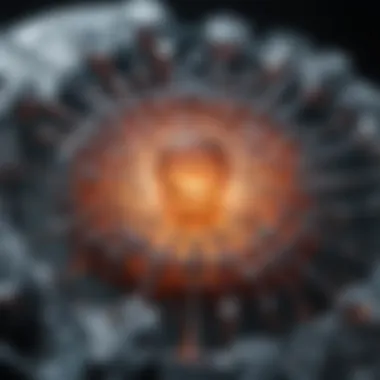
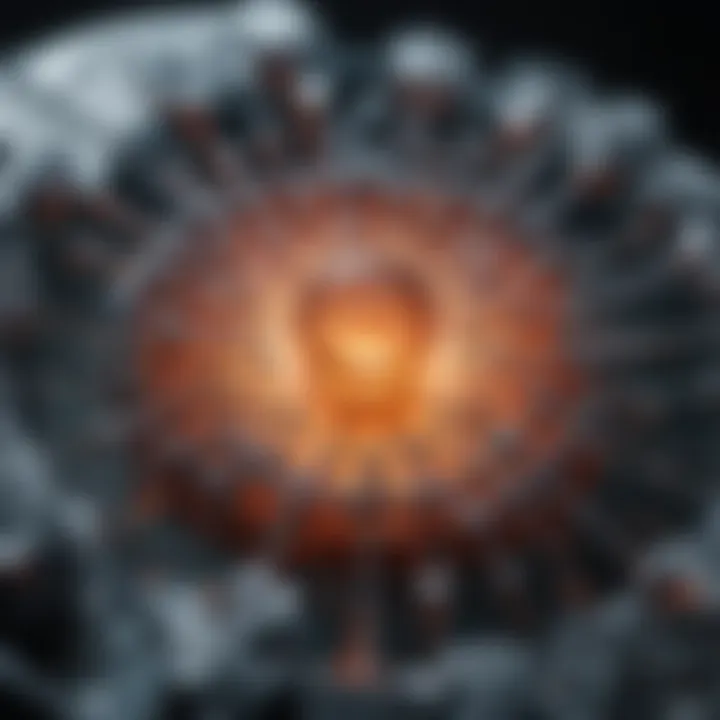
Intro
Piezoelectric crystal ultrasound has carved a niche for itself in various fields, transforming standard practices into highly efficient processes. To understand its significance, we must first grasp the fundamental principles of piezoelectricity. This unique characteristic allows certain materials to generate an electric charge in response to mechanical stress. In simpler terms, when these materials are squeezed or twisted, they produce voltage. Conversely, when an electric field is applied, they change shape. This dual functionality is at the heart of ultrasonic technology and has far-reaching implications in both medical and industrial sectors.
In the realm of medical imaging, piezoelectric ultrasound finds its home in diagnostic equipment, enabling doctors to visualize internal body structures non-invasively. This technology has evolved tremendously, transitioning from bulky equipment to portable devices that can be used in various clinical settings.
Yet, the intrigue doesn’t stop there. This article digs deeper, unearthing the intricate mechanisms behind piezoelectric materials, the challenges researchers face, and the promising applications on the horizon. Readers can expect a thorough exploration tailored for students, researchers, and professionals keen to elevate their knowledge on the topic.
Key Findings
The exploration of piezoelectric crystal ultrasound reveals significant insights and advancements in various applications. Among the major findings include:
- Enhanced Medical Imaging: Piezoelectric ultrasound substantially improves the accuracy and resolution of diagnostic imaging, helping in the early detection of diseases.
- Industrial Applications: This technology is used extensively for non-destructive testing, ensuring structural integrity in manufacturing processes by identifying flaws or defects without harming the material.
- Material Characterization: Piezoelectric properties are beneficial in characterizing new materials, providing insights into their performance and durability under stress.
"The intersection of materials science and ultrasound technology is reshaping how industries evaluate and utilize materials, paving the way for innovation."
Major Results
The findings indicate a strong correlation between the advancements in piezoelectric materials and the evolution of ultrasound applications. The use of higher frequency ultrasound with improved transducer designs has resulted in a significant increase in the resolution and clarity of images in medical diagnostics. In industry, there are concrete examples of how ultrasonic testing helps to maintain quality control and compliance with safety standards.
Discussion of Findings
The implications of these advancements are profound. With increased accuracy in medical imaging, earlier interventions can be facilitated, improving patient outcomes significantly. On the industrial front, as manufacturers strive for higher efficiency and safety standards, the role of piezoelectric ultrasound will likely expand. This emphasizes the necessity for continued research into optimizing these technologies further.
Methodology
The investigation into piezoelectric crystal ultrasound involved a structured approach to collect and analyze relevant data. The following sections outline the research design and data collection methods, providing clarity on how the findings were derived.
Research Design
This study adopted a mixed-method approach, combining quantitative data from experimental results of ultrasound applications with qualitative insights gathered from expert interviews. The multidisciplinary nature of the subject matter necessitated collaboration among researchers in physics, engineering, and medical fields.
Data Collection Methods
Data were gathered through:
- Experimental Trials: Conducting various tests to evaluate different piezoelectric materials under ultrasonic waves.
- Surveys: Collecting feedback from practitioners who utilize ultrasonic equipment in clinical and industrial applications.
- Literature Review: Analyzing existing research to identify trends, challenges, and breakthroughs in the application of piezoelectric ultrasound.
The outcomes from this methodological approach will aid in shaping the future research landscape, offering pathways for innovation and enhanced applications.
Preface to Piezoelectricity
Piezoelectricity is a fascinating topic that ushers us into the world of material science, playing a pivotal role in various technologies we encounter today. At its core, piezoelectricity refers to the ability of certain materials to generate an electric charge in response to mechanical stress. This concept is not merely theoretical; it has real-world applications that are both profound and varied, particularly in fields like medical imaging and industrial testing.
Understanding piezoelectricity helps in appreciating its significance in piezoelectric crystal ultrasound. The phenomenon is rooted in the unique properties of materials, which are specifically called piezoelectric materials. They respond to applied pressure by creating electrical signals, paving the way for innovative tools used across disciplines.
In essence, piezoelectric materials transform mechanical energy into electrical energy and vice versa. By incorporating this function into ultrasound technology, medical imaging has seen substantial progress, allowing for non-invasive examinations of the human body and making diagnosis more accurate and efficient.
Moreover, piezoelectricity does not only find its importance in healthcare; it also plays a critical role in industrial applications. This dual nature echoes the importance of piezoelectricity across various sectors, proving its relevance, versatility, and necessity in both scientific research and practical applications. Understanding this foundation will set the stage for deeper discussions on the mechanics of ultrasound technology and its various uses.
Definition and Historical Background
The term piezoelectricity derives from the Greek word "piezein," meaning to squeeze or press. This characteristic was first observed by Pierre and Jacques Curie in the late 19th century. They discovered that certain crystals generate electric charge when subjected to mechanical stress. This discovery signaled the beginning of extensive research into various materials and their potential applications.
Since then, piezoelectric materials have evolved, influencing innovations in diverse fields, including telecommunications, computing, and of course, medical imaging.
Key Characteristics of Piezoelectric Materials
Piezoelectric materials exhibit several unique features that make them suitable for both sensing and actuation applications. The most notable characteristics include:
- Bideirectionality: The ability to convert mechanical energy into electrical energy and vice versa makes these materials incredibly versatile.
- Sensitivity: High sensitivity to applied forces allows for accurate readings in sensors.
- Temperature Dependence: Performance can vary with temperature changes, which is crucial for applications requiring stability in diverse environmental conditions.
- Frequency Response: Piezoelectric materials can operate across a wide frequency range, allowing them to be tailored for specific applications.
These defining traits are vital in applications ranging from simple buzzers to complex medical imaging systems.
Types of Piezoelectric Crystals
In the realm of piezoelectric materials, several types stand out due to their distinct characteristics and applications. Notable among them are:
Quartz
Quartz is perhaps the most recognized piezoelectric material. Its natural availability and ability to produce a stable output make it a go-to choice in various applications. One key characteristic of quartz is its high mechanical strength, which allows it to withstand considerable stress without cracking.
Moreover, owing to its piezoelectric properties, quartz is often used in electronics like watches and sensors, providing accurate timing and efficient data collection. The purity of natural quartz also enhances its performance, making it a beneficial material in both the consumer electronics industry and more advanced scientific applications.
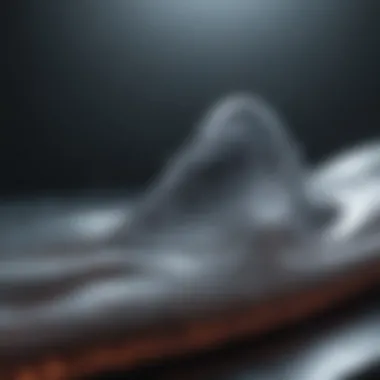
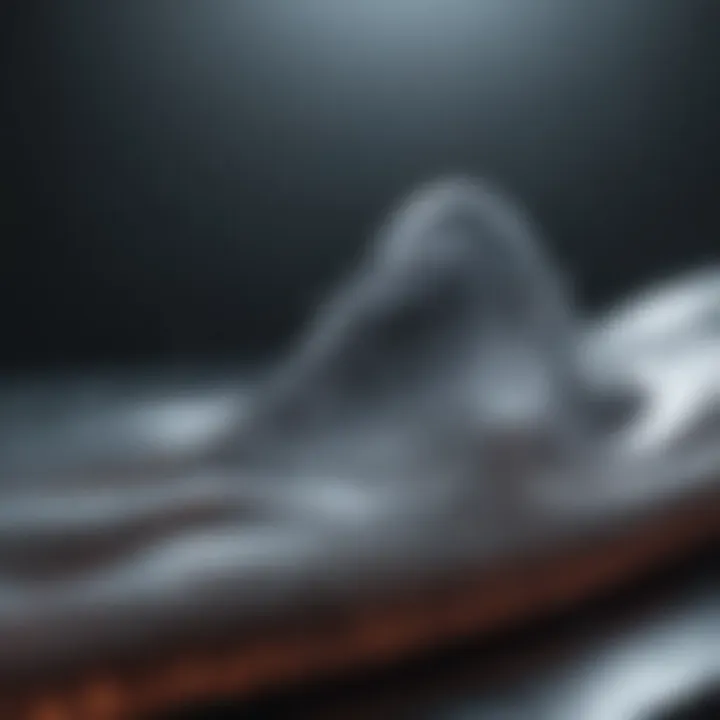
Tourmaline
Tourmaline is a lesser-known player in the piezoelectric sphere, yet it has distinct attributes worth noting. One standout feature of tourmaline is its ability to generate electric charge under pressure, which occurs in a uniquely nonlinear fashion. This characteristic opens up avenues for innovative applications in fields like energy harvesting.
Although not as widely used in standard ultrasound applications, tourmaline’s intriguing properties make it a subject of interest for research into alternative piezoelectric materials. It holds potential, especially in niche fields that explore energy sustainability and innovative resonance absorption techniques.
Lead Zirconate Titanate (PZT)
Lead Zirconate Titanate, commonly referred to as PZT, is a synthetic ceramic material renowned for its robust piezoelectric properties. This material is particularly adept at transforming electrical energy into mechanical energy and is extensively used in ultrasound transducers. The adaptability of PZT allows for a wide range of operational frequencies, making it applicable from industrial nondestructive testing to intricate medical imaging systems.
However, it's essential to note the environmental considerations surrounding PZT due to the lead content. This aspect necessitates research into safer alternatives, but the material's performance remains a benchmark in piezoelectric applications.
"Understanding piezoelectric properties not only broadens our knowledge but also opens up countless opportunities to innovate in technology and science."
In summary, piezoelectric crystals are the backbone of ultrasound technology, each type presenting its unique advantages and potential drawbacks. By delving into their characteristics, we lay the groundwork necessary for exploring their applications in ultrasound and beyond.
Principles of Ultrasound Technology
Understanding the principles of ultrasound technology is crucial for grasping how piezoelectric crystals work within this field. Ultrasound technology, primarily utilized in medical imaging, drilling, and industrial inspections, relies heavily on the intricate relationship between sound waves and their interactions with various materials. Not only does this technology advance imaging techniques, but it also paves the way for non-invasive methods in diagnostics and therapeutic procedures.
Overview of Ultrasound Waves
Ultrasound waves are essentially sound waves with frequencies higher than the upper audible limit of human hearing, typically above 20 kHz. These waves can travel through different mediums—air, water, and solids—each yielding variations in speed and attenuation.
Key Characteristics:
- Frequency: The frequency of ultrasound waves dictates their penetration depth and resolution capabilities. Higher frequencies provide finer detail but can't penetrate deeply, while lower frequencies can travel farther but with less resolution.
- Amplitude: This attribute determines the intensity of the sound waves, affecting the brightness in imaging applications.
- Wavelength: The wavelength is inversely related to frequency. A shorter wavelength corresponds to a higher frequency, which is often preferred in medical imaging.
Understanding these characteristics is essential for professionals leveraging ultrasound for various applications. As the need for precise and reliable imaging grows, ultrasound waves prove invaluable in providing real-time feedback in therapeutic settings.
Generation of Ultrasound via Piezoelectric Crystals
Piezoelectric crystals are the backbone of ultrasound generation. When these crystals are subjected to an electric field, they undergo a mechanical deformation and generate sound waves. This process can be summarized in the following steps:
- Electric Signal Input: An alternating current is applied to the piezoelectric crystal, causing the crystal to oscillate.
- Mechanical Vibration: The oscillation of the crystal generates pressure waves in the surrounding medium, which propagate as ultrasound.
- Transducer Functionality: The transducer, which houses the piezoelectric material, converts electrical energy to acoustic energy and vice versa.
This conversion mechanism is crucial in both diagnostic imaging and therapeutic ultrasound applications. For instance, medical ultrasound imaging relies not just on sending waves but also on receiving the echoes that bounce back from various tissues, allowing practitioners to visualize internal organs and structures.
Interaction of Ultrasound with Materials
When ultrasound waves encounter different materials, complex interactions take place, which are critical for accurate imaging and diagnostic outcomes. The primary interactions include:
- Reflection: When ultrasound waves hit a boundary between two different materials (like soft tissue and bone), some of the waves bounce back. This reflection creates the image as it delineates the contrast between different tissues.
- Refraction: This occurs when waves change direction as they pass through materials of varying densities, affecting their speed and trajectory. Understanding refraction is important for accurate imaging.
- Scattering: This phenomenon occurs when ultrasound waves encounter small particles or irregularities within tissues, causing them to scatter in various directions rather than reflecting straight back. This interaction can provide additional information about the tissue’s microstructure.
The overall effectiveness of ultrasound technology relies heavily on these interactions. It allows for precise imaging that can lead to early diagnosis, which is critical in healthcare settings. A better grasp of how ultrasound waves work with materials can also drive advancements in therapeutic applications.
"The beauty of ultrasound technology lies not only in its ability to visualize but in its non-invasive nature, allowing examinations without the need for incisions or exposure to harmful radiation."
For a closer look at the scientific principles behind ultrasound technology, further readings can be found on resources like Wikipedia and Britannica.
Piezoelectric Crystals in Medical Imaging
The role of piezoelectric crystals in medical imaging is a critical area that exemplifies the intersection of advanced material science and healthcare technology. These crystals are not just devices but form the backbone of ultrasound systems utilized for diagnostics and therapeutic applications alike. Their ability to convert mechanical vibrations into electrical signals and vice versa makes them indispensable in various imaging modalities.
One of the primary benefits of using piezoelectric materials in medical imaging is their sensitivity and precision. The conversion process allows for the generation of high-quality images from the ultrasound waves reflected off internal structures. This capability aids physicians in accurately identifying conditions without the need for invasive procedures, effectively enhancing patient care.
Ultrasound in Diagnostic Imaging
Ultrasound imaging, largely powered by piezoelectric crystals, is particularly favored because of the safety and non-invasive nature of the procedure. High-frequency sound waves penetrate the body and return echoes, which are interpreted by a computer to produce images. Unlike traditional radiographic methods that use harmful ionizing radiation, ultrasound poses minimal risk to patients.
Key characteristics of ultrasound in diagnostic imaging include its ability to provide real-time visuals and its versatility across various medical fields, such as obstetrics and cardiology. Furthermore, advancements in piezoelectric crystal technology have produced better transducers that generate a higher resolution of images and allow for more detailed examination of soft tissues.
Applications in Therapeutic Ultrasound
Beyond diagnostics, piezoelectric crystals play a significant role in therapeutic ultrasound applications, where they help treat conditions ranging from muscular pain to certain tumors. The technology harnesses focused ultrasound waves to deliver energy deep into the body, offering a targeted treatment option that can minimize damage to surrounding healthy tissues.
Some notable therapeutic applications include high-intensity focused ultrasound (HIFU), which aims to destroy malignant tissues and relieve pain. Additionally, ultrasound is used in physiotherapy to promote tissue healing and improve blood flow, showcasing the broader applications of piezoelectric technology in healthcare.
Comparison with Other Imaging Techniques
When comparing ultrasound technology powered by piezoelectric crystals to other imaging modalities like CT scans, MRIs, and nuclear imaging, several key aspects emerge.
CT Scans
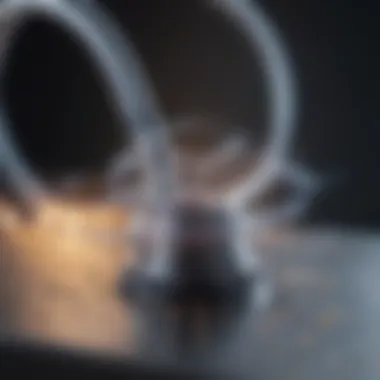
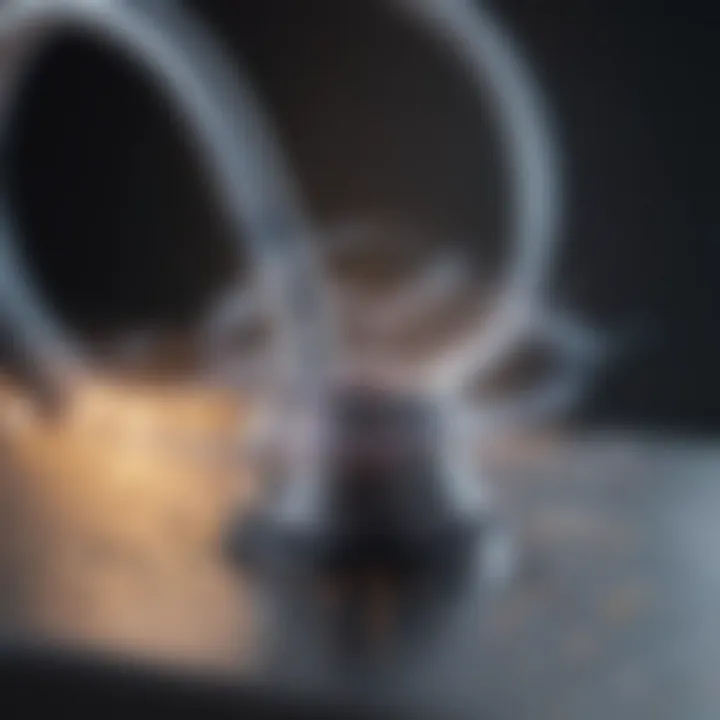
CT scans utilize a series of X-ray images taken from different angles around the body, which a computer reconstructs to create detailed cross-sectional images. A key characteristic is the speed and accuracy of imaging, especially for detecting internal injuries and diagnosing cancers. It is a popular choice due to its quick turnaround time and detailed depiction of bone structures. However, the principal disadvantage is the exposure to radiation, making it less favorable in certain populations, particularly pregnant women.
MRIs
Magnetic Resonance Imaging (MRI) employs strong magnetic fields and radio waves to produce detailed images of internal organs and tissues. One of its notable benefits is the ability to generate high-resolution images of soft tissue, differentiating between various types of tissue clearly. The unique feature of MRIs lies in their non-invasiveness and absence of ionizing radiation. Yet, it has drawbacks, including lengthy scanning times and high costs, which make accessibility an issue for many.
Nuclear Imaging
Nuclear imaging involves the injection of radioactive tracers into the body, which emit radiation detected by gamma cameras. This technique provides functional imaging based on metabolic activity, proving invaluable in oncology and cardiology. The key characteristic is its capability to reveal physiological changes at an early stage. However, it carries inherent risks associated with radiation exposure and is less widely used due to the complexity and costs involved.
In summary, while ultrasound powered by piezoelectric technology offers significant advantages, each imaging modality has its strengths and limitations. Understanding these nuances is essential in making informed decisions in medical diagnostics and treatment.
Industrial Applications of Piezoelectric Ultrasound
The field of industrial applications for piezoelectric ultrasound shines a light on the cutting-edge capabilities of this technology. From manufacturing processes to structural health monitoring, this domain is not just about innovation; it's about enhancing efficiency, precision, and safety across various sectors. Businesses are seeing increasingly complex needs for durability and reliability, making the tools offered by piezoelectric ultrasound indispensable. This section delves into several critical avenues where this technology has made significant impacts.
Non-Destructive Testing
Non-destructive testing (NDT) is a cornerstone of quality assurance in manufacturing. Piezoelectric ultrasound plays a vital role in ensuring that materials and structures meet safety standards without causing damage. By emitting high-frequency sound waves, these systems can detect internal flaws, such as cracks or voids, without interfering with the component's structural integrity.
Key benefits of using piezoelectric ultrasound in NDT include:
- Early Detection: Identifying potential issues before they escalate into catastrophic failures, ultimately saving time and costs.
- Versatility: Suitable for various materials including metals, plastics, and composites.
- Real-Time Analysis: Immediate results from ultrasonic inspections enable quick decision-making.
Organizations in aviation, automotive, and construction industries have adopted piezoelectric technology to augment their inspection processes, pushing the boundaries of reliability and productivity.
Material Characterization Techniques
Material characterization is essential for understanding the properties and behavior of materials under different conditions. Piezoelectric ultrasound provides a practical means to assess these properties without destructive intervention. By analyzing the velocity and attenuation of ultrasonic waves in a material, engineers can gather critical insights into its density, elasticity, and phase changes.
Using ultrasound in this setting can offer:
- Precise Measurements: Understanding material properties with high accuracy ensures that the right materials are used in the right applications.
- Cost-Efficiency: Non-invasive methods reduce the need for costly and time-consuming testing approaches, enhancing overall operational efficiency.
In sectors like electronics, it’s pivotal to characterize materials to avoid failures in performance that could otherwise go unnoticed until it’s too late.
Role in Manufacturing Processes
In manufacturing, piezoelectric ultrasound serves as more than just a quality control tool; it actively influences the production process itself. For example, ultrasonic welding utilizes high-frequency vibrations, enabling materials to bond through friction without external heat. It's a game-changer for industries looking to join dissimilar materials or create fine connections in delicate assemblies.
Important advantages of piezoelectric ultrasound in manufacturing include:
- Reduced Energy Consumption: Compared to traditional welding methods which often require high temperatures, ultrasonic processes consume less energy, aligning with sustainability goals.
- Enhanced Process Control: The ability to monitor and adjust parameters in real-time allows for consistent quality in product output, minimizing waste.
- Broader Applications: Beyond welding, piezoelectric ultrasound is useful in cleaning, cutting, and enhancing chemical processes, showcasing its versatility in the manufacturing ecosystem.
"The integration of piezoelectric ultrasound has shifted the paradigm in how industries approach manufacturing, leading to not only greater efficiency but also innovation in product design."
Advanced Research and Innovations
In the realm of piezoelectric crystal ultrasound, the progress made in advanced research and innovations has been nothing short of transformative. This topic is pivotal not only for understanding the underlying mechanisms of current technologies but also for paving the way for future breakthroughs. Every step taken in this area stands to enhance various applications, from medical diagnostics to industrial testing. These innovations often relate to the development of novel piezoelectric materials and their integration with cutting-edge technologies.
Emerging Piezoelectric Materials
The search for emerging piezoelectric materials is like a treasure hunt. Researchers aim to discover substances that not only exhibit strong piezoelectric effects but also have favorable mechanical and thermal properties. One exciting direction includes the exploration of organic piezoelectric materials and nanostructured composites, which could lead to lighter and more flexible applications. For instance, polymers infused with nanomaterials display impressive piezoelectric responses while being easier to manufacture.
A few noteworthy advancements are:
- Biosourced materials: These include biopolymers and other sustainable options, aiming for greener alternatives that maintain performance.
- Nano-piezoelectric devices: Miniaturization in this field can lead to devices capable of converting mechanical energy into electrical energy in compact applications, from sensors to portable devices.
The advantages of investing in emerging materials are numerous; not only do they offer the potential for enhanced functionality, but they also help in reducing environmental impact. As a result, ongoing research in this area is crucial for realizing the benefits while facing challenges like scalability and cost efficiency.
Integration with Advanced Technologies
Integrating piezoelectric ultrasound with advanced technologies brings a wealth of possibilities. This not only amplifies their applications but also enhances their performance and user experience. Two avenues that stand out in this evolution are the incorporation of Artificial Intelligence and Real-Time Imaging into ultrasound systems. These technologies complement the already profound capabilities of piezoelectric materials, creating synergies that can redefine practices.
Artificial Intelligence
Artificial Intelligence (AI) is revolutionizing several fields, and piezoelectric ultrasound is no exception. One of the specific contributions of AI is in data analysis. The sheer volume of data generated during ultrasound imaging can be overwhelming. AI's ability to process and analyze this data allows for quicker diagnosis and more accurate interpretations. Furthermore, AI systems can learn from previous cases, improving their diagnostic capabilities over time.
Key characteristics of AI highlight its value:
- Adaptability: AI can adjust algorithms based on real-time feedback or newly available studies, ensuring that practices remain cutting-edge.
- Efficiency: By automating certain processes, resources can be allocated more effectively, thereby lowering operational costs.
Yet, while AI's integration presents numerous advantages, it isn't without its challenges. Concerns about data privacy, algorithm bias, and the need for transparent processes remain pressing issues. These factors warrant thorough consideration when implementing AI systems in piezoelectric ultrasound technologies.
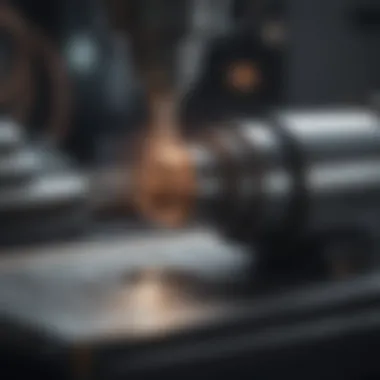
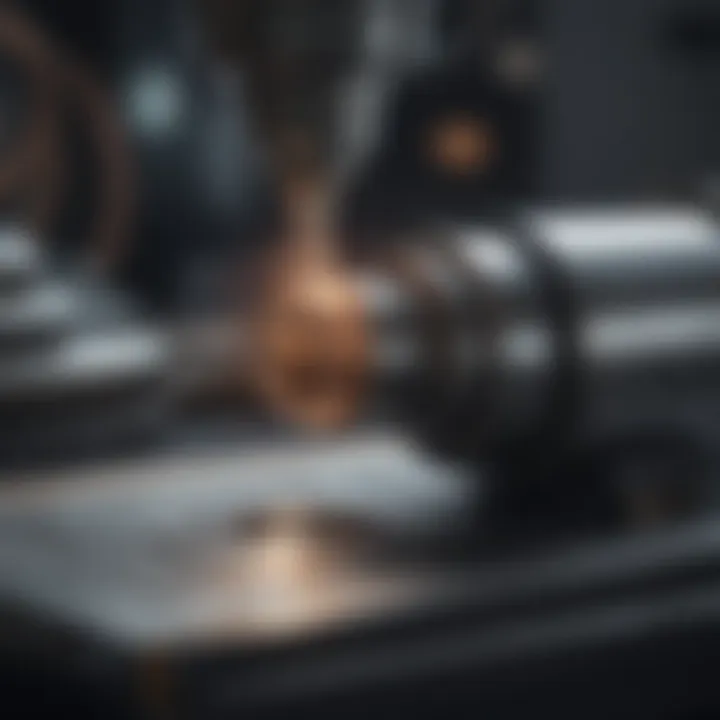
Real-Time Imaging
The capability for real-time imaging is another significant advancement that complements piezoelectric ultrasound technology. This feature allows operators to view and interpret images as they are generated, leading to immediate feedback during procedures. For example, in medical settings, real-time imaging can facilitate prompt decisions in surgical environments.
The benefits of this approach stem from its core attributes:
- Instantaneous feedback: It enables healthcare professionals to assess conditions on-the-spot, enhancing the precision of interventions.
- Interactivity: As most people might wish, the ability to engage with the imaging process allows physicians and patients alike to have insight into the diagnostics in progress.
Nevertheless, achieving seamless real-time imaging poses challenges, such as the need for high-quality equipment and potential delays in data processing. Addressing these challenges is vital to fully exploit this powerful capability.
In summation, the journey of piezoelectric crystal ultrasound through advanced research and innovations illustrates a dynamic landscape rich with potential. As studies continue, the fusion of emerging materials and advanced technologies is likely to pave the way for enhanced capabilities that can significantly impact numerous fields, especially medical and industrial applications.
Ongoing dedication to this research is crucial, as the sky is the limit for what can be achieved when traditional methodologies meet innovation.
Challenges and Limitations
Importance of Challenges and Limitations
In any field of study or application, addressing challenges and limitations is crucial to progressing knowledge and technology. In the realm of piezoelectric crystal ultrasound, this discussion assumes particular significance given the potential impact of these drawbacks on both efficacy and wider adoption. Understanding these limitations ensures that researchers, engineers, and practitioners can strategize effectively to mitigate issues, enhance performance, and maximize the benefits of this technology.
Material Limitations and Performance Issues
Upon examining the performance of piezoelectric materials, several key hurdles emerge. Material fatigue is often cited as a critical concern. Over time, repeated stress on piezoelectric crystals can lead to a gradual degradation of their functionality. This fatigue limits their lifespan and ability to provide consistent results, particularly in clinical settings where reliability is paramount.
Further complicating matters, not all piezoelectric materials respond uniformly to the same stimuli. For instance, natural crystals like quartz exhibit unique properties but may lack the robustness required for high-demand applications. Conversely, synthetic materials such as Lead Zirconate Titanate (PZT) offer greater performance levels but come with drawbacks of their own, including environmental and health concerns.
This brings us to thermal stability. Certain piezoelectric crystals can experience shifts in performance at different temperatures, which affects their efficacy in varying environmental conditions. Ideally, enhancing the thermal stability of these materials can alleviate some of these limitations, leading to improved durability and adaptability in real-world applications.
Regulatory and Safety Considerations
As piezoelectric ultrasound technology continues to evolve, complying with regulatory frameworks is non-negotiable. Standards must be met to ensure that innovations do not compromise user safety or environmental health. Testing protocols and certifications are vital—as any breaches can lead to significant legal and financial repercussions.
From a safety perspective, potential toxicity of certain piezoelectric materials should be scrutinized. For example, the use of lead in PZT raises red flags. The challenge lies in balancing high performance with safe, environmentally-friendly alternatives. Emerging solutions, such as lead-free ceramics, present a promising path, but the transition requires thorough testing to validate both their efficacy and safety.
In addition, the medical field—which most widely employs this technology—must navigate a labyrinth of regulatory standards established by organizations like the FDA. These regulations dictate the parameters for clinical use and necessitate extensive clinical trials to ensure that the technology is not only effective but also safe for human application.
Overall, delving into material limitations and regulatory concerns reveals a landscape that is both challenging and ripe for innovative solutions. Recognizing the boundaries of existing technology will ultimately enable the development of improved piezoelectric materials and more robust applications, paving the way for advancements that can reshape industries.
Future Directions in Piezoelectric Ultrasound Research
The realm of piezoelectric crystal ultrasound is vast, with a horizon brimming with potential advancements and applications. As the demand for more sophisticated and efficient technologies surges, understanding the future directions in piezoelectric ultrasound research becomes imperative. By exploring emerging applications and fostering interdisciplinary collaborations, the field can witness transformative changes.
Innovative Applications in Emerging Fields
As this technology evolves, its applications extend beyond conventional boundaries. For instance, in the realm of environmental monitoring, piezoelectric ultrasound could facilitate real-time assessments of water bodies, allowing for detection of pollutants at minute concentrations. There’s also potential in the area of nanotechnology; integrating piezoelectric materials with micro-scale devices may lead to novel diagnostic tools that operate efficiently at a cellular level.
Some notable innovative applications include:
- Piezoelectric Sensors in Wearable Technology: Monitoring health parameters like heart rate and muscle activity with a high degree of sensitivity.
- Precision Agriculture: Utilizing ultrasound to analyze soil conditions or crop health, optimizing resource usage, and minimizing adverse environmental effects.
- Telecommunications: Piezoelectric ultrasound helping to enhance signal transmission, leading towards more robust communication networks.
- Acoustic Imaging in Art Conservation: Non-invasive techniques using ultrasound can aid in evaluating the condition of artworks, ensuring their preservation.
These applications illustrate that the adaptability of piezoelectric technology can lead to significant advancements, addressing various societal challenges in a myriad of sectors.
Interdisciplinary Collaborations and Insights
Successful research often doesn’t exist in a vacuum. The future of piezoelectric ultrasound is deeply intertwined with collaboration across different fields. Bringing together expertise from material science, engineering, biology, and environmental science opens doors to fresh perspectives and innovative solutions.
For instance, engineers and materials scientists can team up to develop new piezoelectric materials with enhanced properties, such as increased sensitivity or lower energy consumption. Meanwhile, collaboration with medical professionals can yield advanced diagnostic technologies that bridge the gap between theoretical research and clinical application.
"Modern problems require modern solutions. Far more will be achieved with a multifaceted approach to piezoelectric crystal technology.”
Furthermore, academic institutions can work alongside industries to translate theoretical research into practical applications, ensuring that innovations in piezoelectric ultrasound can be swiftly adapted for real-world use. Initiatives like joint research projects, workshops, and symposiums can foster networking opportunities, resulting in breakthroughs that benefit numerous communities.
Culmination
The conclusion serves as the bedrock that connects all the threads woven throughout this exploration of piezoelectric crystal ultrasound. Understanding this field is not just about grasping the mechanics—it cuts to the heart of numerous advancements across various sectors, particularly in health and engineering.
Recapitulating Key Insights on Piezoelectric Ultrasound
In essence, piezoelectric ultrasound technologies have proven to be a game-changer in several domains. Their capability to convert mechanical energy into electrical signals, and vice versa, has facilitated remarkable innovations. For instance, in medical imaging, the precision of ultrasound scans depends heavily on the properties of piezoelectric materials. These crystals, like quartz and Lead Zirconate Titanate, are pivotal in generating high-frequency sound waves that create detailed images of the human body.
Moreover, in the industrial realm, non-destructive testing through piezoelectric ultrasound guarantees the integrity of materials in manufacturing processes. Whether it’s inspecting welds or characterizing new materials, this technology showcases unparalleled efficacy. The adaptability of piezoelectric applications demonstrates that they are more than just a scientific curiosity—they are a fundamental part of modern technological practices.
"Piezoelectric crystals not only revolutionize imaging techniques but also redefine the standards of precision and reliability in various applications."
The Path Ahead for Research and Development
Looking to the future, the potential for piezoelectric ultrasound technology appears boundless. Researchers are on the lookout for novel materials that promise better efficiency and functionality. There’s a growing interest in integrating artificial intelligence with ultrasound technologies to revolutionize real-time imaging capabilities. The collaboration between different fields like engineering, medicine, and computer science can lead to groundbreaking innovations.
It's essential, however, to navigate the bumps on this road. Addressing the existing limitations—such as the durability of materials and regulatory frameworks—will demand attention. Nevertheless, interdisciplinary collaborations can provide the insights needed to overcome these hurdles. Exploring what's ahead, one can envision advanced ultrasound systems that can not only diagnose but also predict health issues, offering proactive care that can save lives.
In wrapping up, piezoelectric ultrasound stands at a fascinating crossroads of technology and invention. Future research endeavors are set to broaden the horizons even further, making this an exciting area for students, researchers, and professionals alike to watch closely.