Exploring Molecular Weight Distribution in Polymers
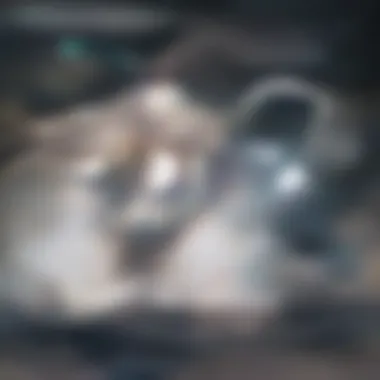
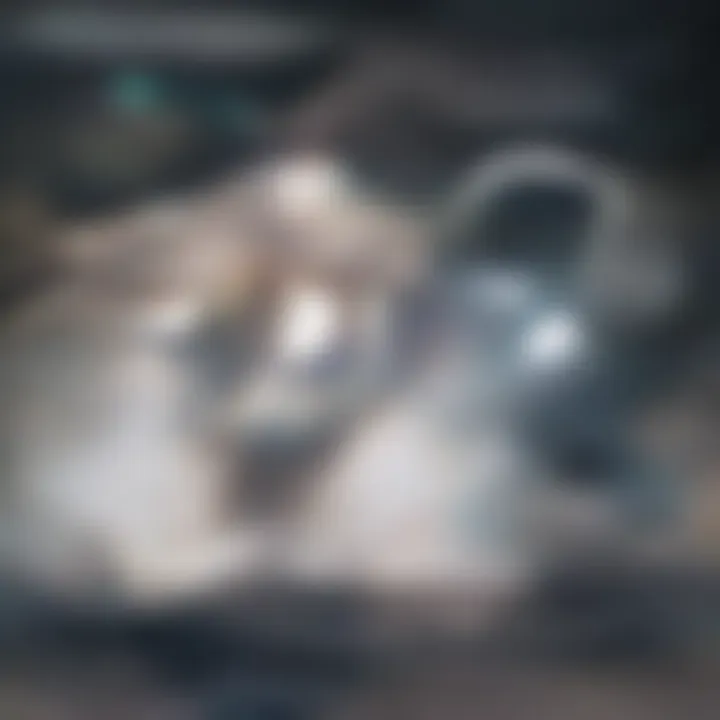
Intro
Molecular weight distribution (MWD) stands as a pivotal aspect in the field of polymer science. Understanding MWD is essential for comprehending how molecular characteristics influence the behavior of polymeric materials. These characteristics dictate the physical and mechanical properties such as viscosity, tensile strength, and thermal stability. Moreover, the MWD profoundly affects how polymers are processed and the applications they can be utilized in, ranging from everyday products to sophisticated nanotechnology solutions.
This section aims to provide a fundamental overview of the importance of MWD, its measurement, and the consequences for the performance of polymers in various settings.
Key Findings
Major Results
- MWD significantly influences mechanical properties and processing behavior in polymers.
- Techniques for measuring MWD include Size Exclusion Chromatography (SEC) and Gel Permeation Chromatography (GPC).
- Variability in MWD can lead to different product performance characteristics, impacting everything from durability to elasticity.
Discussion of Findings
The findings emphasize that MWD is not merely a numerical value but a reflection of the polymer's behavior in real-world applications. For example, a polymer with a narrow MWD may demonstrate enhanced mechanical performance due to more uniform chain lengths, whereas wide MWD can increase variability, enabling advantageous features in specific applications. Additionally, understanding MWD assists in predicting the performance of polymers in different environments, crucial for product development.
Methodology
Research Design
The exploration of MWD is conducted through various experimental frameworks, allowing for the careful measurement and analysis of molecular characteristics. Each method provides distinct insights into how molecular weight affects polymer diversity.
Data Collection Methods
Data is primarily collected using techniques like SEC and GPC. These methods involve passing polymer samples through a column to separate them based on size. This results in a more thorough understanding of not only the average molecular weight but also the full distribution of molecular weights present, which is fundamental for comprehending overall material behavior.
"Understanding MWD is indispensable in polymer science; it lays the foundation for the entire study of polymer functionality and applicability."
By engaging with the principles and techniques outlined, students, researchers, and practitioners can grasp the complexities of MWD in relating theoretical concepts to practical applications, enriching their knowledge and advancing their work in the field.
Preface to Polymer Molecular Weight Distribution
In the realm of polymer science, molecular weight distribution (MWD) serves as a foundational concept. Understanding MWD is essential as it significantly influences the properties and behavior of polymeric materials. Various characteristics such as mechanical strength, thermal stability, and processing behavior are heavily dictated by MWD. Therefore, mastering this concept can empower researchers and practitioners alike when designing materials for specific applications.
Molecular weight, in general, refers to the mass of a given molecule, typically expressed in daltons or grams per mole. However, polymers are not uniform; they consist of many molecules with different weights. This variability leads to MWD, a key parameter that reflects the range of molecular weights present within a sample. Analyzing MWD allows for a deeper insight into the physical and chemical properties of polymers, making it vital to material selection and processing strategies.
Understanding the MWD of a polymer can provide essential insights into its market applications. For instance, in industries such as automotive or aerospace, precise control over MWD can enhance product reliability and functionality. In addition, advancements in technology allow for innovative applications of polymers, thereby increasing their importance in fields ranging from healthcare to electronics. As a result, understanding MWD has implications that transcend traditional materials science.
In this article, we will cover the definition and significance of MWD, provide historical context to enhance comprehension, and explore various methodologies for measuring it.
"Molecular weight distribution is not just a number; it embodies the distinct characteristics and potential of polymeric materials."
Engaging with the intricacies of MWD is therefore integral for anyone involved in polymer science, making this investigation essential for both new learners and veteran researchers alike.
Theoretical Framework of Molecular Weight Distribution
Understanding the theoretical framework of molecular weight distribution (MWD) is essential for comprehending the structure and functionality of polymers. MWD plays a critical role in defining the properties of polymer materials. The distribution gives insight into how molecular weight impacts behavior in applications. Different averages provide a range of perspectives on polymer characteristics, influencing decisions in material selection, processing, and application.
Concept of Average Molecular Weight
Average molecular weight is a key concept in polymer science. It allows for a simplified yet effective way to characterize complex polymer mixtures. The most common measures are the Number Average Molecular Weight, Weight Average Molecular Weight, and Z-Average Molecular Weight. Each provides unique insights into the distribution of molecular sizes within a polymer sample.
Number Average Molecular Weight
Number average molecular weight focuses on the total number of polymer molecules. The approach calculates an average that represents all molecules equally. This characteristic makes it a straightforward and widely usable metric.
One significant advantage of this method is its intuitive nature. It makes it simple to understand the overall size of the polymer. However, there are limitations. Number average can be skewed by the presence of larger molecules, which may not represent the common size of the polymer very well.
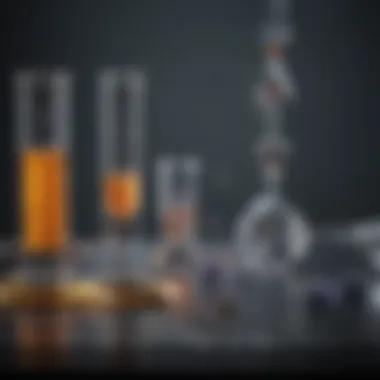
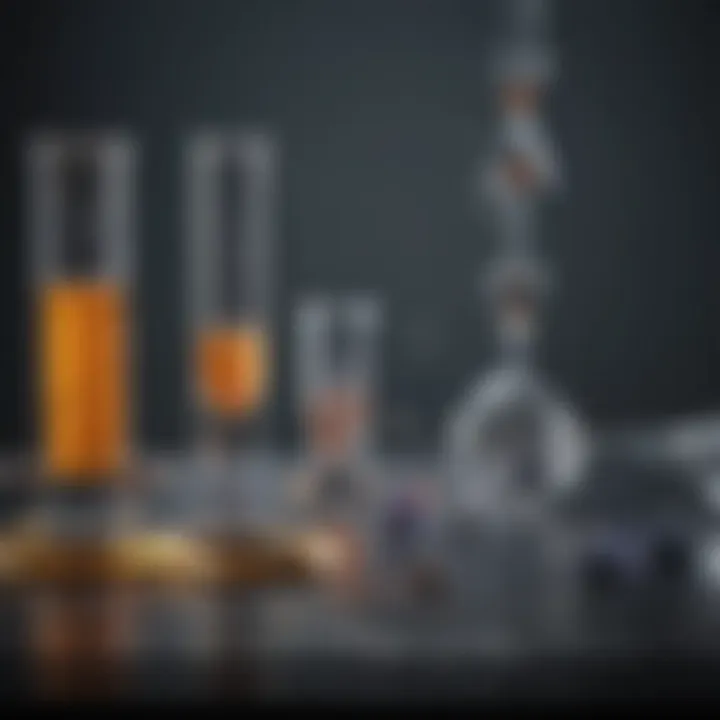
Weight Average Molecular Weight
Weight average molecular weight, in contrast, considers both the size and number of molecules. Larger molecules have a more substantial impact on the overall average. This feature is beneficial when comparing materials where larger molecules influence performance characteristics.
This method is popular for its ability to provide a more accurate representation of polymer behavior under stress. However, it can lead to more complex calculations and interpretations, particularly in mixtures with significant molecular weight variation.
Z-Average Molecular Weight
Z-Average molecular weight accounts for the influence of high molecular weights on the average. It brings forth a balance between the number and weight average considerations. This measure reflects the overall polymer behavior in specific applications, particularly in rheological contexts.
This average is useful in scenarios where high molecular weight components drive performance. On the downside, this method can be less commonly used, resulting in less familiarity among practitioners and researchers.
Distribution Functions in Polymers
In addition to average molecular weight, distribution functions help in understanding the range of molecular sizes present in polymer samples. This understanding is vital since properties like elasticity, melting point, and tensile strength depend fundamentally on how molecular weight is distributed within the polymer.
Gaussian Distribution
Gaussian distribution is a common model assumed in many polymer systems. It reflects a symmetrical distribution of molecular weights around an average. This characteristic makes Gaussian suitable for a variety of materials. However, not all polymers necessarily fit this model. When used, care must be taken as real-world data may present asymmetries that the Gaussian model cannot accommodate.
Exponential Distribution
Exponential distribution presents a different view. It can describe systems where lower molecular weights are more common than higher ones. This model can be reflected in certain types of synthetic polymers. Its usage can help highlight the presence of a significant number of lower molecular weight chains, yet it may fail in systems with a broader range of sizes.
Log-Normal Distribution
Log-normal distribution provides another perspective, especially when dealing with polymers that exhibit a wide range of molecular weights. It captures situations where the logarithm of molecular weight follows a normal distribution. This is beneficial for analyzing certain biopolymers, as it provides a realistic representation of their complex nature. Nonetheless, this model can add complexity in terms of interpretation and analysis.
Methods of Measuring Molecular Weight Distribution
Understanding the molecular weight distribution (MWD) of polymers is critical to the performance and application of materials in various fields. The methods used to measure MWD play a foundational role in determining a polymerβs characteristics, which are essential for its functional applications. Accurate assessment of MWD affects physical, mechanical, and thermal properties, thereby influencing material selection and processing techniques. An in-depth comprehension of these measurement techniques can lead to optimization in product development and innovation.
Gel Permeation Chromatography (GPC)
Principles of GPC
Gel permeation chromatography is a popular method for measuring the MWD of polymers. It relies on a porous gel stationary phase that allows polymer molecules to separate based on their size. Large molecules flow through the column more quickly because they encounter fewer pores, whereas smaller molecules take longer as they can enter the pores. This size-based separation enables GPC to characterize polymer samples effectively.
GPC is favored for its reliability and precision. One of the unique features is its ability to provide a detailed distribution profile that reflects the heterogeneity of polymer samples. However, it does require careful calibration and sample preparation to ensure accurate results, which can be seen as a disadvantage by some.
Calibration Procedures
Calibration in GPC is essential for producing accurate molecular weight determinations. Calibration involves running standards with known molecular weights through the GPC system to create a calibration curve. This procedure ensures that the data obtained from samples can be converted into meaningful molecular weight information. It is crucial for validating the system and achieving reproducibility in measurements.
One key aspect of calibration is selecting appropriate standards that closely match the properties of the samples being measured. Typically, polystyrene standards are used, but this can limit the method if the sample's behavior deviates from that of these standards. This is a known consideration that researchers must account for.
Interpretation of Results
The interpretation of results from GPC is critical for understanding a polymer's characteristics. Data are typically presented as molecular weight distribution curves. These curves depict the relative abundance of different molecular weights within a sample. The position and shape of the curve provide insights into the polymer's MWD, indicating if the sample has a narrow or broad distribution.
A benefit of GPC is its clarity in presenting the molecular weight distribution visually, allowing for straightforward comparisons between different samples. Yet, careful analysis is vital, as misinterpretation can lead to erroneous conclusions about a polymer's properties. Understanding how to read these curves accurately is an essential skill for those working in polymer science.
Mass Spectrometry Techniques
Matrix-Assisted Laser Desorption Ionization (MALDI)
Matrix-assisted laser desorption ionization is an advanced mass spectrometry technique that provides precise molecular weight information for polymers. This method uses a laser to ionize polymer samples embedded within a matrix, allowing for the analysis of large, complex molecules. The reliability and effectiveness of MALDI make it a valuable tool, especially for large biopolymers.
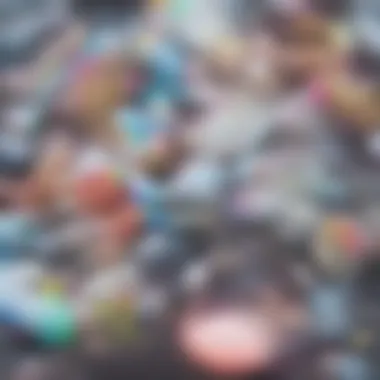
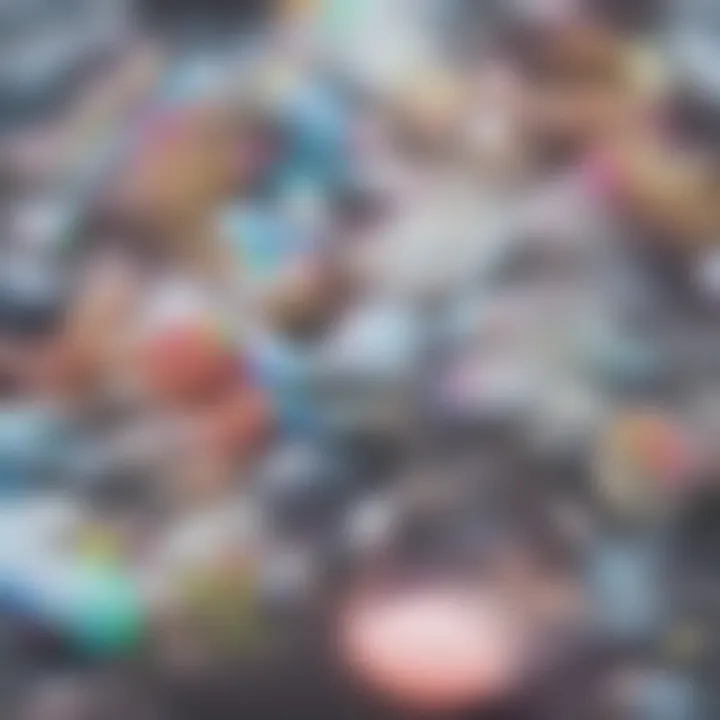
A significant advantage of MALDI is its ability to analyze high molecular weight compounds without extensive fragmentation. However, the need for a suitable matrix can introduce variability, making careful selection essential to achieve optimal results.
Electrospray Ionization (ESI)
Electrospray ionization is another technique used for measuring the molecular weights of polymers. This method involves applying a high voltage to a solution to produce charged droplets. As these droplets evaporate, they release ions that can be analyzed by mass spectrometry. ESI is particularly beneficial for analyzing polymers in solution and is capable of providing real-time data.
One strength of ESI is its compatibility with a wide range of solvents and its ability to assess multiple samples quickly. Nevertheless, it may face challenges with very high molecular weight polymers, as they can fragment during the process, complicating the interpretation of data.
Advantages and Limitations
These mass spectrometry techniques each possess distinct advantages and limitations. While MALDI offers structural information and less fragmentation, ESI provides speed and versatility. However, both methods require skilled operators and careful sample preparation to avoid inaccuracies in results. Ultimately, understanding these factors helps to determine the most suitable method for specific applications in polymer research.
Static and Dynamic Light Scattering
Static and dynamic light scattering techniques are also useful for measuring MWD. These methods analyze the scattering of light by molecules in solution, allowing researchers to determine size distributions and molecular weights accurately.
The uniqueness of these techniques lies in their ability to analyze samples without the need for extensive sample preparation, which can be advantageous in many scenarios. However, they still require sophisticated equipment and interpretation skills.
Overall, each method for measuring molecular weight distribution brings its strengths and weaknesses. The choice of technique can significantly affect the outcome of a study, underscoring the importance of understanding these methods in the context of polymer analysis.
Influence of MWD on Polymer Properties
Molecular Weight Distribution (MWD) plays a significant role in determining how a polymer will perform in various applications. Understanding this distribution is crucial for researchers and manufacturers alike, as it affects not only mechanical characteristics but also thermal behaviors and processing capabilities. MWD gives insight into the average molecular weight and the variation within a sample, which in turn influences properties like strength, elasticity, and durability. The implications of MWD go beyond theoretical knowledge; they have tangible effects on material selection and product performance.
Mechanical Properties
Tensile Strength
Tensile Strength refers to the maximum amount of tensile (stretching) stress that a material can withstand before failure. In polymers, tensile strength is essential because it dictates how a material will perform under load. Higher tensile strength generally indicates better performance for applications requiring durability and resistance to deformation. A polymer with a well-defined MWD can achieve specific tensile strength targets, making it an attractive choice for various products. However, achieving a balance in MWD is key, as too narrow of a distribution can lead to weak points due to a lack of structural diversity in the polymer chains.
Elasticity and Flexibility
Elasticity and flexibility are critical attributes for numerous applications, including packaging and automotive parts. A polymer's ability to return to its original shape after deformation is heavily influenced by its MWD. Polymers with a broad MWD often display improved elasticity, as the presence of different chain lengths facilitates energy dissipation. This versatility can enhance user experience through improved product performance. However, if the distribution is too wide, it might lead to inconsistent performance, which could be a disadvantage in precision applications.
Impact Resistance
Impact Resistance measures a material's ability to absorb energy during an impact without failing. This property is particularly relevant in fields such as construction and automotive industries, where durability is paramount. Polymers with optimized MWD are often tailored for higher impact resistance by balancing different molecular weights within the structure. A well-managed MWD allows for the incorporation of both short and long chains, enhancing the material's ability to disperse energy effectively. Conversely, if the MWD is overly concentrated around a narrow molecular weight, the material may shatter under stress, leading to failure in critical applications.
Thermal Properties
Glass Transition Temperature (Tg)
Glass Transition Temperature (Tg) is the temperature range in which a polymer transitions from a brittle glassy state to a more rubbery state. The Tg is influenced by the polymer's MWD, as variations in chain lengths can change the mobility of the chains. Polymers with higher MWD can exhibit altered Tg values, allowing for tailored thermal behaviors suited for specific applications. A polymer with precisely controlled MWD may enable better performance in higher temperature applications, but too broad a distribution may cause variations in properties, possibly complicating processing.
Melting Temperature (Tm)
Melting Temperature (Tm) is another critical parameter, especially in crystalline polymers. Tm impacts how a polymer behaves at elevated temperatures. MWD affects the Tm value, where variations can lead to a range of melting behavior across a polymer sample. Polymers with a narrow MWD often show distinct melting behavior, while those with a broader distribution may result in a less defined melting point. This can be advantageous for processes such as extrusion or injection molding, as the flowing behavior is affected by these thermal properties. However, a wide MWD can also result in difficulties during processing due to inconsistent melting behavior.
Processing Characteristics
Flow Behavior
Flow Behavior is a key factor in the processing of polymers. It determines how a polymer sample moves and deforms under applied stress during processing operations like injection molding and extrusion. A well-characterized MWD can provide insights into flow behavior, significantly impacting the efficiency of processing techniques. For example, polymers with a lower viscosity due to a suitable MWD can improve flow characteristics, making them easier to process. However, if MWD is not managed properly, it can lead to poor flow, resulting in defects in the final product.
Viscosity Considerations
Viscosity plays an integral role in the processing of polymers, dictating how a polymer melts and flows during production. It is critically affected by the distribution of molecular weights present. High MWD can lead to increased viscosity, which can complicate processing, while a lower MWD often allows for smoother processing conditions. Thus, selecting the right MWD is essential for achieving desired viscosity levels tailored to specific applications. Balancing these considerations is necessary to ensure optimal outcomes in production and final product performance.
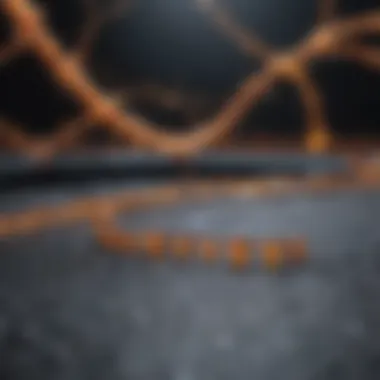
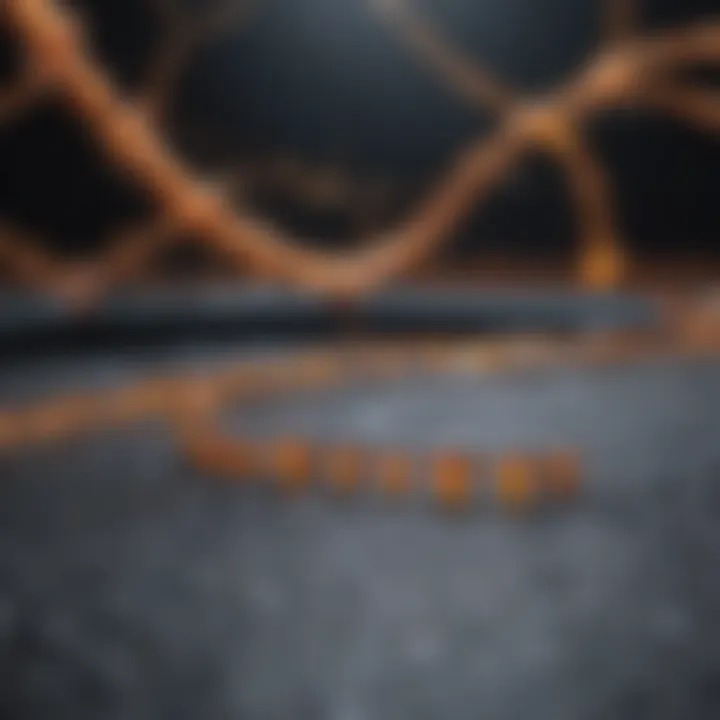
Applications of MWD Understanding
Molecular weight distribution (MWD) plays a pivotal role in various real-world applications. The significance of understanding MWD transcends beyond academic curiosity. It directly informs material selection, guides researchers in the development of biopolymers, and influences advancements in nanotechnology. A comprehensive understanding of MWD allows for tailored properties of polymers, which are essential in optimizing their performance in specific applications.
Impact on Material Selection
When selecting materials for a particular use, MWD can be the deciding factor. Different applications may require specific mechanical, thermal, or processing characteristics. Thus, the variation in molecular weight can lead to a profound impact on how materials behave under stress or temperature changes.
- Mechanical Properties: High-molecular-weight polymers may exhibit improved strength and durability, making them suitable for structural applications. Conversely, low-molecular-weight materials may be preferred for applications where flexibility and processing ease are crucial.
- Thermal Stability: Materials intended for high-temperature applications usually rely on polymers with well-defined MWD to ensure that thermal properties align with functional requirements.
- Processing Methods: Variations in MWD will dictate the processing techniques employed. For instance, materials with a narrow MWD can be easier to process, leading to higher quality products.
In making informed choices, engineers and scientists must analyze the MWD of potential materials to ensure optimal performance and longevity of the products being developed.
MWD in Biopolymers
In the realm of biopolymers, understanding MWD is critical for designing materials that meet biological performance requirements. Biopolymers differ significantly in their structure and functionality compared to synthetic polymers. Thus, MWD influences not just physical properties but also biological interactions.
- Compatibility with Biological Systems: Tailoring the MWD can enhance the compatibility of biopolymers with living organisms, making them suitable for applications in drug delivery and tissue engineering.
- Degradation Rates: In targeted applications, the degradation characteristics of biopolymers play a crucial role. Optimizing MWD can lead to necessary degradation rates, which are vital for ensuring that the polymer performs as desired in specific medical applications.
Thus, for biopolymers to be effectively utilized in medical fields, researchers must have a robust understanding of their MWD characteristics.
Significance in Nanotechnology
In the rapidly evolving field of nanotechnology, MWD understanding is increasingly becoming essential. The performance and properties of nano-sized polymeric materials are intimately linked to their molecular weight distribution.
- Control over Nanostructures: Achieving desired specifications in nanomaterials often requires precise control over MWD. For example, the formation and stability of nanoparticles depend on the polymerβs molecular weight.
- Enhanced Functional Properties: Polymers with tailored MWD can lead to materials with unique electrical, thermal, and mechanical properties. Engineers strive to engineer these materials for applications in sensors, coatings, and energy storage devices.
Understanding MWD can thus facilitate innovations in nanotechnology that are both efficient and effective. As scientists continue to explore the capabilities of polymers, MWD will remain a cornerstone for advancements.
The knowledge of molecular weight distribution will determine the future direction of polymer research and its numerous applications across industries.
Future Directions in MWD Research
The realm of molecular weight distribution (MWD) research is in constant evolution. New insights into polymer characteristics and behaviors accompany the advancement of technology and analytical techniques. Understanding these future directions is vital for researchers and practitioners alike, as they hold the potential to unlock new applications and enhance existing processes.
One significant area of focus is the emergence of advanced characterization techniques. Methods that can provide more precise and comprehensive insights into MWD are needed to address the complexities of modern polymer science. As materials are developed for increasingly specialized uses, measuring the molecular distribution with high accuracy becomes crucial.
Another important aspect is the integration of machine learning into MWD analysis. Using algorithms and data-driven approaches can significantly increase the efficiency and accuracy of MWD measurements. By processing large datasets, machine learning can help identify patterns in molecular behavior that traditional methods may overlook. This intersection of polymer science and computational technology promises to enhance understanding in ways previously unimagined.
Emerging Characterization Techniques
Emerging characterization techniques aim to improve the resolution and reliability of MWD analysis. Some of the innovative approaches currently under investigation include:
- Multi-Angle Light Scattering (MALS): This method can determine absolute molecular weight without the need for calibration. It provides real-time data on molecular conformation and size.
- Novel Chromatography Methods: Techniques such as High-Performance Liquid Chromatography (HPLC) have developed further, integrating new stationary phases for enhanced separation of polymer chains.
- NMR Spectroscopy: Nuclear Magnetic Resonance offers insights into molecular structure that can correlate to MWD, enabling researchers to deduce distributions indirectly.
These advanced techniques offer more detail regarding the polymer structure, allowing for advanced applications in material science and engineering fields. By incorporating multiple analytical techniques, a more robust view of MWD can be constructed.
Integrating Machine Learning in MWD Analysis
Integrating machine learning in MWD analysis presents a paradigm shift in how polymer scientists can interpret data. As machine learning algorithms can process vast amounts of data, they offer various advantages:
- Pattern Recognition: Machine learning can analyze patterns in MWD data that may not be apparent through traditional analysis, allowing for deeper insights into molecular behavior.
- Predictive Modeling: Algorithms can be trained with existing data to predict the outcomes of new polymer formulations. This can lead to more efficient design processes, where properties are estimated before actual synthesis.
- Automation: Many machine learning solutions can work in real-time, automating the analysis process and minimizing human error.
In summary, the future directions in MWD research are promising and multifaceted. Emerging characterization techniques and the integration of machine learning present opportunities for deeper insights and more efficient workflows. Investment in these areas will ultimately lead to advancements in polymer applications across various industries, making the study of MWD increasingly integral to the field of polymer science.
The continual advancements in MWD techniques and analysis underscore the importance of ongoing research and collaboration in the field.
Closure
The conclusion section emphasizes the critical role that molecular weight distribution (MWD) plays in polymer science. Understanding MWD is essential for those involved in polymer research, development, and application. Its significance extends beyond mere measurement; it influences the physical behaviors and overall performance of polymeric materials.
Importance of MWD in Polymer Science
- Fundamental Insights
MWD provides insights into the characteristics of polymers. By analyzing how molecular weight varies within a sample, researchers can predict how a polymer will behave under various conditions. This is crucial in applications that require specific mechanical properties or thermal stability. - Quality Control
Accurate MWD measurements are vital for quality assurance in production processes. Consistent molecular weight distribution ensures that the polymers produced meet industry standards and perform reliably in their intended applications. - Material Performance
The performance of polymers is intimately linked to their MWD. Variations in molecular weight can significantly affect properties such as tensile strength, elasticity, and processability. Thus, understanding MWD allows for the fine-tuning of polymer formulations to achieve desired performance attributes. - Innovation in Applications
A deep understanding of MWD fosters innovation. As polymer technology evolves, new applications in fields such as biopolymers and nanotechnology emerge. Knowledge of how MWD impacts polymer behavior can guide researchers in developing advanced materials with tailored properties. - Interdisciplinary Relevance
The implications of MWD extend into various disciplines, including materials science, mechanical engineering, and environmental science. This interdisciplinary relevance makes MWD a crucial topic for researchers and professionals across multiple domains, highlighting its universal importance.
In summary, the conclusion reiterates that mastery of MWD is not just a technical requirement but a strategic advantage in polymer science. Understanding the intricate relationship between molecular weight and polymer properties enhances the ability to innovate, optimize processes, and ensure the quality of products in the polymer industry. By comprehensively exploring MWD, this article equips researchers, educators, and practitioners with the knowledge needed to excel in their respective fields.