Exploring Liquid Scintillation: Principles and Innovations
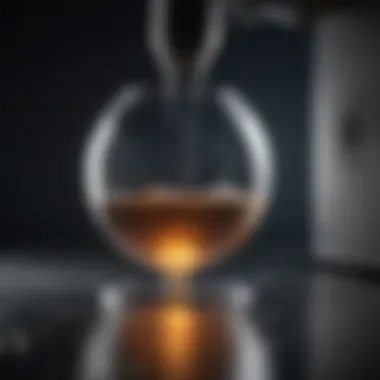
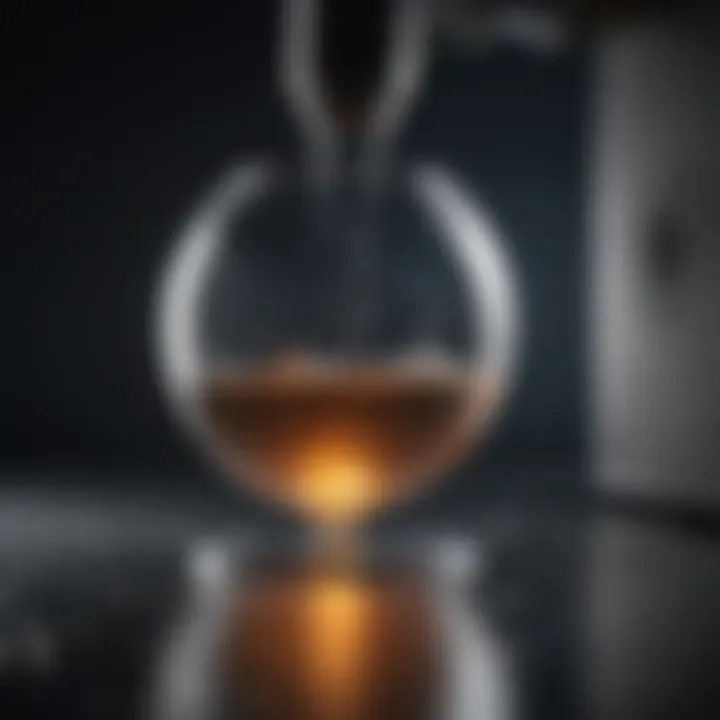
Intro
Liquid scintillation is an intriguing technique that holds a key position in the field of radiation detection. It’s not just about spotting radiation; it’s about understanding the core principles and applications that this method offers. As we delve into this subject, we link together various scientific aspects and explore how advancements in this technology can make a real impact.
The world of liquid scintillation is underpinned by fascinating physical and chemical principles. These principles guide the visibility of ionizing radiation by transforming it into visible light. Imagine a world where you can see the subtle movements of radiation; that’s what liquid scintillation makes possible.
In this exploration, we will cover essential findings, methodologies, and implications associated with liquid scintillation. By breaking down complex concepts into digestible pieces, we aim to create a narrative that is not only informative but also engaging for a diverse audience, including students, researchers, educators, and professionals.
Let’s embark on this journey to understand how liquid scintillation operates and the variety of fields in which it finds practical application, such as environmental monitoring, nuclear medicine, and radiobiology. This synthesis aims to equip you with a comprehensive understanding of liquid scintillation and its significance in modern science.
Foreword to Liquid Scintillation
Liquid scintillation plays a crucial role in the realm of radiation detection. This technique uses specific materials and processes to capture the energy released when ionizing radiation interacts with matter. As we explore its intricacies, we uncover its significance not just in scientific research but also in practical applications that affect our world today. From environmental monitoring to advancements in medical technologies, liquid scintillation's influence is widespread and vital.
Historical Overview
The origins of liquid scintillation date back to the mid-20th century when scientists began to seek more effective methods to identify and quantify ionizing radiation. Initial endeavors in this area were rudimentary at best, relying heavily on solid scintillators and simpler detection devices.
Innovations came with the introduction of organic solvents in the 1940s, which sparked a revolution in how radiation could be measured. Notably, the work of scientists like George W. Beadle and others laid the groundwork for the blending of liquid materials and detection systems. It wasn't long before liquid scintillation counting emerged as a preferred technique, blending efficiency with precision in ways that were previously thought impossible.
Definition and Basic Concepts
In essence, liquid scintillation involves a clear, often colorless liquid that contains scintillating materials. When ionizing radiation passes through this liquid, it interacts with the molecules, leading to the emission of brief flashes of light. This light is then quantified, offering a measure of the radiation that has passed through.
Key Concepts in Liquid Scintillation:
- Scintillator: The substance that emits light when excited by radiation.
- Photomultiplier Tube (PMT): The device that detects the scintillation light and amplifies it into an electrical signal.
- Quenching: A phenomenon where non-scintillator materials suppress the light emission, affecting measurements.
Understanding these foundational aspects sets the stage for diving deeper into how liquid scintillation is utilized in various fields, the advancements that have been made, and the challenges that remain.
Fundamental Principles of Scintillation
Understanding the fundamental principles of scintillation is essential for a thorough grasp of how this technique is utilized in a range of scientific applications. By studying these principles, researchers can better design experiments, troubleshoot issues, and ultimately improve detection accuracy.
At its core, scintillation is the process whereby certain materials emit flashes of light when they are exposed to ionizing radiation. This intrinsic property forms the basis of many detection techniques. The efficiency of light production in scintillation materials can significantly affect the sensitivity of measurements, making these principles not just foundational but also critical for practical implementations in research and industry.
Mechanism of Scintillation
Scintillation occurs due to the excitation of a scintillation material after it interacts with incoming ionizing radiation. When radiation deposits enough energy in the scintillator, it excites the atoms within the material and leads to the subsequent light emission as those atoms return to their ground state. This light usually appears in the visible spectrum, which then can be detected by photodetectors, such as photomultiplier tubes.
Several factors can affect the efficiency of scintillation. Among these, the density and atomic number of the material play crucial roles in determining how effectively it can convert radiation energy into scintillation light. This groundbreaking mechanism underlies the detection methods widely used today, enabling advancements in fields like nuclear medicine, environmental science, and beyond.
Scintillation Materials
Different materials can be employed for scintillation depending on the application and desired characteristics. Scintillation materials can be broadly classified into two categories: organic scintillators and inorganic scintillators.
Organic Scintillators
Organic scintillators are primarily carbon-based compounds. They typically present a high light yield and fast time response, making them ideal for detecting high-energy particles. One of the key characteristics of organic scintillators is their ability to offer excellent energy resolution, which means they can distinguish between different energy levels of incoming radiation with finesse.
Their flexibility in usage is an additional aspect that makes organic scintillators popular. They are often easier to handle and can be manufactured in various forms, like liquids or plastics. However, a notable disadvantage is their susceptibility to quenching, which can reduce their effectiveness in certain experimental conditions, particularly under continuous high radiation exposure.
Inorganic Scintillators
In contrast, inorganic scintillators are generally composed of crystalline materials. They tend to have a higher density and atomic number, which can result in better interaction probabilities with incoming radiation. A key feature of these scintillators is their remarkably high stability, even in harsh environmental conditions. This stability serves the requirements of applications that demand long-term reliability, such as in long-term nuclear monitoring.
However, while inorganic scintillators generally provide good light output, they often exhibit slower response times compared to their organic counterparts. This trade-off can affect their suitability in certain high-speed applications where rapid detection is paramount.
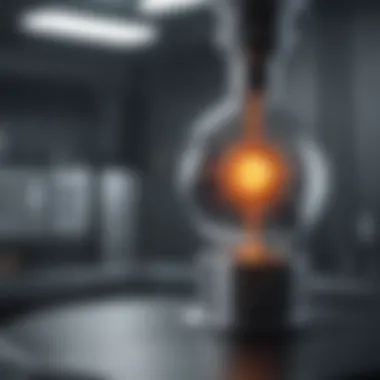
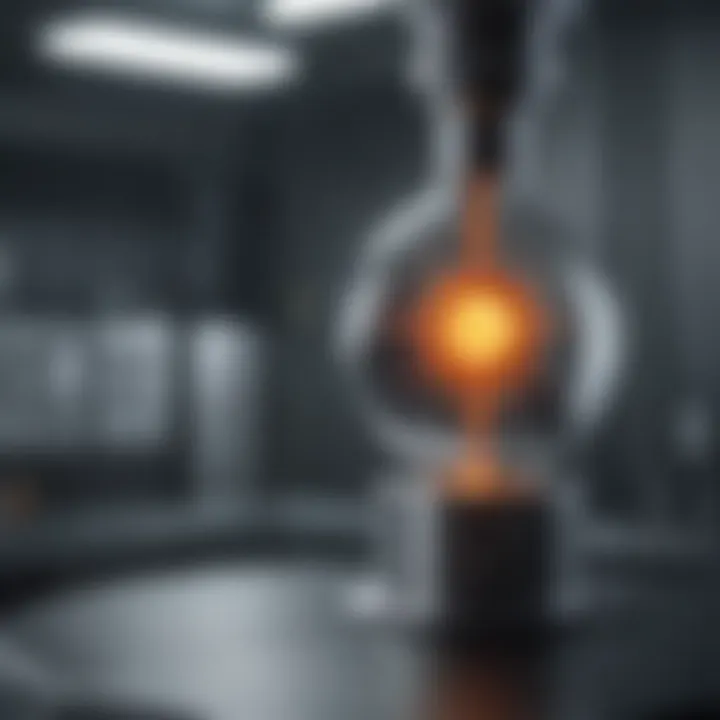
Types of Liquid Scintillation Techniques
Understanding the different types of liquid scintillation techniques is crucial in maximizing the utility of scintillation counting in various scientific applications. Each method caters to specific requirements in detecting ionizing radiation, thus improving the sensitivity and reliability of measurements. Essentially, these techniques serve as tools in diverse environments, from laboratories to field assessments, providing researchers and professionals with vital data concerning radiation levels and safety. The implications extend from clinical diagnostics to environmental science, underpinning the importance of precision in radiation measurement.
Traditional Liquid Scintillation Counting
Traditional liquid scintillation counting remains one of the cornerstone techniques in the world of radiation detection. This method employs organic solvents mixed with scintillation materials to detect and count beta and gamma radiation. When ionizing radiation interacts with the scintillator, it produces light pulses. The key is that these pulses are then translated into measurable signals.
Key Factors of Traditional Liquid Scintillation Counting:
- Sensitivity: The method is particularly sensitive to low-energy beta radiation, making it invaluable in research areas like radiobiology and biochemistry.
- Sample Versatility: A wide range of sample types—solution, solids, or even biological materials—can be examined using this technique.
- Data Quality: The quantitative nature allows for high-quality data essential in regulatory monitoring and safety assessments.
Utilizing this type of counting not only highlights its primary role in detecting radiation but also emphasizes its significance in generating reliable data that can influence health and environmental policies.
Radiolabeled Compound Detection
Radiolabeled compound detection employs liquid scintillation in the context of compounds that have been tagged with radioactive isotopes. This method is particularly prevalent in biological and chemical research. The functionality lies in how scintillation counting can assess the activity levels of these compounds within a biological matrix or a specific reaction environment.
Benefits of Radiolabeled Compound Detection:
- Dynamic Studies: Enables real-time monitoring of metabolic processes by observing tagged compounds in vivo.
- Quantitative Analysis: Offers precise measurements of compound activity, enhancing understanding in pharmacokinetics and drug development.
- Ease of Interaction Assessment: By tracking the radiolabeled compounds’ interaction with biological targets, researchers glean essential insights into mechanisms of action.
Integrating this approach into research not only showcases the method's adaptability but also reinforces its position as a critical component in studying complex biological systems.
Alpha and Beta Scintillation Detection
Alpha and beta scintillation detection techniques focus specifically on distinguishing particles based on their energy emissions and types, allowing detailed insights into radiation types present in a sample.
Distinctions to Consider:
- Alpha Scintillation Detection: Generally more straightforward than beta detection, alpha particles emit high-energy light as they travel through scintillator materials. Due to their high mass and charge, their interactions are characterized by short ranges and significant energy deposition. This makes alpha detection key in uranium or radon monitoring, for instance.
- Beta Scintillation Detection: While beta particles are lighter and penetrate matter more effectively, capturing their emissions requires careful calibration to distinguish between different energy levels. Beta detection finds its role in health physics, radioisotope tracing, and even environmental studies.
Overall, focusing on alpha and beta detection broadens the scope of liquid scintillation applications, ensuring that radiological analysis is thorough and precise.
Applications of Liquid Scintillation
The applications of liquid scintillation are as varied as they are significant, permeating multiple fields of scientific inquiry and industrial application. Understanding the role this technology plays across different sectors helps illuminate its value in contemporary research and practical applications. The core benefit of liquid scintillation counting lies in its sensitivity and versatility for detecting low levels of ionizing radiation. Whether in environmental monitoring or medical diagnostics, liquid scintillation serves as a vital tool for a wide spectrum of applications. Here, we delve into some key areas where liquid scintillation techniques have proven indispensable.
Environmental Monitoring
The necessity of environmental monitoring cannot be overstated, particularly in an age where radioactive contamination poses real risks to ecosystems and human health. Liquid scintillation plays an integral role in assessing the levels of radioactive isotopes in soil, water, and air. For instance, scientists can detect isotopes like tritium or carbon-14, which serve as biomarkers for pollution and environmental perturbations.
The technique allows for precise quantification, proving invaluable in areas near nuclear power plants or sites of past nuclear testing. Furthermore, by providing timely data, liquid scintillation contributes to the management of environmental hazards:
- Identifying radioactive leaks
- Monitoring background radiation levels
- Assessing compliance with environmental regulations
"Liquid scintillation counting not only enhances our ability to detect environmental pollutants but also helps ensure public safety by monitoring potential radiation exposure."
Nuclear Medicine and Dosimetry
In the realm of nuclear medicine, the use of liquid scintillation cannot be overlooked. This technology is primarily employed for monitoring dosage and tracking radiopharmaceuticals within the human body. Liquid scintillation enables doctors to ensure accurate dosing and minimize risks associated with radiation therapy.
Key applications include:
- Dose calculations: Liquid scintillation provides precise measurements of administered radioisotopes, making it possible to determine effective patient doses.
- Patient monitoring: By detecting residual radiation levels in patients post-treatment, healthcare providers can optimize follow-up care and enhance patient safety.
Additionally, liquid scintillation techniques are crucial for overall dosimetry in clinical settings. They allow for the continuous evaluation of radiation exposure to healthcare professionals, ensuring an acceptable safety margin in hospitals.
Radiobiology Studies
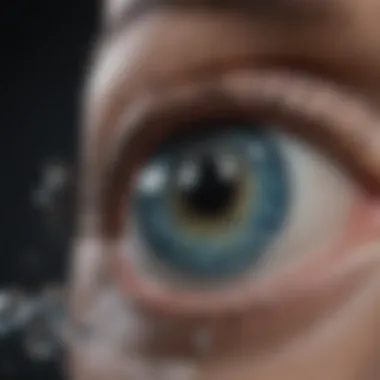
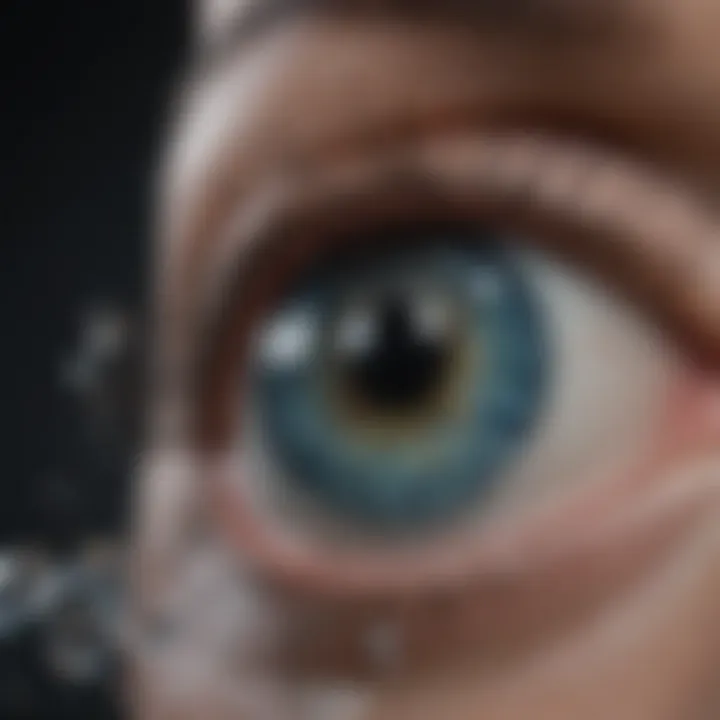
Radiobiology—a field focusing on the effects of ionizing radiation on biological systems—greatly benefits from liquid scintillation techniques. These methods enable the assessment of cellular responses to radiation, which is crucial for understanding both therapeutic applications and the implications of radiation exposure.
Researchers often utilize liquid scintillation to study:
- Cellular repair mechanisms: Understanding how cells recover from radiation damage can guide the development of protective agents or therapeutic strategies.
- Gene expression: Liquid scintillation helps in examining changes in gene expression following radiation exposure, leading to insights into cancer biology and treatment options.
Incorporating liquid scintillation counting into comprehensive experimental designs enhances the reliability of findings, thereby contributing to advancements in scientific knowledge and medical practices.
Industrial Applications
Industries that utilize radioactive materials are particularly benefitted by liquid scintillation techniques. In sectors ranging from pharmaceuticals to nuclear energy, the ability to detect and quantify radiation levels is paramount for safety and regulatory compliance.
Specific industrial applications include:
- Quality control: Ensuring the integrity of radioactive materials used in manufacturing processes.
- Radiation safety monitoring: Continuous assessment of work environments to maintain safe radiation levels for employees.
Moreover, liquid scintillation plays a crucial role in developing new materials, particularly in assessing their inherent radiological properties before mass production.
In summary, the applications of liquid scintillation extend across diverse fields, underscoring its pivotal role in modern science and industry. With its ability to detect low levels of radiation, maintain safety standards, and foster environmental protection, liquid scintillation remains an indispensable tool for researchers, healthcare professionals, and industry experts alike.
Advantages of Liquid Scintillation Techniques
Liquid scintillation techniques have carved a niche for themselves within the realm of radiation detection. Their benefits go beyond the mere act of measuring ionizing radiation; they encompass a range of factors that enhance their overall utility in scientific research. A deeper look at these advantages reveals why they have become a prominent choice in various applications.
Sensitivity and Detection Limits
One of the standout features of liquid scintillation counting lies in its unmatched sensitivity. These techniques can detect even the faintest signals of ionizing radiation, making them valuable in scenarios where detecting low-level activity is critical.
- Low Background Count: Liquid scintillation counters typically have lower background radiation levels, which helps in distinguishing the signal from noise more effectively. This is especially pertinent in environments where contamination might skew results.
- Energy Resolution: These methods also boast excellent energy resolution. This means that the instruments can accurately differentiate between the energies of different radioactive isotopes. Such capability is particularly important in analytical applications, such as environmental monitoring, where understanding the type of radiation present can inform subsequent actions.
- Quantitative Measurements: Researchers can obtain highly quantitative results, allowing for accurate assessments of radionuclide concentrations in samples. For instance, in medical diagnostics, this sensitivity plays a crucial role in determining minuscule amounts of radiolabeled compounds.
"In locations where precision is paramount, liquid scintillation techniques elevate the standard of detection, making them the preferred method for many researchers."
Versatility in Detection Methodologies
Another remarkable advantage of liquid scintillation techniques is their versatility. The methodologies can be adapted to detect a variety of ionizing particles, making them suitable for a wide range of applications.
- Multiple Types of Detection: From measuring alpha particles to beta radiation, the adaptability of fluid scintillation mediums allows for diverse usage. This multi-functionality can reduce the need for multiple specialized instruments, streamlining the detection process.
- Integration into Various Systems: Additionally, liquid scintillation can be easily integrated with other detection systems, enhancing its capabilities. Coupling this technique with data processing software, for instance, can bolster analysis speed and accuracy.
- Application in Diverse Fields: Whether used in nuclear medicine, environmental research, or industrial settings, the broad applicability of liquid scintillation techniques makes them a cornerstone in radiation detection.
The advantages of liquid scintillation techniques encapsulate an intricate balance of sensitivity and versatility. Their unique features not only enhance detection processes but also expand the horizons for scientific research, echoing their importance in modern science.
Challenges and Limitations
Liquid scintillation, while immensely useful, does come with its own set of challenges and limitations that researchers, engineers, and practitioners in various fields must navigate. Understanding these hurdles is crucial not just for effective application, but also for innovation and improvement of the technology. This section will delve into two primary areas of concern: the chemical stability of scintillators and the environmental implications associated with their disposal.
Chemical Stability of Scintillators
Chemical stability is a critical factor in the effectiveness of liquid scintillation systems. The scintillation materials used must remain consistent in performance over time and under varying conditions. Degradation of these materials can lead to fluctuations in detection efficiency, skewing results significantly. For example, organic scintillators often suffer from photodegradation when exposed to light, causing them to lose their capacity to effectively detect radiation. Consequently, researchers are tasked with selecting materials that not only emit scintillation light effectively but also maintain their properties through prolonged use.
Factors influencing chemical stability include:
- Temperature Sensitivity: High or repeated temperature fluctuations can alter the molecular structure of scintillators, resulting in decreased performance.
- Chemical Compatibility: Interactions with other chemical compounds in a sample may also destabilize the scintillation solutions.
- Radiation Exposure: Continuous exposure to radiation can deteriorate scintillation materials, necessitating frequent replacement.
Inadequate chemical stability can lead to wasteful practices and increased costs, as materials need regular replacements or adjustments. Therefore, employing more resilient scintillation materials and developing better preservation techniques are vital steps in overcoming these challenges.
Disposal and Environmental Concerns
The disposal of liquid scintillation wastes brings its own set of complexities and environmental concerns. As certain scintillation materials are hazardous, their disposal must be managed carefully, adhering to strict regulations. Improper disposal methods can pose serious risks to public health and the environment, including:
- Toxic Chemical Release: Some components of scintillation cocktails are chemically hazardous, potentially leading to contamination of soil and water if not disposed of properly.
- Radioactive Waste Management: Many liquid scintillation systems involve radioactive substances, necessitating stringent protocols for handling, storage, and disposal.
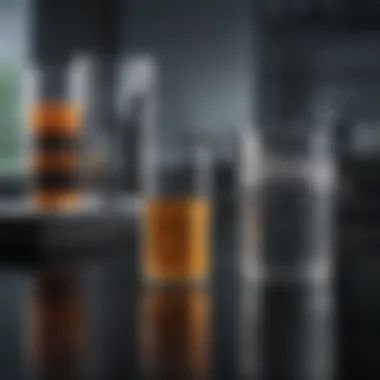
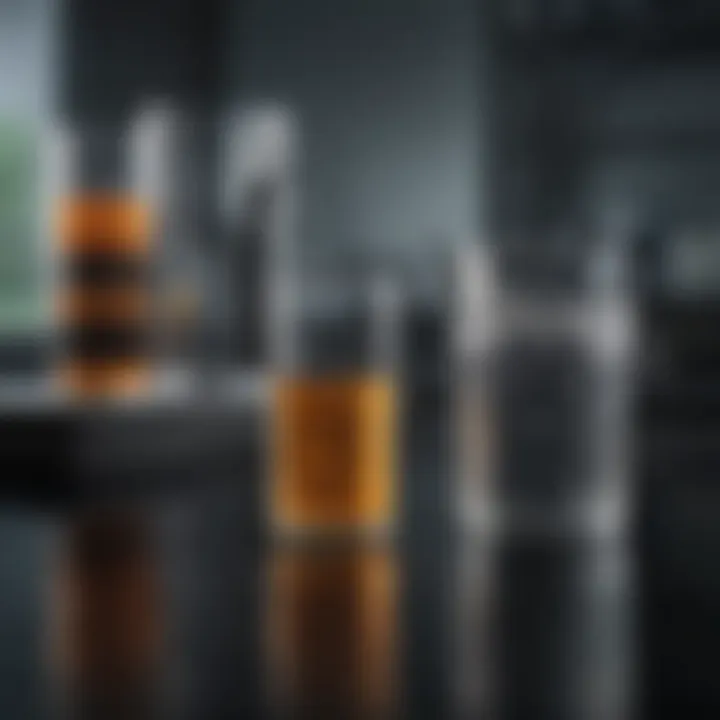
The growing emphasis on sustainability in scientific practices has led to increased scrutiny of waste management strategies. Researchers are exploring greener alternatives to traditional scintillators, assessing bio-based or less harmful substances. Innovations and improved protocols in waste disposal challenges are essential.
"Understanding the challenges helps unlock pathways for innovative solutions. As we become more aware, our ability to develop sustainable practices grows."
Solving the issues surrounding chemical stability and waste disposal is fundamentally important for ensuring that liquid scintillation remains a viable and responsible method for radiation detection. Innovating in these areas not only advances the technology itself but also aligns scientific practice with a responsible stewardship of the environment.
Innovations in Liquid Scintillation Technology
Liquid scintillation technology is evolving, and these innovations are not just enhancements—they signify a substantial leap forward in our capabilities to detect and measure ionizing radiation with fidelity. As the demands on this technology grow, so do the efforts to refine its components, expand its applications, and integrate it into the broad landscape of scientific instrumentation. This section will explore the significant advancements made in scintillation materials and the integration of liquid scintillation with modern detection systems.
Advancements in Scintillation Materials
The heart of liquid scintillation counting lies in its scintillation materials, which convert the energy from ionizing radiation into visible light. Recent developments in these materials have opened new avenues for enhancing sensitivity and selectivity in radiation detection.
- Organic Scintillators: These have long been the workhorses of liquid scintillation. Advancements in organic compounds now allow for higher light yields and faster response times. New chemical formulations, such as those based on phenolic compounds, increase the efficiency and stability of light production in varied conditions.
- Inorganic Scintillators: Once thought less efficient for liquid applications, new combinations of inorganic materials exhibit impressive photon yields, significantly boosting detection capabilities. The introduction of novel dopants has transformed these materials into viable contenders against their organic counterparts.
- Nanocomposites: A particularly exciting area of research involves nanotechnology. By incorporating nanoparticles into the scintillation medium, scientists are discovering ways to enhance light output and tailor responsiveness to specific radiation types. This paves the way for more precise measurements in fields ranging from radiopharmaceutical development to environmental monitoring.
“The continuous improvement of scintillator materials illustrates a broader trend: science isn't static. Materials that once had limitations are being pushed beyond prior boundaries, resulting in new capabilities.”
Integration with Modern Detection Systems
As liquid scintillation techniques grow in sophistication, so does the need for their integration with advanced detection systems. In a world that thrives on data, the intersection of scintillation with cutting-edge technology is paramount.
- Digital Detection Systems: The shift towards digital systems allows for enhanced data collection and analysis. Coupling digital signal processing with liquid scintillation counters leads to more accurate interpretation of scintillation events, reducing noise and improving signal clarity.
- Automated Sample Handling: Automation has improved throughput in labs. With robotic systems handling sample preparation and measurement, laboratories can achieve better consistency and a significant increase in productivity, eliminating human error in routine tasks.
- Real-Time Monitoring: Integrating liquid scintillation counters with real-time monitoring systems allows for immediate feedback on radiation levels. This is crucial in situations like nuclear facilities or environmental assessments, where time-sensitive decisions are necessary. The combination helps in creating a dynamic response to any anomalies detected, bolstering safety and compliance measures.
The innovations in liquid scintillation technology signify a transformative moment in how we perceive, measure, and analyze radiation. As these technologies continue to advance, they open new frontiers in research and application, reflecting a responsive and responsible scientific community ready to meet the challenges of the future.
Future Directions and Research Trends
The landscape of liquid scintillation techniques is evolving rapidly, driven by advancements in science and technology. Understanding future directions in this field is critical not only for improving current methodologies but also for revealing new possibilities in research. Here, we will explore both emerging research areas and the pivotal role liquid scintillation could play in future studies.
Emerging Research Areas
Liquid scintillation is finding a niche in diverse areas of research that were not previously considered. Some key emerging research topics include:
- Nanotechnology Integration: There is growing interest in employing nanomaterials to enhance scintillation properties. Incorporating nanoparticles can increase light output and improve energy resolution, which could be pivotal in detecting low-energy emissions.
- Biological Applications: Studies in radiopharmaceuticals are gaining traction. Liquid scintillation could become a key player in developing new diagnostic tools and therapeutic strategies in medicine, particularly cancer treatment.
- Environmental Radiation Monitoring: As global awareness of radiation in the environment rises, new methodologies are being explored for using liquid scintillation for real-time monitoring of radioactive pollutants.
- Advanced Computing for Data Analysis: The integration of machine learning in data processing offers potential to improve the speed and accuracy of liquid scintillation counting techniques, enabling better analytical capabilities and diagnostics.
The exploration of these areas promises not only to enhance our scientific understanding but also to address practical challenges in health, safety, and the environment.
The Role of Liquid Scintillation in Future Studies
As new research avenues unfold, the relevance of liquid scintillation will only increase. Its role can be summarized in several aspects:
- Enhanced Sensitivity: Future studies in nuclear medicine could leverage the high sensitivity of liquid scintillation to more accurately monitor radiation doses in patients, helping to improve treatment outcomes.
- Integration with Modern Detection Systems: Liquid scintillation is not an isolated technology. Its future will likely involve more synergies with other detection systems, facilitating multi-modal approaches in scientific research that could combine various data streams for richer insights.
- Long-term Environmental Studies: With ongoing monitoring of environmental health becoming a priority, liquid scintillation could help scientists study the long-term effects of radiation exposure on ecosystems.
The continuing evolution of liquid scintillation technology, therefore, holds significant promise for a wide array of applications. As researchers move forward, the insights gained through this technique will likely lead to innovations that enhance our capacity to detect, identify, and understand ionizing radiation in context.
"Liquid scintillation is set to illuminate the path for future innovations in detection technologies, paving the way for breakthroughs our society sorely needs."
End
The conclusion of this article emphasizes the multifaceted importance of liquid scintillation in various scientific domains. This technique is not just a method for detecting ionizing radiation; it is a gateway into understanding the very essence of particles that interact with matter. The principle at work here, which relies on the internal conversion of energy into light, unlocks many vital applications across major fields like nuclear medicine, environmental science, and even industrial processes.
Implications for Science and Society
Liquid scintillation has profound implications that stretch beyond laboratory walls and into real-world applications. In nuclear medicine, it aids in developing diagnostics and therapeutic strategies that can save lives. The technology enhances our ability to monitor environmental radiation levels, contributing to public safety and the health of ecosystems.
Moreover, advancements in this field have ramifications that can foster collaboration among diverse scientific disciplines. Liquid scintillation is not a siloed approach; it encourages interdisciplinary efforts, combining chemistry, physics, and biology to shape future research directions.
"The ability to detect even minute levels of radioactive contamination places liquid scintillation at the forefront of both scientific inquiry and societal concern."
From a societal standpoint, the responsible use of liquid scintillation technologies has the potential to inform policy decisions and regulations, especially concerning nuclear energy and waste management. As we grapple with the environmental impacts of various technologies, liquid scintillation can bridge the gap between safety and innovation.
In light of the advancements discussed, researchers and industry professionals are urged to consider the ethical implications of these technologies. The balance between scientific progress and its impact on society is delicate and requires constant vigilance.
In summary, liquid scintillation is more than a functional technique for measurement; it embodies a critical intersection of science and societal well-being. As we move forward, its role in enhancing detection methodologies and broadening applications showcases the technology’s significance in contemporary research and its potential to shape the future.