Unveiling the Critical Roles of Glial Cells
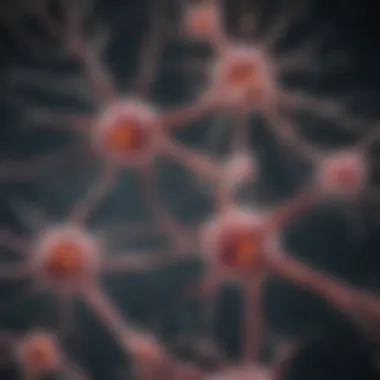
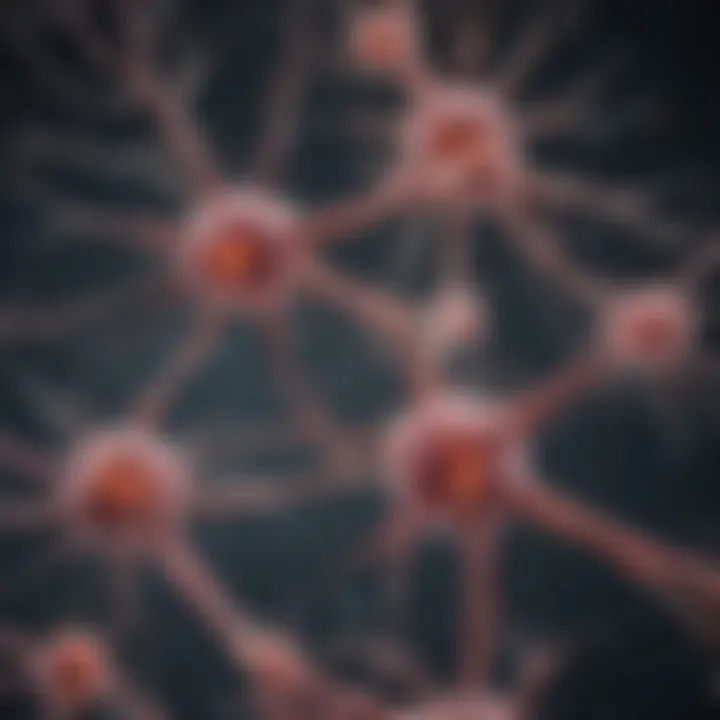
Intro
Glial cells, once regarded merely as support cells in the nervous system, are now understood as integral participants in numerous physiological processes. This article explores the various types of glial cells, each with unique functions that contribute to the overall well-being of the nervous system. The intricate and multifaceted roles played by these cells underline their importance in maintaining homeostasis, conserving neuronal integrity, and promoting effective communication within neural networks.
Current research continues to unveil their critical impact on brain health and disease, increasing interest in their potential therapeutic applications. By delving into glial cell functions, we aim to illuminate their vital contributions and inspire further exploration in neurobiology.
Key Findings
Major Results
Recent investigations into the workings of glial cells have provided significant insights into their role. Key findings include:
- Diversity of Cell Types: Glial cells encompass a variety of types, such as astrocytes, oligodendrocytes, and microglia. Each type has distinct roles and functions that facilitate neuronal support.
- Homeostatic Maintenance: Glial cells help regulate ion balance and neurotransmitter levels in the brain, which is crucial for proper neuronal activity.
- Neuroinflammation and Repair: Microglia, the resident immune cells of the brain, are essential for responding to injury and protecting against pathogens. They can contribute to both protective and detrimental effects depending on the context.
- Neurotransmission Support: Astrocytes participate in neurotransmitter recycling, thereby modulating synaptic activity and contributing to learning and memory processes.
Discussion of Findings
The findings suggest that glial cells are not merely passive supporters but active participants in the nervous system's functionality. Understanding their complex roles could lead to revamping approaches for treating neurological disorders, from Alzheimer’s disease to multiple sclerosis.
"Glial cells are pivotal players in the neural circuitry, shedding light on their importance for therapeutic advancements and brain health."
Methodology
Research Design
The exploration of glial cell functions typically employs a combination of experimental and observational studies. Research designs often integrate neuroimaging techniques, cellular assays, and in vivo models to assess the behavior of these cells under various conditions.
Data Collection Methods
Data is collected through several approaches, including:
- Immunohistochemistry: This technique aids in visualizing specific protein expressions in glial cells.
- Electrophysiology: Used to examine the electrical properties of glial cells and their interactions with neurons.
- Genetic Manipulations: These methodologies explore the functions of specific glial cell types by knocking out or over-expressing genes of interest.
By dissecting the methodologies and examining emerging findings, this article aims to provide a comprehensive understanding of glial cells and their functions.
Prologue to Glial Cells
Glial cells are an integral component of the nervous system, often overshadowed by the more conspicuous neurons. In this article, we explore their multifaceted roles, illustrating their importance in maintaining brain health and functionality. Understanding glial cells is vital due to their numerous functions, which extend far beyond mere support for neurons. Glial cells contribute to homeostasis, protection, and the facilitation of communication within the nervous system.
In this section, we will define glial cells, provide their historical context, and highlight their evolving understanding within neuroscience. Delving into how scientists have historically viewed these cells enhances our contemporary understanding and sets the stage for discussing their various functions and implications in health and disease.
Definition and Overview
Glial cells, or neuroglia, are non-neuronal cells in the central and peripheral nervous systems. They serve multiple roles including:
- Providing support and insulation for neurons.
- Maintaining homeostasis, specifically by regulating the extracellular environment.
- Contributing to the repair process following injury.
There are several types of glial cells, each with distinct functions. For instance, astrocytes are involved in nutrient transport and waste removal, while oligodendrocytes form the myelin sheath essential for efficient electrical signaling. Microglia play a key role in immune defense within the brain, while ependymal cells contribute to cerebrospinal fluid production and circulation.
Understanding these definitions and the roles of different glial cells paves the way for more in-depth discussions on their functions throughout this article.
Historical Context
Historically, glial cells were regarded as mere supporting actors in the intricate play of the nervous system. The term "glia" is derived from the Greek word for "glue," reflecting the early belief that these cells primarily served to bind and support neurons.
This perception began to shift in the latter half of the 20th century as researchers uncovered the functional diversity within glial cells. Pioneering studies demonstrated that glial cells possess dynamic properties and participate actively in neural communication. Some scientists, such as Ramón y Cajal, laid the groundwork by identifying glial cells as crucial to the structure of the nervous system, yet their roles remained poorly understood for decades.
In recent years, breakthroughs in molecular biology and imaging techniques have greatly enhanced our grasp of glial cell functions. Studies have shown that glial cells are not simply passive entities, but active players in various neural processes encompassing protection, repair, and even modulation of synaptic activity. This evolving perspective on glial cells continues to shape current neurological research and therapeutic approaches.
"Glial cells are no longer seen as mere support cells but as integral participants in the functioning of the nervous system."
The historical context establishes a foundation for the in-depth exploration of glial cell types and their essential functions in the nervous system, which will be discussed in subsequent sections.
Types of Glial Cells
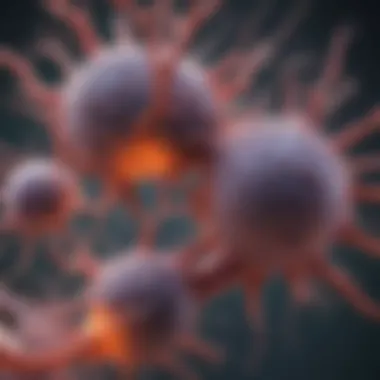
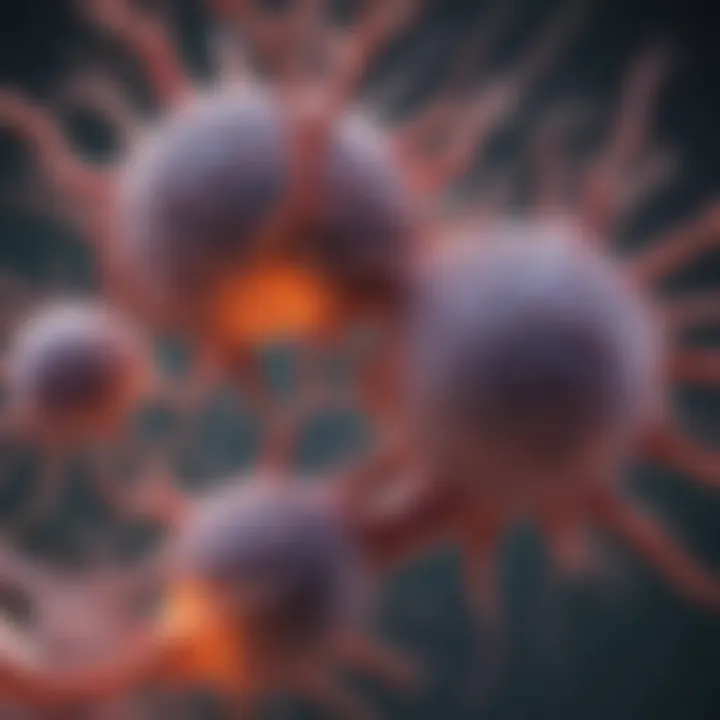
The study of glial cells is essential for understanding the entire nervous system. Unlike neurons, which are primarily responsible for transmitting signals, glial cells perform a variety of key functions that ensure the health and functionality of neurons. Identifying the different types of glial cells is crucial. Each type has unique roles that support various aspects of nervous system function. Recognizing these distinctions enhances our understanding of neural communication, homeostasis, and adaptability.
Astrocytes
Astrocytes are star-shaped glial cells that serve several critical functions within the central nervous system. They contribute to the structural integrity of the brain by providing a scaffold that supports neurons physically. Astrocytes are also involved in regulating blood flow in the brain. By sensing neuronal activity, they can dilate or constrict blood vessels to meet metabolic demands. This ability makes astrocytes integral to maintaining homeostasis.
Moreover, astrocytes play a significant role in neurotransmitter uptake and recycling. They manage specific neurotransmitters like glutamate, preventing excitotoxicity, which can damage neurons. Inovaations in researching astrocyte functions indicate their influence on signaling pathways and synaptic plasticity, underscoring their importance in learning and memory.
Oligodendrocytes
Oligodendrocytes are responsible for myelinating axons in the central nervous system. They create myelin sheaths that insulate axons, leading to increased speed and efficiency of nerve signal transmission. The role of oligodendrocytes in neuronal protection and support cannot be overstated. Without myelination, neural communication would be significantly impaired, leading to various neurological issues.
Research has shown that oligodendrocytes also participate in metabolic support for axons. They provide crucial nutrients to maintain the survival and functionality of neurons. A growing body of evidence indicates that oligodendrocytes may play a role in neuroinflammation and neurodegeneration, making them important in the context of diseases like multiple sclerosis.
Microglia
Microglia are the immune cells of the central nervous system. They are small but highly dynamic and serve a dual role in maintaining brain health. First, they constantly monitor the environment and respond to injury or pathogens. Microglia's activation leads to the release of pro-inflammatory cytokines, which can initiate repair mechanisms. However, excessive activation can contribute to neuroinflammation and further damage.
Additionally, microglia play a role in synaptic pruning during development and in response to learning. This process is crucial for refining neural circuits and ensuring efficient communication. Their ability to adapt suggests a previously underestimated level of influence on overall brain function.
Ependymal Cells
Ependymal cells line the ventricles of the brain and the central canal of the spinal cord. They serve important functions, notably the production and circulation of cerebrospinal fluid (CSF). CSF offers mechanical protection, removes waste, and provides buoyancy to the brain. Ependymal cells are ciliated, which aids in propelling CSF through the ventricular system.
New studies indicate that ependymal cells may also serve as progenitors for other glial cells. This finding opens new avenues for understanding how these cells contribute to repair mechanisms after injury.
In summary, understanding the types of glial cells is fundamental to neuroscience. Each type contributes distinctly to the homeostasis, protection, and functional enveloping of nervous tissue. This knowledge fosters deeper insights into potential therapeutic targets for various neurological disorders.
Core Functions of Glial Cells
The core functions of glial cells are fundamental to the proper operation of the nervous system. These functions go beyond the traditional view of glial cells as mere support structures for neurons. Instead, glial cells play crucial roles in maintaining the intricate balance necessary for neuronal health and communication. Their varied responsibilities encompass support, protection, maintenance, and facilitation of neural function. Understanding these roles is essential to grasping how the nervous system operates as a whole and how disruptions in these functions can lead to dysfunction or disease.
Support of Neuronal Function
Glial cells provide essential support to neurons in several ways. One prominent example is the role of astrocytes. Astrocytes are star-shaped cells that surround synapses and blood vessels, helping to regulate neurotransmitter levels. They also provide metabolic support to neurons by supplying nutrients. This relationship between astrocytes and neurons is vital for sustaining neuronal activity.
Moreover, oligodendrocytes form the myelin sheath around axons. This myelination is crucial because it increases the speed of electrical impulses traveling along the nerve fibers. Consequently, efficient communication between neurons relies heavily on the supportive functions of these glial cells.
In addition, microglia act as the first line of defense in the central nervous system, supporting neuronal function by removing cellular debris and ensuring a healthy environment for neurons to thrive.
Homeostasis Maintenance
Glial cells are integral to maintaining homeostasis within the nervous system. They regulate the extracellular environment, ensuring that ions and neurotransmitters are kept at appropriate levels. For instance, astrocytes have the capacity to take up excess potassium ions released during neuronal firing. This activity prevents hyperexcitability and maintains the resting membrane potential of neurons.
Moreover, glial cells participate in the regulation of the blood-brain barrier. The blood-brain barrier is a selective barrier that protects the brain from toxins while allowing essential nutrients to pass through. Astrocytes and endothelial cells work closely together to maintain this barrier, thus safeguarding the brain’s environment. This process illustrates how glial cells contribute to homeostasis, ultimately protecting neural function and integrity.
Protection and Repair Mechanisms
Another critical function of glial cells is their protective role during injury and disease. Microglia, for example, can become activated in response to injury or disease states. Upon activation, they adopt a phagocytic role, clearing debris and releasing cytokines that can influence inflammation and repair processes. This response is crucial in mitigating damage and beginning the repair process after neural injury.
Moreover, in the peripheral nervous system, Schwann cells are known for their remarkable ability to regenerate damaged axons. They guide the regrowth of neurons, effectively supporting recovery from injuries. The protective and repair functions are an essential aspect of glial cell biology, and their effective operation is paramount in maintaining neural health and longevity.
Glial cells not only support neurons but also play active roles in maintaining homeostasis, protecting against injury, and facilitating repair.
In summary, the core functions of glial cells reveal their complexity and importance within the nervous system. Their supportive roles are intertwined with homeostatic balance and protective mechanisms. Understanding these functions is vital for comprehending potential therapeutic interventions in neurological diseases, as targeting glial cells could yield new avenues for treatment.
Role in Neural Communication
The role of glial cells in neural communication is profound. These cells, once considered merely supportive, actively participate in regulating the interactions between neurons. They influence how signals are transmitted, altered, and processed. Understanding this domain not only heightens our appreciation of brain activity but also frames glial cells as essential contributors to overall brain functionality. The following subsections delve into specific aspects of this intricate communication.
Chemical Signaling
Glial cells engage in chemical signaling through a variety of mechanisms. They release neurotransmitters, which are fundamental to neuronal communication. For instance, astrocytes promote synaptic transmission by releasing glutamate, a major excitatory neurotransmitter.
Moreover, glial cells also respond to signals from neurons, exhibiting a two-way communication system. This reciprocal interaction suggests that glial cells fine-tune neuronal responses and play a significant role in modulating synaptic activities. In many cases, they help maintain the balance of ions and molecules in the synaptic cleft, ensuring that chemical signals can effectively propagate through the neural networks.
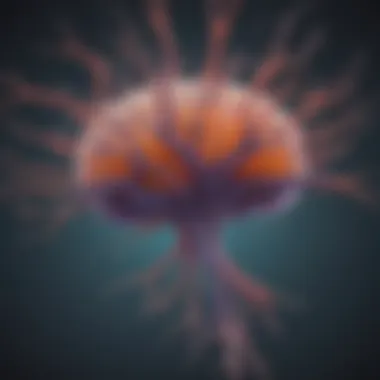
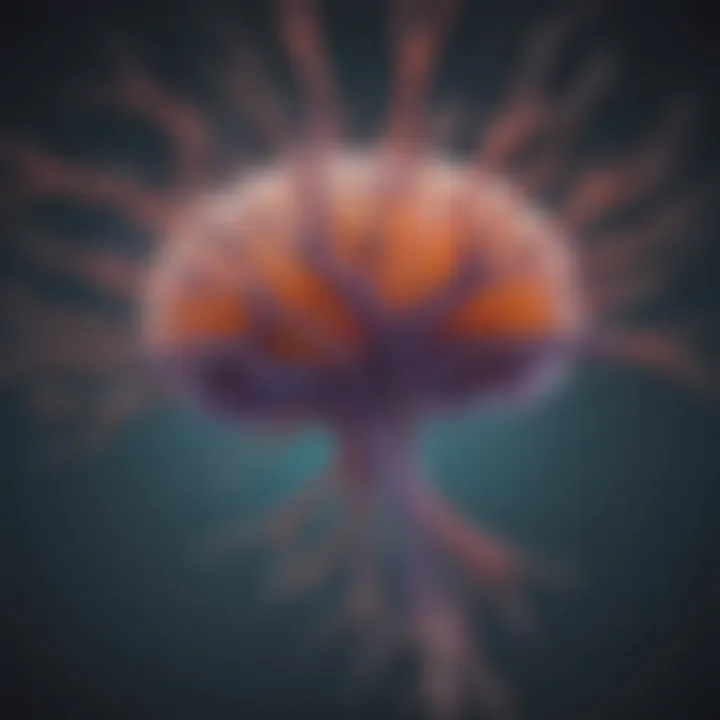
"The intricate communication between glial cells and neurons reveals a layered complexity in brain function that researchers strive to understand."
Electrical Signaling
Electrical signaling is another crucial aspect of glial function in neural communication. While neurons primarily communicate through action potentials, glial cells also utilize electrical signals. Certain types of glial cells, such as astrocytes, have been shown to engage in calcium signaling. This process involves fluctuations in intracellular calcium levels, which can lead to the release of gliotransmitters and affect nearby neurons.
The ability of glial cells to generate electrical signals supports the concept of astrocytic connectivity. Such connectivity ensures that glial cells can work synchronously across large areas of the brain, potentially influencing many neurons simultaneously. This aspect highlights their role not just as passive responders but as active participants that can affect brain states.
Synaptic Functionality and Plasticity
The involvement of glial cells in synaptic functionality and plasticity is critical for understanding learning and memory. Glial cells, particularly astrocytes, have a role in modulating synaptic strength and efficacy. They can respond to neuronal activity and subsequently adjust their signaling pathways. This adaptability aids in synaptic plasticity—the process that underlies learning.
Research indicates that glial cells can influence long-term potentiation (LTP) and long-term depression (LTD), both essential for synaptic plasticity. By regulating neurotransmitter uptake and ion concentrations, glial cells not only support but also shape the very processes of synaptic communication.
Glial Cells and Neuroinflammation
Neuroinflammation is a crucial aspect to consider when examining the functions of glial cells in the central nervous system. Glial cells, particularly microglia, play an essential role in maintaining the overall health of the brain. Their ability to respond to injury and disease highlights their importance not only in homeostasis but also in the pathology of various neurological conditions. Understanding how glial cells contribute to neuroinflammation offers valuable insights into potential therapeutic approaches to mitigate adverse effects.
Microglial Activation
Microglia are the resident immune cells of the central nervous system. They constantly monitor the environment and respond to signs of inflammation or injury. Upon activation, microglia undergo morphological changes, often becoming more amoeboid in shape. This transformation enables them to migrate to sites of damage or infection. Microglial activation results in the release of various signaling molecules, such as cytokines and chemokines, which can influence both local and systemic immune responses.
While microglial activation can be protective, prolonged or excessive activation may lead to neurodegeneration. In many neurodegenerative diseases, microglia adopt a pro-inflammatory phenotype, contributing to a cycle of chronic inflammation. This highlights the dual role of microglia as both defenders and potential aggressors in neuronal health. Understanding the balance between their beneficial and harmful functions is crucial in the context of neuroinflammation.
Role in Neurodegenerative Diseases
The contribution of glial cells to neurodegenerative diseases such as Alzheimer's, Parkinson's, and multiple sclerosis cannot be overlooked. In these conditions, neuroinflammation orchestrated by microglial activation plays a pivotal role. For instance, in Alzheimer's disease, the accumulation of amyloid-beta plaques leads to microglial activation, which, in turn, can exacerbate neuronal damage and contribute to disease progression.
Research indicates that targeting microglial activation may present new avenues for treatment. Strategies that either inhibit excessive activation or enhance protective microglial functions could potentially slow disease progression. Additionally, understanding the mechanisms of microglial involvement in neurodegenerative processes can aid in the development of biomarkers. These biomarkers could help in diagnosing conditions earlier and more accurately.
Neuroinflammation remains a complex field of study with significant implications for glial cell functions. The insights gained from this research not only enhance our comprehension of brain health but also contribute to advancing therapeutic strategies. As investigations continue to deepen, the correlation between glial cells and neuroinflammation may lead to transformative advancements in combating neurological disorders.
Impact on Neurodevelopment
The role of glial cells in neurodevelopment is critical and multifaceted. Understanding their functions at various stages of brain maturation helps clarify how these cells influence both physiological and pathological processes in the nervous system. Glial cells are not just passive support structures; they actively participate in the development of neural circuits and the overall architecture of the brain. This has significant implications for both normal brain development and the emergence of neurological disorders.
Glial Development Across Life Stages
Glial cells undergo substantial changes throughout an organism's life. Early in development, astrocytes and oligodendrocytes are formed from neural progenitor cells. These early-stage glial cells are essential for the proper growth and differentiation of neurons. They provide scaffolding that guides migrating neurons and secrete factors that promote neuronal survival and maturation. It is critical to note that the timing of glial cell development is tightly regulated, creating a suitable environment for neural connections.
In infancy, glial cells continue to proliferate and differentiate. Astrocytes become more numerous and carry out important functions, such as maintaining the blood-brain barrier and regulating synaptic transmission. In adolescence, the maturation of oligodendrocytes leads to increased myelination of axons, which boosts the speed of neural transmission. This process is not only essential for cognitive development but also for emotional regulation.
As individuals age, glial cells maintain their roles but can also become reactive in response to injury or neurodegenerative processes. This reactivity can influence neurodevelopmental conditions. Therefore, understanding glial development offers insights into various life stages and their correlation to cognitive, emotional, and behavioral functions.
Influence on Neurogenesis
Glial cells play a significant role in neurogenesis, the process of generating new neurons. Research indicates that astrocytes in the adult brain can give rise to neurons through a process called astrocytic neurogenesis. This is particularly relevant in regions like the hippocampus, where new neurons are generated throughout life and contribute to learning and memory functions.
Furthermore, glial cells release neurotransmitter-like substances and growth factors that can stimulate neurogenesis. For example, brain-derived neurotrophic factor (BDNF), produced by astrocytes, supports the survival of new neurons and enhances synaptogenesis.
"Glial cells support neurogenesis not just by providing a physical structure, but also through direct biochemical signaling that shapes neural fate and function."
Despite these beneficial effects, an imbalance in glial function can impair neurogenesis. In conditions such as stress or neuroinflammation, the release of pro-inflammatory cytokines by activated microglia can hinder the generation of new neurons, thereby negatively impacting cognitive abilities.
Thus, the influence of glial cells extends beyond structural support to directly affect the birth of new neurons and their integration into existing neural networks. This highlights the necessity of further studies to explore therapeutic strategies targeting glial functions to enhance neurogenesis, particularly in the context of cognitive disorders.
Therapeutic Implications of Glial Function
The role of glial cells in the nervous system extends well beyond traditional support functions. Understanding these cells' therapeutic implications has gained considerable attention in recent years. This section highlights the potential contributions of glial cells to regenerative medicine and identifies them as promising drug targets in various neurological conditions.
Glial Cells in Regenerative Medicine
Regenerative medicine is an evolving field that aims to repair or replace damaged cells, tissues, or organs. Glial cells hold significant potential in this area. For instance, astrocytes have shown capabilities in promoting neural repair after injury. They release growth factors that support neuronal survival and regeneration. The modulation of microglial activity is also crucial in managing inflammation and enhancing recovery post-injury.
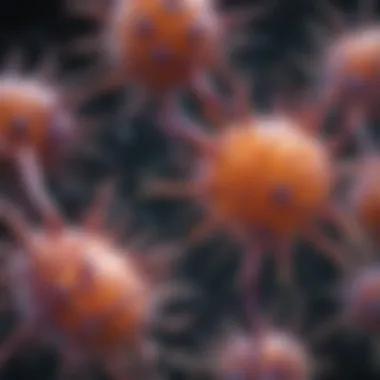
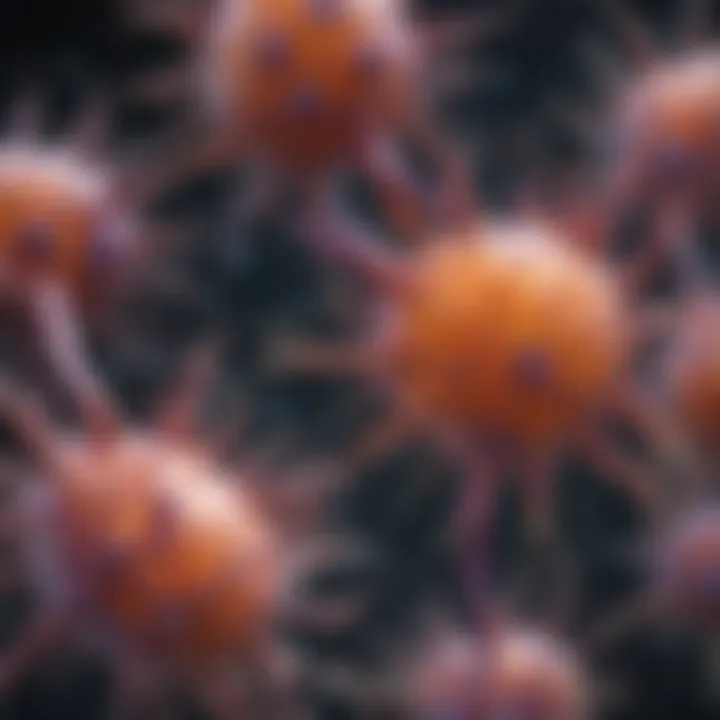
Recent studies have suggested that enhancing the function of oligodendrocytes can lead to remyelination, especially in disorders such as multiple sclerosis. The ability to manipulate glial cells could lead to breakthroughs in treatments that focus on restoring lost functions.
Glial cells are not just passive players; they actively participate in the repair processes of the nervous system.
Potential Drug Targets
Glial cells present unique opportunities as drug targets in various therapeutic contexts. Their involvement in neuroinflammation, neurodegeneration, and other neurological disorders positions them as focal points for drug development. There is an emerging understanding that targeting specific mechanisms of glial function can lead to favorable outcomes in treating diseases such as Alzheimer's and Parkinson's.
For example, inhibiting the excessive activation of microglia can mitigate damage caused by chronic inflammation in the brain. Similarly, pharmacological approaches that enhance astrocyte function might improve the support provided to neurons, thus protecting them from neurotoxic insults.
Challenges in Glial Cell Research
Researching glial cells presents a range of challenges that can complicate efforts to fully understand their roles within the nervous system. These challenges are important because they affect how scientists view glial cells and their contributions to brain health and disease. Addressing these issues will advance our comprehension of glial functions and lead to potential therapeutic strategies in neurological disorders.
Methodological Limitations
One of the primary hurdles in glial cell research is methodological limitations. Traditional techniques often lack specificity when studying the complex interactions of glial cells with neurons. This complexity arises because glial cells are not as abundant as neurons, and their diverse subtypes perform various functions. As a result, isolating specific glial cell types for study can present significant difficulties.
Moreover, methods like immunostaining may not adequately differentiate among glial cell types. In addition, there is a reliance on animal models, which may not completely mimic human nervous system function. This gap can lead to results that, while informative, do not always directly translate to human health.
"Advancements in imaging technologies and genetic manipulation techniques hold promise for overcoming some of these research limitations."
Interdisciplinary Approaches
To counteract the methodological challenges, interdisciplinary approaches to glial cell research are essential. Integrating insights from fields such as molecular biology, bioinformatics, and neuroscience can promote comprehensive studies. For example, collaborations between biologists, chemists, and data analysts can yield new tools and methods to investigate glial cells more effectively.
Understanding the molecular signaling pathways involving glial cells requires a multidisciplinary understanding. By leveraging knowledge across various scientific domains, researchers can better address questions surrounding glial cell behaviors and their impacts on neurodevelopment and pathology.
Future Directions in Glial Cell Studies
As research on glial cells continues to advance, the future directions in this field hold promising implications for both basic neuroscience and clinical applications. Understanding how glial cells function in health and disease is crucial. This exploration can lead to breakthroughs in treatment strategies for a variety of neurological disorders. The drive towards innovative methodologies and novel research perspectives is vital for advancing our knowledge.
Emerging Technologies
Recent technological advancements are redefining how we study glial cells.
- Genetic Engineering: Techniques such as CRISPR-Cas9 enable precise manipulation of glial cell genes. This can elucidate gene functions and their connections to brain diseases.
- Imaging Techniques: Advanced imaging methods like two-photon microscopy allow researchers to observe glial cell dynamics in real time. This enhances our understanding of cellular interactions in live tissues.
- Multi-Omics Approaches: Integrating genomics, transcriptomics, and proteomics facilitates a comprehensive understanding of glial cells. These methods can uncover cellular responses under different conditions.
Innovative technologies not only enhance our knowledge but also pave the way for targeted therapies.
These emerging technologies help bridge gaps in our understanding of glial cells and highlight their roles in maintaining neural function.
Translational Research Prospects
Translational research aims to convert laboratory discoveries into clinical applications. Glial cells have a significant presence in this area for several reasons:
- Drug Development: Understanding glial cell functions can lead to the identification of potential drug targets. This is particularly essential in neurodegenerative diseases where glial cells play a protective or detrimental role.
- Regenerative Strategies: Exploring ways to harness glial cells for promoting repair mechanisms after injury could lead to new treatments for conditions like spinal cord injuries.
- Personalized Medicine: Tailoring interventions based on individual glial cell profiles could improve treatment efficacy and minimize side effects.
Finale
In this article, we have explored the intricate and vital roles of glial cells in the nervous system. Their functions extend far beyond supporting neurons; they are active participants in various neural processes essential for maintaining overall brain health and functionality.
Summary of Findings
Throughout the article, we examined different types of glial cells such as astrocytes, oligodendrocytes, microglia, and ependymal cells. Each type has unique features and performs specific functions that contribute to the complexity of neural operation. Key findings include:
- Support for Neurons: Glial cells provide structural support and nourishment.
- Homeostasis: They help maintain ion balance and nutrient supply within the brain.
- Neural Communication: By participating in chemical and electrical signaling, they facilitate effective synaptic function.
- Protection and Repair: Glial cells play critical roles in protecting neurons from injury and assisting in their repair when needed.
These functions illustrate that glial cells are vital in maintaining the delicate balance necessary for effective brain operation.
Significance of Glial Cell Research
Research into glial cells is of immense significance for several reasons:
- Understanding Diseases: Studying these cells could provide insights into neurodegenerative diseases such as Alzheimer's and Parkinson's. Understanding the mechanisms of microglial activation can help target therapeutic strategies.
- Innovations in Therapy: The potential for glial cells in regenerative medicine indicates possible advancements in treating injuries and diseases associated with the nervous system.
- Influencing Neurodevelopment: Insights into the role of glial cells during different life stages can enhance knowledge of neurodevelopmental disorders and their implications.
In summary, glial cell research holds promise for unlocking new avenues in neurobiology. Increased understanding can lead to improved diagnosis, treatment, and management of various neurological conditions, clearly emphasizing the need for continued exploration in this crucial area of neuroscience.
"The exploration of glial cells opens new frontiers in understanding brain functions and therapeutic potential in neurobiology."
This narrative underlines that glial cells are not mere background players in the nervous system. Instead, they are critical components whose functions are pivotal to the rich tapestry of brain health.