Comprehensive Analysis of Energy Storage Systems
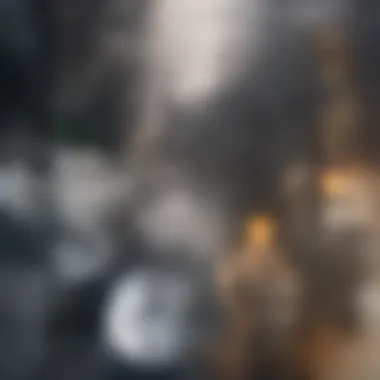
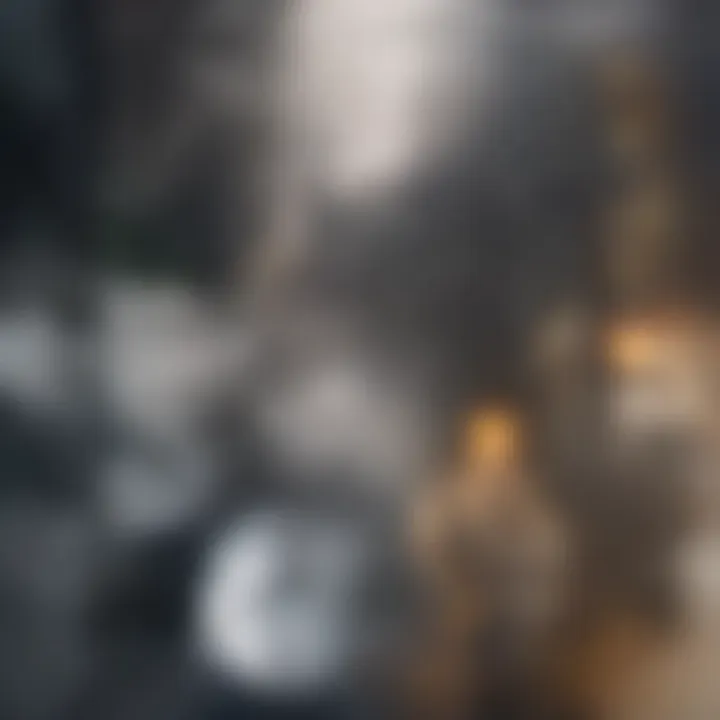
Intro
Energy storage systems play a crucial role in the transition to cleaner and more sustainable energy sources. With the growing reliance on renewable energy, understanding how these systems operate becomes increasingly important. The ability to store energy efficiently can help balance supply and demand, enhance grid stability, and facilitate a smoother integration of renewable energy into the existing infrastructure. This article will explore various types of energy storage systems, their applications, mechanics, and future developments in the field.
The ongoing advancements in technology signal a significant shift in how energy is managed. This analysis will provide a comprehensive overview for students, researchers, educators, and professionals in the field, ensuring they are well-informed about the relevant technologies and their implications for energy sustainability.
Key Findings
Major Results
A variety of energy storage technologies exist today, each with unique mechanisms and performance characteristics. A few noteworthy types include:
- Mechanical Storage: This includes pumped hydroelectric storage and flywheels. These systems store energy by utilizing mechanical principles, often involving gravity.
- Thermal Storage: This method stores energy in the form of heat. Systems can utilize materials like water, salt, or other substances that can absorb and store thermal energy.
- Electrochemical Storage: Lithium-ion batteries dominate this area. They are widely used in both portable electronics and electric vehicles due to their efficiency and scalability.
Emerging technologies such as solid-state batteries, which promise higher energy densities and safety, are also on the horizon. These innovations show promise in enhancing the performance of energy storage systems further.
Discussion of Findings
The findings indicate that energy storage systems are essential for improving the reliability of energy supply, especially as reliance on intermittent renewable sources, like wind and solar, increases. As we transition to greener energy sources, understanding the advantages and limitations of each storage type is critical. For instance, while pumped hydro is efficient and scalable, its geographical constraints may limit its usage. Conversely, batteries offer mobility and versatility but often come with concerns regarding material sourcing and recycling.
Methodology
Research Design
This analysis employs a comprehensive literature review approach. Sources included research articles, case studies, and reports from recognized institutions in the energy sector. By synthesizing various perspectives, the scope of the analysis aims to provide a balanced understanding of the current landscape in energy storage systems.
Data Collection Methods
Data was gathered from multiple reputable sources including academic journals, government reports, and industry publications. This approach ensured a broad range of insights into the functioning and efficacy of different energy storage technologies. Furthermore, the discussion incorporates recent advancements and trends, allowing for an informed perspective on future developments in the field.
"Energy storage is the linchpin for a sustainable energy future." - International Energy Agency
The analysis will explore how the integration of these storage systems can help reduce the overall carbon footprint and improve energy efficiency in various applications. Understanding how to effectively implement these technologies can guide better energy management strategies, which are vital for addressing the challenges posed by climate change.
Intro to Energy Storage Systems
Energy storage systems play a vital role in modern energy management. They serve as a bridge that connects energy generation with consumption, allowing for improved efficiency and reliability. At its core, energy storage involves capturing energy produced at one time for use at a later stage. This capability becomes increasingly essential in the context of renewable energy sources like solar and wind, which are inherently intermittent.
The global shift towards sustainable energy demands innovative solutions, and energy storage systems present numerous advantages. They support the integration of diverse energy resources, helping to stabilize grids and reduce reliance on fossil fuels. This aids in mitigating climate change and promotes environmental sustainability. Moreover, energy storage systems can enhance energy security by providing backup power during outages or peak demand periods.
Numerous technologies exist within the realm of energy storage. Each technology has unique characteristics, advantages, and limitations, which can significantly influence their applications. For instance, mechanical systems often excel in providing rapid responses during peak loads, while electrochemical systems, like lithium-ion batteries, are prevalent in mobile applications due to their compact nature.
As the future unfolds, the significance of these systems will likely increase. Understanding their mechanisms, costs, and environmental impacts is crucial for stakeholders. This includes energy producers, consumers, and policymakers who aim to meet energy needs sustainably.
"Energy storage is more than just a temporary solution; it is integral to a resilient energy future."
In this article, we will explore the variety of energy storage technologies, their comparative efficiencies, and emerging trends that are shaping the landscape. With a focus on performance, cost, and the environmental implications, we aim to provide a comprehensive understanding of the role energy storage systems play in transforming the energy sector.
The Importance of Energy Storage
Energy storage systems play a critical role in the advancement of sustainable energy practices. Their importance is underscored by the growing need for efficient energy management solutions amid rising energy demands and the integration of renewable sources such as solar and wind. These systems not only facilitate the management of energy supply but also optimize the use of generated renewable energy.
One of the key benefits of energy storage is its ability to bridge the gap between energy generation and consumption. In many cases, demand does not align perfectly with energy availability. Energy storage technologies allow excess power generated during peak production times to be stored for later use. This improves the overall efficiency of renewable energy sources.
Moreover, energy storage contributes to a more resilient grid. It acts as a buffer during peak load periods, which can help to reduce the strain on the electrical grid. This is particularly significant as the global shift to renewable energy sources increases grid complexity. The ability to store energy enhances grid reliability and helps mitigate risks associated with outages or supply imbalances, thus improving overall performance.
In addition to this, energy storage systems are central to dynamic energy management and demand response initiatives. They allow for real-time energy supply adjustments, creating opportunities for cost-saving and enhancing operational flexibility.
Simplifying the understanding of these systems, we can point out some of the elements that emphasize their importance:
- Support for renewable energy integration: They enable a stable and continuous supply from variable energy sources.
- Grid stability: They mitigate fluctuations in energy supply and demand, ensuring smoother operations.
- Demand response capability: They enhance responsiveness to peak load demands, allowing for efficient energy distribution.
"Energy storage systems are critical in transitioning to a sustainable energy future, allowing for more reliable and efficient energy supply management."
Overall, understanding the importance of energy storage is crucial for academics, researchers, and industry professionals alike. It becomes evident that these systems are not just supplementary technologies; they are essential for successfully navigating the challenges of modern energy systems.
Overview of Energy Storage Technologies
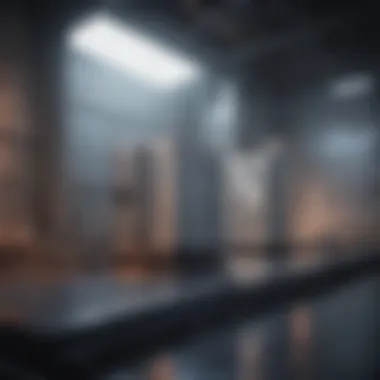
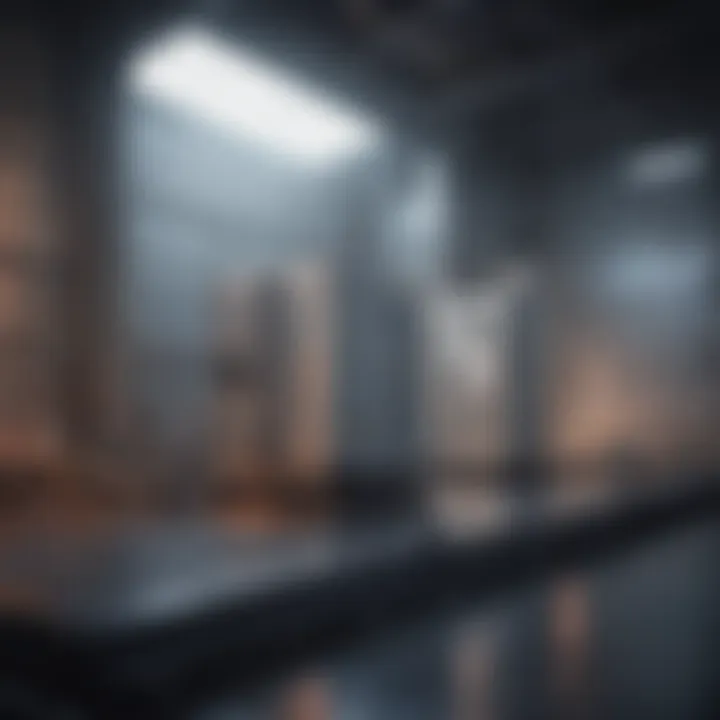
Energy storage technologies are critical components in the modern energy landscape. They enable the integration of renewable energy sources, enhance grid reliability, and help manage energy demand more effectively. Understanding these technologies is vital for professionals and researchers aiming to optimize energy systems for efficiency and sustainability.
Energy storage systems can be broadly categorized into various types, each with unique characteristics and applications. These technologies serve multiple purposes, from balancing supply and demand in power grids to storing excess energy from renewable sources like wind and solar. The significance of energy storage systems lies in their ability to provide flexibility and stability to the energy market, which is increasingly relying on intermittent energy sources.
Mechanical Energy Storage
Flywheel Systems
Flywheel systems are a type of mechanical energy storage that utilizes kinetic energy. They store energy by spinning a flywheel at high speeds. The primary advantage is their ability to deliver energy very quickly when needed, making them suitable for applications requiring immediate power. Flywheels have a long lifecycle and require minimal maintenance, which contributes to their popularity.
However, flywheels are predominantly used for short-term energy needs and might not be suitable for long-duration energy storage. Their efficiency can also be affected by factors like friction and air resistance. Despite these drawbacks, they represent a valuable option in the energy storage landscape, especially for applications like frequency regulation in energy grids.
Pumped Hydro Storage
Pumped hydro storage involves two water reservoirs located at varying elevations. During low energy demand, excess electricity is used to pump water from the lower reservoir to the upper one. When energy is needed, water is released back down to generate electricity. This technology is favored for its ability to store large amounts of energy over long periods.
The key characteristic of pumped hydro storage is its high efficiency and capacity, offering a reliable solution for large-scale energy storage needs. However, it requires specific geographical conditions and significant initial investment. Environmental concerns also arise due to the alterations of landscapes to create reservoirs. Nonetheless, pumped hydro storage remains one of the most widely used methods for energy storage globally.
Thermal Energy Storage
Molten Salt Storage
Molten salt storage systems are designed to store thermal energy using salts that solidify at lower temperatures and become fluid when heated. They are primarily used in conjunction with solar power plants, allowing for the storage of excess thermal energy generated during daylight hours for later use. The significant upside of molten salt storage is its capacity for long-duration storage, thus supporting energy availability even during cloudy periods.
However, the technology requires careful management as the salts can be corrosive and pose challenges in terms of material durability. Despite these limitations, molten salt systems are an effective solution for enhancing the reliability of renewable energy, particularly in solar thermal applications.
Phase Change Materials
Phase change materials (PCMs) store and release thermal energy during the phase transitions between solid and liquid states. This property enables them to maintain temperature levels effectively. PCMs can be integrated into various applications, from building design to industrial processes.
Their primary benefit is their ability to provide a compact and efficient means of energy storage without the need for heavy machinery or large systems. However, their capacity is limited compared to other thermal storage options, which may restrict their application in large-scale scenarios. Still, phase change materials represent an innovative approach in thermal energy management and storage.
Electrochemical Energy Storage
Lithium-Ion Batteries
Lithium-ion batteries are among the most widely used electrochemical energy storage solutions today. They are favored for their high energy density and ability to recharge quickly, making them suitable for portable devices, electric vehicles, and stationary energy storage systems. Their lightweight, compact design is a key advantage, significantly contributing to their popularity and increasing adoption.
However, lithium-ion batteries also present challenges, such as the volatility of materials and environmental concerns related to mining and disposal. Improving their life span and sustainability remains an area of active research.
Flow Batteries
Flow batteries store energy in liquid electrolytes contained in external tanks. This configuration enables scalable energy storage, addressing the limitations of conventional batteries. The flexibility in designing flow battery systems allows them to be tailored to specific energy requirements, making them suitable for various applications.
Yet, flow batteries typically have lower energy density compared to lithium-ion technologies, which can limit their attractiveness in some scenarios. Their relative complexity and higher initial cost can also be significant factors in their adoption. Nonetheless, flow batteries represent a promising path for large-scale and long-duration energy storage needs.
Lead-Acid Batteries
Lead-acid batteries are one of the oldest forms of electrochemical energy storage. They are cost-effective and widely used for backup power systems, automotive applications, and off-grid solar solutions. Their established technology and availability make them a practical choice in various scenarios.
However, their lower energy density and shorter lifespan compared to newer technologies like lithium-ion batteries are notable drawbacks. Environmental concerns arise from lead toxicity and recycling requirements. Despite these issues, lead-acid batteries remain relevant, particularly where cost and reliability are critical factors.
Capacitive Energy Storage
Supercapacitors
Supercapacitors are energy storage devices that combine features of traditional capacitors and batteries. They excel in delivering high power density and rapid charge/discharge cycles, making them ideal for applications requiring quick bursts of energy, such as in power electronics.
A key characteristic of supercapacitors is their long cycle life, which can extend to hundreds of thousands of cycles, significantly outperforming conventional batteries in this aspect. However, their energy density is lower, meaning they hold less energy for a given volume, which can limit their use in long-duration storage applications. Despite this, supercapacitors play a vital role in energy storage systems, especially in conjunction with other technologies to balance energy supply and demand.
Chemical Energy Storage
Hydrogen Storage
Hydrogen storage involves various methods of storing hydrogen gas for use in energy production. Hydrogen can be used in fuel cells to generate electricity or in combustion processes, making it a versatile energy carrier. Its abundance and potential to be produced from renewable sources present significant advantages.
However, challenges exist in hydrogen storage technology, including the complexities of safe storage and transportation, and the energy required for conversion and compression. Despite these obstacles, hydrogen storage holds promise in achieving a sustainable energy future and decarbonizing various sectors.
Biochemical Systems
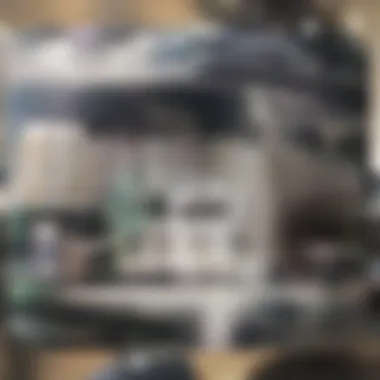
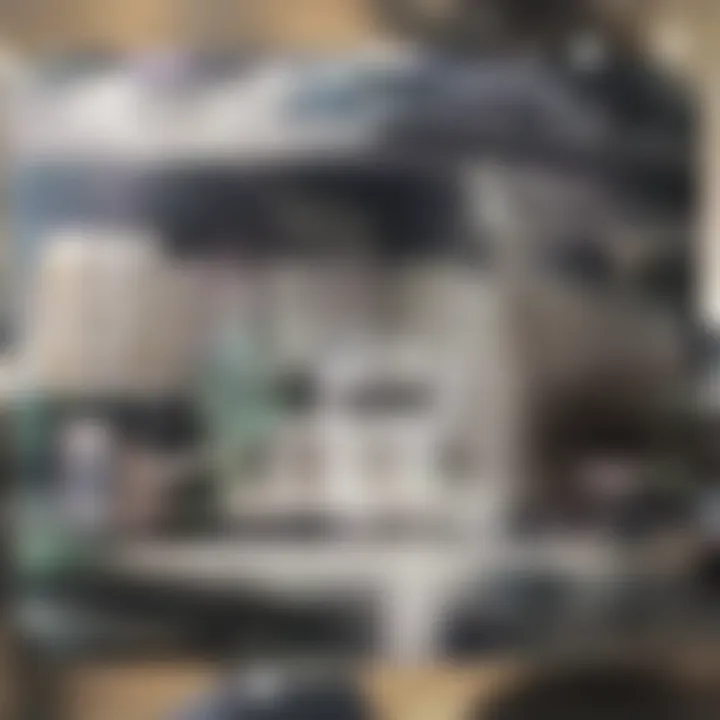
Biochemical systems leverage organic materials to store and convert energy through processes like anaerobic digestion or fermentation. This method can produce biogas or biofuels that serve as alternatives to fossil fuels. The primary benefit of biochemical systems is their integration with waste management, turning organic waste into valuable energy sources.
Nevertheless, these systems are often less efficient compared to conventional energy storage technologies. Furthermore, scalability can be an issue, dependent on the availability of sustainable feedstock. Despite these limitations, biochemical systems present an innovative option for reducing waste and enhancing energy sustainability.
Comparative Analysis of Energy Storage Systems
The comparative analysis of energy storage systems is pivotal in understanding the landscape of energy technologies. This section dissects various storage methods, such as mechanical, thermal, and electrochemical systems. By examining these differences, we unveil essential characteristics, operational efficiencies, and limitations. Understanding these comparisons allows stakeholders to make informed decisions, driven by specific energy needs and sustainability goals.
Efficiency and Performance
Efficiency refers to the energy loss during storage and retrieval. In energy storage systems, the performance metrics vary significantly. For instance, lithium-ion batteries possess about 90% efficiency, making them highly effective for various applications. In contrast, pumped hydro storage often boasts more significant capacities but can have lower efficiencies due to energy loss in water motion and friction. Mechanical systems, like flywheels, usually demonstrate rapid energy discharge but may not store energy for extended periods.
Performance also encompasses response time. Electrochemical systems typically respond more quickly than mechanical counterparts. It becomes crucial when energy demand peaks or renewables generate excess power. Therefore, comparing efficiency and performance enables better alignment with project goals, whether focusing on quick discharge or long-term energy retention.
Cost Considerations
Cost remains a fundamental factor in any energy project. The analysis should include initial capital costs, operational expenses, and maintenance fees. Lithium-ion batteries, while efficient, can have high upfront costs. However, their decreasing price trajectory is noteworthy. Conversely, pumped hydro storage demands high initial infrastructure investment yet offers low ongoing operating costs. This long-term perspective is essential when evaluating the cheapest options over a system's lifespan.
Additionally, economies of scale influence costs. Larger installations may achieve lower per-unit costs, making them more attractive for utility-scale applications. As technologies evolve, the cost-benefit balance may shift, emphasizing the significance of continual assessment in energy storage investments.
Environmental Impact
The environmental implications of energy storage technologies are increasingly essential as sustainability takes center stage. Different systems exhibit varied environmental footprints. For example, lead-acid batteries contain toxic materials, presenting disposal and recycling challenges. In contrast, the lifecycle of lithium-ion batteries typically carries better sustainability, although resource extraction can still pose ecological risks.
Pumped hydro storage, while environmentally disruptive during construction, often provides efficient, large-scale solutions ideal for renewable energy integration. Other systems, like hydrogen storage, show promise for cleaner alternatives, though production methods need further optimization for reduced emissions. Analyzing environmental impacts gives insights into how energy storage systems contribute to overall sustainability goals and informs decision-making for future developments.
Emerging Technologies in Energy Storage
Emerging technologies in energy storage are critical for advancing the efficiency and implementation of sustainable energy solutions. These innovations not only expand the capacity and versatility of existing systems but also bring new materials and methods to the forefront. As we shift towards a more renewable-based energy system, understanding these technologies becomes essential for enhancing energy reliability and performance.
Advanced Battery Technologies
Sodium-Ion Batteries
Sodium-ion batteries present a promising alternative to traditional lithium-ion systems, especially due to the abundance and low cost of sodium. One key characteristic of sodium-ion batteries is their capacity for high energy density, comparable to that of lithium-ion batteries. This makes them suitable for a range of applications, from grid storage to electric vehicles.
A unique feature of sodium-ion technology is its ability to operate effectively at various temperatures, which is essential for varying climates. In addition, sodium-ion batteries have demonstrated robust cycle stability, leading to a longer life cycle when compared to some lithium solutions. Vital advantages include their non-toxicity and reduced dependence on rare materials, which aligns well with the global push for more sustainable technology. However, drawbacks do exist, such as lower efficiency in energy conversion and charging times that tend to be longer than lithium counterparts.
Solid-State Batteries
Solid-state batteries mark a significant advancement in battery technology by utilizing a solid electrolyte instead of a liquid one. This key characteristic enhances safety, as it reduces the risk of leakage and flammability associated with liquid electrolytes. Solid-state batteries are notably more energy dense, allowing for greater energy storage in a smaller footprint, making them advantageous for electric vehicles and portable devices.
One unique aspect of solid-state batteries is their potential for fast charging capabilities, which can revolutionize how energy is consumed. This compressed charging time means users can recharge quickly, improving convenience and efficiency. Despite these benefits, challenges remain. The production costs are currently high, and issues regarding scalability need to be addressed before solid-state batteries can fully penetrate the market.
Innovations in Thermal Storage
Thermal storage focuses on capturing heat energy for later use, which plays an essential role in balancing energy supply and demand. Innovations in this field include advanced materials capable of storing more energy across various temperature ranges. Developments such as thermochemical storage can store heat effectively for long durations, enhancing the practicality and utility of thermal energy systems within large-scale applications like concentrated solar power.
Next-Generation Capacitors
Next-generation capacitors are becoming increasingly significant in energy storage, especially for applications requiring rapid energy discharge. With attributes such as improved power density and charging speeds, these capacitors can facilitate faster response times in various electronic devices. Innovations in materials, such as graphene and different polymer composites, have taken capacitors to a new level, allowing them to achieve higher efficiencies and longer life spans. Despite being very promising, the cost and integration with existing technologies remain crucial considerations as this sector evolves.
Case Studies of Energy Storage Implementation
The examination of case studies in energy storage implementation is crucial for understanding the practical applications and benefits of energy storage systems. Such studies provide tangible examples of how different technologies are employed in real-world scenarios. Analyzing these cases illuminates the challenges and successes associated with diverse energy storage solutions. By evaluating these implementations, stakeholders can draw insights that inform future projects, streamline processes, and potentially lead to innovative solutions.
Utility-Scale Energy Storage Projects
Utility-scale energy storage projects play a significant role in balancing supply and demand across the electrical grid. These large installations can absorb excess energy during peak production times and release it when demand surges. One notable example is the Hornsdale Power Reserve in South Australia, which utilizes Tesla's lithium-ion batteries to provide grid stability and frequency control. Since its operational launch, this facility has successfully demonstrated how large-scale battery storage can reduce reliance on fossil fuels.
Another significant case is the Lake Powell Pumped Storage Plant in Utah. This project employs a pumped hydro storage system, allowing it to store surplus energy by moving water to a higher elevation during low demand periods and releasing it for electricity generation during peak times. Such utility-scale applications showcase how energy storage can enhance grid functionality and reliability.
Commercial and Industrial Applications
In commercial and industrial sectors, energy storage systems contribute to cost savings and efficiency improvements. Many businesses have started to recognize that energy storage can mitigate electrical demand charges, optimize energy use, and provide backup power during outages. For instance, companies like Amazon have deployed battery systems in their warehouses to ensure operational continuity. This approach enables them to minimize disruptions and manage energy costs effectively.
A notable case is that of a large supermarket chain in California, which installed a solar-plus-storage system. This combined approach allowed the retailer to use stored solar energy during peak pricing periods, reducing energy costs significantly. Such implementations are crucial as they not only advocate for sustainable practices but also illustrate the financial benefits of energy storage systems in commercial businesses.
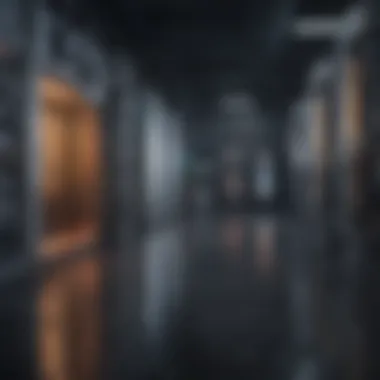
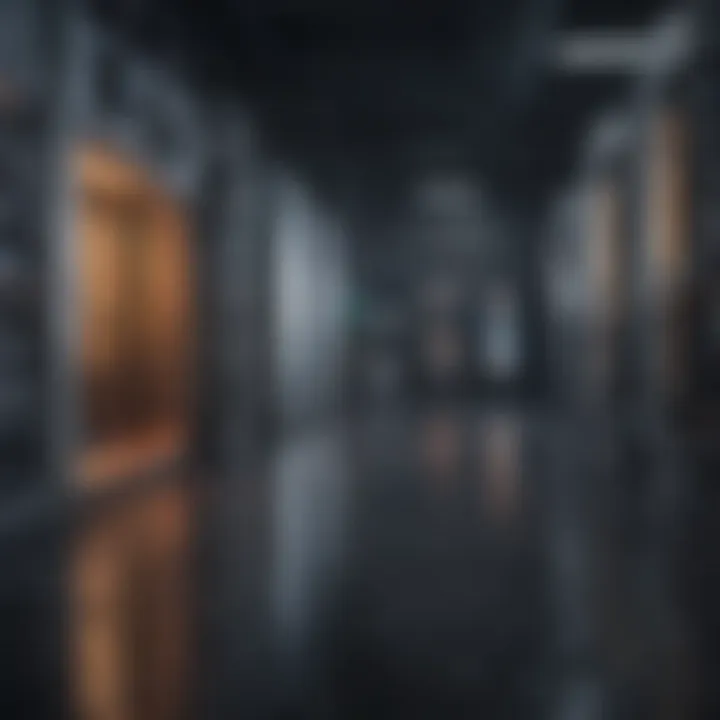
Residential Energy Storage Solutions
Residential energy storage solutions are gaining traction, aligning with the global trend toward sustainable living. Homeowners are increasingly investing in systems like the Tesla Powerwall or LG Chem batteries, which allow them to store energy generated from solar panels. This integration enables them to utilize stored energy during the evening or when utility rates are at their peak.
For example, a project in Germany demonstrated how residential energy storage can enhance energy independence. Homeowners with solar panels were able to achieve over 90% of their energy needs from their storage systems, significantly decreasing their reliance on conventional power sources. This not only decreases energy bills but also contributes to a reduction in carbon footprints. Such case studies highlight the importance of residential systems in driving the shift toward a decentralized, sustainable energy landscape.
These case studies exemplify the capacity of energy storage technology to transform energy consumption patterns, enhance reliability, and contribute to global sustainability goals.
As energy storage continues to evolve and gain popularity, the analysis of these implementation cases provides essential insights for future advancements and encourages broader adoption across multiple sectors.
Regulatory and Policy Context
The regulatory and policy framework surrounding energy storage systems is critical in shaping the future of energy management. This context not only affects how technologies are developed and deployed but also influences investment decisions, research directions, and overall market dynamics. A favorable regulatory environment can spur innovation, while a lack of coherent policy may hinder progress in harnessing the potential of energy storage.
Government Incentives and Support
Government incentives play a pivotal role in energizing the energy storage market. Financial supports such as grants, tax credits, and subsidies can make energy storage solutions more accessible. These measures often aim to reduce initial investment costs for consumers and businesses alike.
Benefits of government incentives include:
- Lowering Entry Barriers: By alleviating financial burdens, incentives stimulate interest and adoption among various sectors.
- Stimulating Technological Advancements: As policies evolve, they encourage research and development in new storage technologies, optimizing efficiency and cost-effectiveness.
- Promoting Renewable Integration: Incentives can effectively support the deployment of energy storage systems alongside renewable energy sources, fostering a cleaner energy future.
Several countries have launched initiatives to incentivize the use of energy storage. For instance, in the United States, the Federal Investment Tax Credit provides tax benefits for the installation of battery storage systems when coupled with solar energy. Other nations like Germany offer feed-in tariffs, enhancing the economic viability of energy storage projects.
International Standards and Regulations
International standards and regulations govern the operation and compatibility of energy storage systems across borders. These frameworks are essential in ensuring safety, reliability, and interoperability of storage technologies. Applicable standards allow for seamless integration into existing power grids, promoting cross-border trade and utility cooperation.
Key considerations regarding international regulations include:
- Safety Protocols: Establishing rigorous safety standards is imperative to minimize risks associated with the storage of energy.
- Performance Metrics: Regulations often delineate performance criteria which energy storage systems must meet to qualify for incentives or integration into grid systems.
- Harmonization of Standards: As storage technologies evolve rapidly, international cooperation can lead to compatible regulations that facilitate faster deployment and integration of innovative solutions.
In summary, a strong regulatory and policy framework, composed of government incentives along with international standards, is crucial for effectively advancing energy storage systems. This relationship not only fosters innovation but also shapes a robust market that can adapt to changing energy demands.
Future Trends in Energy Storage
The evolution of energy storage systems anticipates developments that could significantly alter the landscape of energy management. Understanding future trends is crucial as they highlight where technology is heading, how systems can improve, and the effects these changes may have on energy sustainability.
Many factors contribute to these trends, especially the increasing need for efficiency. Optimizing the performance of energy storage systems helps integrate renewable sources more effectively. This is especially pertinent as countries shift toward cleaner energy dominance. Advanced technologies promise better efficiency and lower costs, which would make energy storage more accessible. Investments are steering research towards innovative materials and formulations, which can lead to cost-effective solutions.
Predicted Market Growth
Predictions suggest that the global market for energy storage will experience exponential growth in the coming years. This is partially fueled by the escalating demand for renewable energy solutions. Market analysts estimate a sizeable increase in investments, reflecting confidence in advanced battery technologies and other storage solutions. The overall trend is expected to align with government policies aimed at reducing carbon emissions, which will drive further expansion in this sector.
Key elements influencing this market growth include:
- Increased utility-scale energy storage projects.
- Rising consumer preference for residential energy storage.
- Supportive regulatory frameworks that encourage adoption.
Integration with Smart Grids
The integration between energy storage systems and smart grids stands as a critical development. Smart grids enhance the efficiency and reliability of electricity distribution, making it easier to manage variable renewable energy sources. Through a combination of digital technology and real-time data, smart grids allow for responsive adjustments based on energy demand and supply fluctuations.
Energy storage plays a vital role within this framework. By serving as buffer storage, these systems can efficiently manage load distribution and help mitigate peak load issues. As more households and businesses adopt solar photovoltaic systems, the importance of energy storage for balancing demand will only grow.
Smart grids also facilitate greater consumer engagement through demand response programs. Users can benefit financially by adjusting their energy consumption according to real-time pricing signals. This proactive approach can lead to more efficient energy usage patterns, ultimately reducing costs and emissions.
"Future trends in energy storage capitalize on advancements in technology and shifts toward sustainable energy solutions, leading the way for more efficient systems."
As energy storage systems continue to evolve and integrate with intelligent grid technologies, the benefits are anticipated to be far-reaching, paving the way for a more resilient and sustainable energy future.
Epilogue
The conclusion serves as a critical reflection on the exploration of energy storage systems throughout this article. It synthesizes the vast information presented, highlighting the implications and future direction of energy storage technologies. As the global energy landscape evolves, understanding the mechanisms and applications of different energy storage systems is vital to align with changing energy demands and sustainability goals.
Summary of Key Findings
In summarizing the key points from the article, we must emphasize the multifaceted role of energy storage. Some significant findings include:
- Diversity of Technologies: Various types of energy storage, including mechanical, thermal, and electrochemical systems, serve distinct purposes and demonstrate unique efficiencies and capabilities.
- Integration with Renewables: Energy storage is crucial for incorporating renewable energy sources like wind and solar. It allows for energy capture when production exceeds demand, thus making renewable energy more viable and widespread.
- Economic Benefits: The deployment of energy storage solutions can lead to significant cost savings in energy management for utilities, businesses, and residential users. Lower energy costs and reduced peak demand are key economic advantages.
- Environmental Significance: Properly utilized energy storage systems contribute to lowering greenhouse gas emissions. This progress aligns with urgent global climate goals, making them a critical component in the transition to sustainable energy.
Call to Action for Research and Development
The findings within this study point towards an important call to action. Research and development efforts must focus on several areas:
- Innovation in Technologies: Continued investment in advanced battery technologies, as well as alternative storage methods, is paramount. Innovations in sodium-ion and solid-state batteries must be accelerated to maximize performance and reduce environmental impact.
- Cost Reductions: Identifying ways to lower the costs of energy storage systems will increase access for a wider range of users. Solutions must be tailored for commercial, industrial, and residential applications to meet diverse needs.
- Policy and Regulation Development: Policymakers should align regulations to support energy storage investments. Incentives, together with robust standards, could stimulate growth in the sector.
- Education and Awareness: Raising awareness about the role of energy storage in modern energy systems can catalyze adoption. Educating businesses and homeowners can lead to broader acceptance and utilization.